Abstract
Amoxicillin (Amx) and cephalexin (Cfx) are β-lactam antibiotics widely used in human and veterinary medicine. Two points of interest surrounding these molecules are the photodegradation of the molecules and their microbiological implications, as well as the persistence and bioaccumulation in the environment which may cause resistance to bacterial strains. The kinetic and mechanistic aspects of the photosensitized degradation of Amx and Cfx have been studied in water at pH 7.4 and 10 by stationary and time-resolved methods. Kinetic evidence indicates that the Rose Bengal-sensitized photooxidation of Amx at pH 7.4 proceeds via O2(1Δg) and mechanisms while at pH 10 the degradation path occurs, principally, via O2(1Δg). For Cfx, this process is attributed to O2(1Δg) and
. Photoproducts, which arise from the addition of oxygen atoms and subsequent oxidation of the groups –CH3 to –COOH, were detected. For both antibiotics the bacteriostatic activity decreases in parallel to their photodegradation. The results of this study could potentially help scientists to better understand and predict the photodegradability of these antibiotics on living organisms and in different environmental compartments.
Introduction
Pharmaceutical drugs, such as antibiotics, belong to the class of biologically active and commercially valuable substances. The degradation of these kinds of compounds is relevant and has received particular attention from two aspects:
1. | Related to the evaluation of the kinetic aspects involved in the photooxidative damage and microbiological implications of the process on the specific therapeutic properties,Citation1,Citation2 and | ||||
2. | Concerned to the degradation of this class of organic pollutants due to the persistence and bioaccumulation of these compounds in the environment.Citation3,Citation4 |
In this work we are specifically interested in the first aspect.
In biological systems, under normal conditions and particularly, in oxidative stress scenarios, different reactive oxygen species (ROS) can be produced. Owing to the fact that the already mentioned species and the different pharmaceutical can be localized in the same microenvironment, there is great relevance to the study of the photodegradation of pharmaceuticals mediated by ROS. In this context, it is interesting to investigate the microbiological implications that arise from the photodegradative process of antibiotics.
For this study, β-lactam antibiotics (Antb) have been selected. These Antb belong to the most widely used in human and veterinary medicine due to high therapeutic efficiency.Citation4–Citation6 This class of antibiotics acts at the last stage in the synthesis of bacterial cell wall by inhibiting the enzymes that catalyze this process.Citation7,Citation8 Specifically, in this paper, amoxicillin (Amx) and cephalexin (Cfx) have been used. Amx is a broad spectrum amino penicillin antibiotic whereas Cfx is a very important alternative in the treatment of infections caused by Staphylococcus aureus.Citation9,Citation10 This bacterium has been chosen for the microbiological assay due to the relevance of this class of microorganisms. They can cause serious multiple infections either community-acquired or nosocomial, including boils, abscesses, endocarditis, osteomyelitis, sepsis and septic shock. They can also infect pacemakers, artificial heart valves, and joint implants.Citation11
The purpose of this work is to investigate, in vitro, the kinetic and mechanistic aspects of the photosensitized degradation process of Amx and Cfx and their possible implications in the antimicrobial activity. To this aim, several specific objectives have been proposed: the study of Amx and Cfx ROS photodegradation-mediated under different pH conditions; a structure-photooxidability comparative kinetic study, a photoproducts analysis and the evaluation of the antimicrobial activity of photodegraded antibiotics.
Materials and methods
Materials
Rose Bengal (RB), Perinaphtenone (PN), Amx, Cfx, sodium azide (NaN3), superoxide dismutase (SOD) were purchased from Sigma-Aldrich (Capital Federal, Argentina) S.A. Deuterated water (99.9%), KH2PO4, Na2HPO4.12H2O, NaOH, and KOH were obtained from Aldrich. Ammonium acetate, methanol, and glycerol high-performance liquid chromatography (HPLC) quality were provided by Sintorgan (Buenos Aires, Argentina). Phenol was purchased from Carlo Erba (Sabadell, Spain).
Bacterial isolates
Two isolates belonging to the genus Staphylococcus, Staphylococcus aureus ATCC 25923 and DM1 were used in the experiments. The ATCC 25923 strain was isolated from animal infection; and the DM1 strain was isolated from human nosocomial infection in Rio Cuarto, Córdoba, Argentina. Staphylococcus aureus ATCC 25923 was selected for these experiments for its resistance to different antibiotics.
The isolates belong to the Microbiology Department bacterial collection of the National University of Rio Cuarto, Cordoba, Argentina. They were maintained at −20°C in 30% glycerol.
Culture media
The culture media used were purchased from Laboratorios Britania S.A. (Buenos Aires, Argentina). These media were Mueller Hinton agar (MHA; meat infusion 300 g; peptone casein acid 17.5 g; starch 15 g; agar 15 g; distilled water 1000 ml) and nutrient broth (NB: meat extract 3 g; soy peptone 5 g; NaCl 8 g; distilled water 1000 ml). Both media were autoclaved at 121°C for 20 minutes.
Methods
Steady-state photolysis
Stationary aerobic photolysis of water solution containing Antb and RB (Abs549 = 0.5, (RB) ≈ 6 × 10−6 M) or PN (Abs365 = 0.5, (PN) ≈ 5 × 10−6 M) was carried out in a home-made photolyser. This is provided with a quartz-halogen lamp (OSRAM XENOPHOT HPLX 64640, 150 w–24 V G35, OSRAM Ausgsburg, Germany) which generates a continuous light that ranges from the central ultraviolet through the visible and into the infrared wavelength regions (ca. 300–2000 nm). A cut-off filter of λ > 320 was used in order to ensure that the light was only absorbed by the photosensitizer. The light was passed through a water filter and focused on the reaction vessel (either a hermetically sealed reaction cell with an oxygen electrode, or a 1 × 1 cm spectrophotometric cuvette) containing the continuously stirred solutions.
The experiments were performed in water at pH 7.4 for both Antb. Particularly, in the case of Amx, due to the presence of a phenolic group, alkaline conditions were also used in order to investigate the effect of the pH.
A specific oxygen electrode Orion 97-08 was employed for oxygen consumption.
From oxygen uptake vs. irradiation time plots, the initial slopes were determined in order to evaluate the relative rates of oxygen consumption. SOD was used in order to investigate whether superoxide anion radical () is involved in the photooxidation process. In this sense, oxygen uptake experiments were done in the absence and in the presence of 1 mg/100 ml SOD.
Reactive rate constants (kr) (process [Citation12], Scheme ) for the reaction of the Antb with O2(1Δg) was determined by a comparative method,Citation12 for which a knowledge of the reactive rate constants for the photooxidation of a reference compound R is required.1 where slopeAntb and slopeR are the respective slopes of their pseudo first order plots of Antb and reference consumption respectively upon sensitized irradiation. The reference compound and Antb were used at identical concentration; oxygen uptake in water was monitored, instead of substrate consumption. Assuming that the reaction of O2(1Δg) with the quencher is the only means of oxygen consumption, the ratio of the first order slopes of oxygen uptake by the reference compound and the substrate, each at the same concentration (slope reference/slope substrate), yields kr/krR. Phenol was used as a reference compound (R), with a krR valueCitation13 of 2.6 × 106 M−1 s−1 (at pH 7.4) and 5.0 × 106 M−1 s−1 (at pH 10).
Scheme 1. Main processes in the photosensitized degradation of the antibiotics (Antb). S, photosensitizer; P, products.
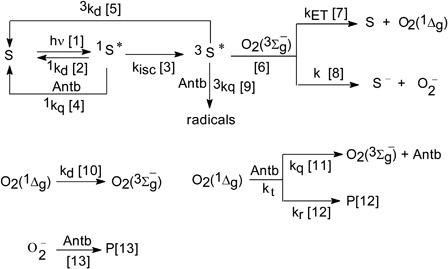
The absorption spectra were measured employing Hewlett Packard 8452A diode array spectrophotometer (Agilent Technologies, Wokingham, UK).
Time-resolved O2(1Δg) phosphorescence detection
The laser-kinetic spectrophotometer for time-resolved O2(1Δg) phosphorescence detection has been previously described.Citation14 In brief, it consisted of a Nd:YAG laser (Brilliant, laser pulses 30 mJ per pulse, ca. 4 ns FWHM) was the excitation source. The irradiance at the surface of the solution was 1.1 × 1013 W/m2. The output at 355 nm was employed to excite the photosensitizer PN.
The emitted radiation (mainly 1270 nm) was detected at right angles using an amplified Judson J16/8Sp germanium detector after passing through appropriate filters. The output of the detector was coupled to a digital oscilloscope and to a personal computer for signal processing. Sixteen shots were used usually averaged in order to get a good signal-to-noise ratio, from which the decay times were determined. The absorbance of the photosensitizer, PN, was 0.2–0.3 at the excitation wavelength. The kinetic decays of the phosphorescent signals were first order in all cases. The experiments were done in D2O, due to the enlargement of the O2(1Δg) lifetime in this solvent.Citation15
The rate constants kt (addition kq + kr, processes [Citation11] and [Citation12], respectively, in Scheme ) were determined from a Stern–Volmer treatment (Eq. 2),2 where τ0 and τ represent the O2(1Δg) phosphorescence lifetime in the absence and in the presence of Antb, respectively.
Laser flash photolysis experiments
Triplet quenching was determined by laser flash photolysis. A Spectron SL400 Nd:YAG laser generating 532 nm laser pulses (20 mJ per pulse, ca. 18 ns FWHM) was the excitation source. The irradiance at the surface of the solution was 1.1 × 1010 W/m2. The laser beam was defocused in order to cover all the path length (10 mm) of the beam being analyzed from a 150-WXe lamp. The experiments were performed with rectangular quartz cells with right angle geometry. The detection system comprises a PTI monochromator coupled to a Hamamatsu R666 PM tube. The signal was acquired by a digitizing scope (Hewlett-Packard 54504, Agilent Technologies, Santa Clara, CA, USA) where it was averaged and then transferred to a computer. All the kinetic determinations were performed at 25 ± 1°C. For the laser photolysis experiments the solutions were de-oxygenated by bubbling during 30 minutes with high purity argon.
Solutions of RB with absorbance ca. 0.2–0.3 at the excitation wavelength were used.
In order to avoid undesired effects such as self-quenching and triplet–triplet annihilation, the disappearance of electronically triplet excited state of RB (3RB*) was measured at low dye concentration (typically 0.02 mM) and at low laser energy. The decays of 3RB* were monitored from the first-order decay of the absorbance at 650 nm, a zone where the interference from other possible species is negligible.
A Stern–Volmer treatment was applied (Eq. 3) in order to determine the rate constants values of process [Citation9], (Citation3kq)3 where 3τ0 and 3τ are the respective lifetimes of 3RB* in the absence and in the presence of Antb.
HPLC-mass analysis
HPLC-mass analysis has been done in order to elucidate the nature of the photoproducts. Steady-state photolysis experiments with air-saturated RB solutions were irradiated with the setup described above. The samples were analyzed with a chromatograph Thermo Scientific Accela, equipped with a quaternary pump, an autosampler and a system spectrometric ion trap (Thermo Scientific LTQ XL) with electrospray ionization interface. A RP-C18 column (150 mm × 2,1 mm × 5 µm) was used. The analysis was carried out at room temperature, using isocratic elution with methanol:water (9:1 v/v) plus ammonium acetate (0.2 mM) as eluent at a flow rate of 0.2 ml per minute, injecting 10 µl. Mass spectra were recorded in both positive and negative ion modes.
Microbiological assay
Staphylococcus aureus ATCC 25923 was used as the control treatment. Both bacterial strains were initially grown in NB medium at 37°C to obtain a cell suspension of 1.5 × 108 cfu ml−1. After incubation, bacterial suspensions were homogeneously spread onto the MHA plates. The bacteriostatic activity of 0.7 mM Amx and 1.0 mM Cfx in solution with RB (Abs549 = 0.5) at pH 7.4 against S. aureus ATCC 25923 and S. aureus DM1 was evaluated employing a modification of Kirby–Bauer methodology,Citation16 in which filter paper disks of 6-mm diameter containing Antb solutions at different irradiation times were placed onto MHA plates surface with the bacterium. The plates were incubated at 37°C for 24 hours and the inhibitory activity was evaluated by measuring the diameter of clear zone around the disks before (H0) and after (H) photolysis. In all cases, the experiments were carried out in triplicate per treatment.
Results and discussion
Reaction scheme
The processes involved in the generation of ROS by visible light irradiation of the photosensitizer S solutions in the presence of Antb are shown in Scheme . Two photosensitizers were employed in the present work: RB which is well established as an important photosensitizer for oxidation reactions and has been used widely in biology and chemistryCitation17–Citation19 and, PN, as an auxiliary photosensitizer in order to evaluate the kinetic process mediated exclusively by O2(1Δg). Both are efficient O2(1Δg) photogenerators with quantum yields for the production of this oxidative species of 0.70 and 1.0 for RBCitation20 and PN,Citation21 respectively, in water. By absorption of a photon, S gives rise to its electronically excited singlet state (process [Citation1]) and, through intersystem crossing, to its electronically excited triplet state (process [Citation3]). The last state can transfer energy and/or an electron to ground state oxygen (O2(3Σg−)) in the aerated solution, generating O2(1Δg) (process [Citation7]) and/or (process [Citation8]). Particularly, for RB a quantum yield in water of 0.2 has been informedCitation17,Citation22,Citation23 for the process [Citation8]. O2(1Δg) and
have been reported as reactive species for oxidation of different substrates.Citation17,Citation18,Citation24
The species O2(1Δg) can decay by collision with solvent molecules (process [Citation10]), and can interact physically (rate constant kq) and/or chemically (rate constant kr) with Antb (processes [Citation11] and [Citation12], respectively), with overall rate constant kt = kq + kr.
Stationary photolysis of Antb upon photosensitization
The visible-light irradiation of air-equilibrated aqueous solution of Amx and Cfx in the presence of RB (Abs549 = 0.5) or PN (Abs365 = 0.5) modifies the absorption spectra of Antb. In parallel, oxygen consumption has been observed.
For Amx, the magnitude of the spectral modifications as well as oxygen uptake was different at the two pHs assayed (7.4 and 10). As observed in , the reactivity in alkaline solutions was much higher than that observed at pH 7.4. This effect is due to the presence of phenolate groups at pH 10, which is more reactive than the non-ionized species, as it was observed for other aromatic compounds with ionisable groups such as –OH or –SH.Citation14
Figure 1. Spectral changes 0.1 mM amoxicillin + Rose Bengal (A549 = 0.5) vs. Rose Bengal (A549 = 0.5) upon visible-light photoirradiation, in aqueous solution at pH 10. Numbers represent irradiation time in min. Inset: Oxygen uptake in the visible-light irradiation by amoxicillin (0.5 mM) upon Rose Bengal-sensitized photooxidation (A) pH 7.4; (B) 10.
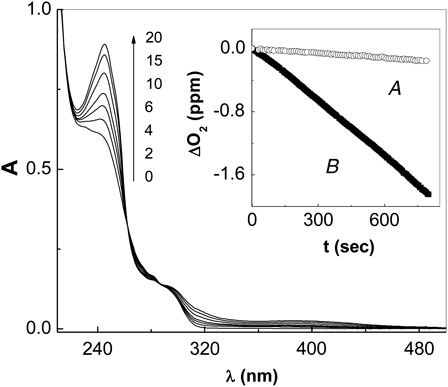
From oxygen uptake vs. irradiation time plots, the initial slopes were determined in order to evaluate the rate of oxygen consumption () upon RB and PN-sensitized irradiation. A comparison of the relative rate oxygen uptake () values employing RB and PN as photosensitizers () clearly indicates that the respective reaction mechanisms, or the photogenerated ROS involved are different from each other.
Interactions photosensitizers-Antb
The quenching of electronically excited singlet state of RB (1RB*) by Antb could not be detected in aqueous solutions. This is most likely because in this solvent the Citation1RB* lifetime is very low, in the order of picoseconds,Citation17 and the excited singlet state cannot be intercepted by Antb within the concentration range employed in this solvent (Antb concentration up to 10 mM). Hence, the process [Citation4] is not significant under the typical experimental conditions employed in this study (ca. Antb 0.5 mM).
The interaction of electronically excited triplet state of RB (3RB*) with Antb (3kq, process [Citation9]) was graphically quantified as shown in ().
Figure 2. Stern–Volmer plots for the time-resolved quenching of electronically triplet excited of Rose Bengal by amoxicillin (A) pH 7.4; (B) pH 10. Inset I: Profiles of oxygen consumption, upon Rose Bengal-sensitized photoirradiation at pH 7.4 (A) Rose Bengal (A549 = 0.5) + 0.5 mM amoxicillin; (B) Rose Bengal (A549 = 0.5) + 0.5 mM Amoxicillin + 1 mg/100 ml SOD. Inset II: Stern Volmer plot for the quenching of O2 (1Δg)-phosphorescence emission by (A) amoxicillin at pH 7.4; (B) cephalexin pH 7.4, and (C) amoxicillin at pH 10.
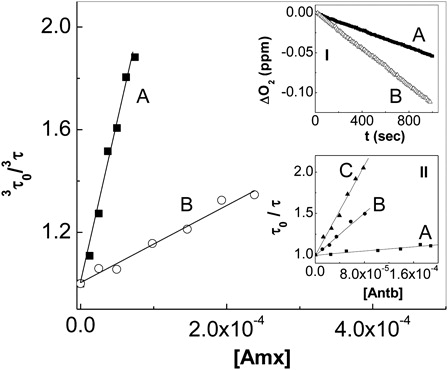
On the other hand, the interaction of Antb with 1PN* can be disregarded. This dye is an aromatic ketone with non-fluorescent character by virtue of a very fast intersystem crossing. As a result, the state 3PN* is populated in the sub-nanosecond time-scale with virtually 100% efficiency.Citation21 Accordingly, only triplet state processes need to be considered.
The amplitude of the signal of O2(1Δg) generation, employing PN as a dye photosensitizer, remained invariant both in the absence and in the presence of Antb in the concentration range employed (Antb concentration up to 0.5 mM). This experimental evidence allows to discard an interaction Antb-3PN*, under this work conditions. Thus, we could claim that an exclusively O2(1Δg)-mediated mechanism operates in the PN-sensitized photodegradation of both Antb.
Results indicate that the sensitization with RB seems to be somewhat different from that with PN, especially on the basis of the interaction Antb-3RB* observed, and due to the different values of found. Consequently, for RB the possibility of a photodegradation mechanism based on the interaction with different ROS was investigated.
Participation of reactive oxygen species in the RB-sensitized photooxidation of Antb
As was previously described in the experimental section, oxygen uptake experiments have been done in the absence and in presence of 1 mg/100 ml SOD, in order to evaluateCitation25–Citation27 the participation of (process [Citation14]).
3
In the case of Amx at pH 7.4, the rate increase of oxygen consumption due to the presence of SOD (, inset I) supports the involvement of the species in the proposed mechanism. It has been reported that SOD can either inhibit or promote the
-mediated oxidation of different substrates.Citation28 H2O2 appears as a product of
dismutation (process [Citation14]), contributing in this way to oxygen consumption.
For Amx at pH 10, the presence of SOD does not produce any effect on the rate of oxygen consumption. This result banishes a significant contribution to an -mediated mechanism.
In the case of Cfx, the presence of SOD increased the rate of oxygen consumption, as compared with the run in the absence of SOD.
On the other hand, for both Antb at the assayed pHs the rate oxygen uptake in the presence of NaN3 diminishes as compared with the one in the absence of this scavenger. This fact shows the participation of O2(1Δg) in the RB-sensitized photodegradation of Amx and Cfx.
Interaction of O2(1Δg) with Antb
As it was already mentioned, the PN-sensitized photodegradation for both Antb proceeds exclusively via O2(1Δg). In this context, the contribution of O2(1Δg) to the photodegradation process of Antb was evaluated using PN as photosensitizer.
The rate constants kt for the overall interaction Antb-O2(1Δg) were determined through a Stern–Volmer treatment (, inset II; ). These experiments unambiguously demonstrate the existence of this interaction, which may be physical in nature (process [Citation11], Scheme ) and/or chemically reactive (process [Citation12]).
The reactive contribution of O2(1Δg), kr (process [Citation12]) to the overall process was evaluated through oxygen uptake measurements as it was mentioned in the experimental section. The kr values were obtained with phenol as a reference compound.
Typical runs of oxygen consumption are shown in the inset of . Moreover, also shows the relative rates of oxygen uptake by Amx and Cfx compared with phenol, upon PN-photosensitized irradiation.
Figure 3. Relative rates of oxygen uptake, upon perinaphtenone-sensitized photoirradiation by (A) phenol; (B) cephalexin and (C) amoxicillin; filled bars pH 7.4; empty bars pH 10. The respective higher rate values of oxygen uptake were arbitrarily normalized to one. Inset: First order plots for the photooxidation perinaphtenone-sensitized at pH 10 (A) phenol; (C) amoxicillin.
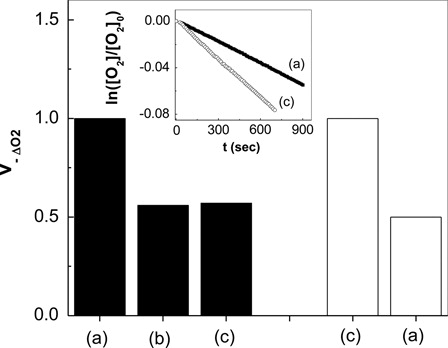
The kr/kt ratio for Antb was included in . This ratio represents the fraction of overall substrate–O2(1Δg) interaction that effectively leads to chemical reaction, as it was previously described.Citation13
In the case of Amx, which has a phenolic group in its structure, the kr and kt values (see ) are highly dependent on the degree of ionization of –OH group. The kr/kt ratio indicates that at pH 10 the chemical degradation is more efficient as compared with the results obtained at pH 7.4. On the basis of acid–base equilibrium of Amx (pKa1 = 2.4, pKa2 = 7.4, and pKa3 = 9.6), at pH 10 more than one half of Amx molecules have the –OH group ionized.Citation29,Citation30 pH dependence has been also observed in aromatic compounds with ionisable groups such as –OH or –SH as it was mentioned above in the text (for a review, see referenceCitation13). In these cases, the acceptedCitation31–Citation33 mechanism of said interaction is the initial formation of an encounter complex substrate–O2(1Δg) with partial charge-transfer character, a formation that is favored by the higher electron-donor ability of the ionized species. The deactivation of this complex will merely lead to physical quenching, whereas complete electron transfer will produce chemical oxidation of the substrate, as shown in Scheme . The balance between physical and chemical quenching is a sensitive function of spin–orbit coupling properties and entropy factors.Citation31–Citation33
In the case of Cfx, taking into account the pKa values (pKa1 = 2.5, pKa2 = 7.3), at pH 7.4, about 50% of the Cfx molecules have the –NH2 group unprotonated.Citation34 Under these conditions a high predominance of the physical O2(1Δg) quenching was observed. For compounds with primary amino groups only physical quenching has been reported.Citation35
Photooxidation products
shows the data of the HPLC–MS analysis of a RB-sensitized irradiated solution of both Antb, at pH 7.4. The samples were photolized between 1 and 5 hours in order to achieve a measurable amount of product.
Table 2. Analysis of the products from the RB-sensitized photooxidation of Amx and Cfx in methanol: water (9:1 v/v) plus ammonium acetate (0.2 mM) by HPLC − isocratic elution by HPLC–MS
In the case of Amx (compound 1), the products arise from the formal addition of oxygen atoms and subsequent oxidation of both groups –CH3 to –COOH (compound 2, which corresponds to assignment: Amx + (2COOH) – (2 CH3) in ). Furthermore, Amx incorporates one –OH group to benzoic ring (compound 3, Amx + (2COOH) – (2 CH3) + (OH)). The analysis of photoproducts of Amx was also done in alkaline conditions and similar results were observed.
Similarly for Cfx (compound 4) the oxidation of group –CH3 to –COOH and the addition of one –OH group to the benzoic ring (Cfx + (2COOH) – (CH3) + (OH)) (compound 5) where observed, while the compound 6 (Cfx + (COOH) – (CH3) + (2OH)) arise from the incorporation of two more –OH groups and compound 7 (Cfx + (COOH) – (CH3) + (3OH)) to the addition of three –OH groups to the aromatic ring.
As shown in , in the mixture of the photoproducts analyzed for both Antb separately, a fraction of them without photooxidation remain present (compound 1 and compound 4) (Scheme ).
Microbiological analysis
The microbiological activity of 0.7 mM Amx or 1.0 mM Cfx visible-light irradiated solutions, in the presence of RB in sensitizing concentrations, was determined at pH 7.4 by evaluation of the inhibition halo before (H0) and after (H) photolysis ().
Figure 4. Relative rates of decrease of the bactericidal activity of (A) amoxicillin and (B) cephalexin at pH = 7.4 upon Rose Bengal-sensitized photoirradiation. H and H0 represent the diameter of the inhibitory halo (the clear zone around the disks for Staphylococcus aureus) in a culture medium of Staphylococcus aureus. Inset: Spectral evolution of cephalexin, upon Rose Bengal-sensitized, as a function of photoirradiation time, in minutes.
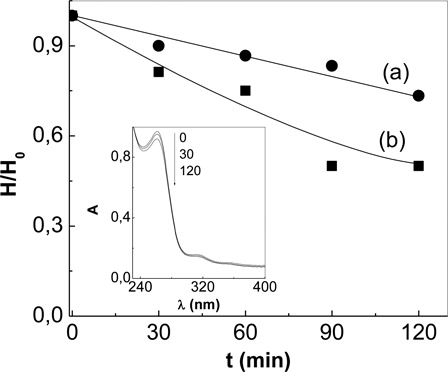
The reference strain S. aureus ATCC 25923 was resistant to both Antb before and after photolysis, which indicates the multiresistance of this strain as was already observed against other Antb.Citation36
shows the relative rates of bactericidal activity of Antb with increasing photolysis time and the inset, the absorbance changes as a function of irradiation time. The results clearly show a decrease of the bactericidal activity of Amx and Cfx for the strain S. aureus DM1 as a consequence of the photosensibilized process. The observed behavior suggests that the photodegradation products have no inhibitory activity on the strain S. aureus DM1, under the experimental conditions studied.
Similar results were obtained in O2(1Δg)-mediated photooxidations with other antibiotics such as sulfa drugs,Citation37 thiazoles1 and tetracyclines2 against microorganisms like S. aureus, Bacillus subtilis, and Pseudomonas aeruginosa.
More recently, photocatalytic decomposition of erythromicin in waterCitation38 has shown a similar behavior. In this case, the degradation intermediates do not have antimicrobial activities against the tested Escherichia coli bacteria.
Conclusions
The antibiotics Amx and Cfx are degraded in aqueous solutions under visible-light irradiation in the presence of photosensitizers such as RB or PN through different mechanisms.
In the presence of PN, both Antb are photooxidized by a O2(1Δg)-mediated mechanism. In the case of Amx, in similitude to other compounds with ionisable –OH groups, this process is more efficient in alkaline medium where the phenol group is ionized. The photodegradation of Cfx has an important contribution from the physical O2(1Δg) quenching.
With RB, the photoxidation of Amx at pH 7.4 proceeds via O2(1Δg) and mechanisms while at pH 10 the degradation path occurs, mainly, via O2(1Δg). For Cfx, the photooxidation process is attributed to O2(1Δg) and
. The bactericidal activity of both Antb decreases in parallel with the photodegradation of the pharmaceuticals.
HPLC/MS analysis of the reaction mixture Antb–RB shows that the products result from the addition of oxygen atoms to Antb and subsequent oxidation of the groups –CH3 to –COOH. Furthermore, –OH groups are added to benzoic ring. The presence of Antb in the analyzed mixture indicates that these substrates are not totally degraded under the conditions used.
The microbiological experiments have shown that the sensitized photo-oxidation produces a clear decrease in the microbiological activity of the Antb.
Acknowledgements
The authors are grateful to Universidad Nacional de Río Cuarto (SECyT, UNRC), Consejo Nacional de Investigaciones Científicas y Técnicas (CONICET) and Agencia Nacional de Promoción Científica y Tecnológica, all from Argentina, for the financial support. The authors also thank Professor N. A. Garcia for his valuable contribution to the interpretation of the results.
References
- Posadaz A, Sanchez E, Guttierrez MI, Calderon M, Bertolotti S, Biasutti MA, et al. Riboflavin and rose Bengal sensitized photoxidation of sulfathiazole and succinylsulfathiazole. Kinetic study and microbiological implications. Dye and Pigments. 2000;45:219–28.
- Castillo C, Criado S, Diaz M, Garcia N. Riboflavin as sensitiser in the photodegradation of tetracyclines. Kinetics, mechanism and microbiological implications. Dyes and Pigments. 2007;72:178–84.
- Trovó AG, Santos Melo SA, Fernandes Pupo Nogueira R. Photodegradation of the pharmaceutical amoxicillin, bezafibrate and paracetamol by photo-Fenton process-Aplication to sewage treatment plant effluent. J Photochem Photobiol A, Chem 2008;198:215–20.
- Elmolla ES, Chaudhuri M. Degradation of amoxicillin, ampicillin and cloxacillin antibiotics in aqueous solution by the UV/ZnO photocatalytic process. J Hazard Mater 2010;173:445–9.
- Rozas O, Contreras D, Mondaca MA, Pérez-Moya M, Mansilla HD. Experimental design of Fenton and photo-Fenton reactions for the treatment of ampicillin solutions. J Hazard Mater 2010;177:1025–30.
- Yeon JJ, Wan GK, Yeojoon Y, Joon-Wun K, Young MH, Hyun WK. Removal of amoxicillin by UV and UV/H2O2 processes. Sci Total Environ 2012;420:160–7.
- Silverstein DC, Hopper K. Small animal critical care medicine chapter 195: penicillins and cephalosporins. Saint Louis, Missouri: Saunders Elsevier; 2009.
- Castle SS. xPharm: The comprehensive pharmacology reference. Penicillin 2007:1–3.
- Marín ZR, Areu RA, Gundian J, Manresa R, Sánchez J, Morales SR. Cefalosporinas. Acta Medica 1998;8:40.
- Jawetz E, Melnick JL, Adelberg EA. Microbiología Médica. 14a Edición. México: Editorial Manual Moderno; 1992.
- Sinha B, Fraunholz M. Staphylococcus aureus host cell invasion and post-invasion events. Int J Med Microbiol 2010;300:170–5.
- Foote CS, Ching TY. Chemistry of singlet oxygen XXI. Kinetics of bilirubin photooxigenation. J Am Chem Soc 1975;97:6209–14.
- García NA. Singlet molecular oxygen mediated photodegradation of aquatic phenolic pollutants. A kinetic and mechanistic overview. J Photoch Photobiol B 1994;22:185–96.
- Miskoski S, Bertolotti S, Arguello G, Garcia NA. On the O2(1Δg)-mediated photooxidative behaviour of tripeptide glycyltyrosyl-alanine in alkaline medium: a kinetic study. Amino Acids 1993;4:101–3.
- Nonell SL, Moncayo F, Trull F, Amat-Guerri F, Lissi E, Soltermann AT, et al. Solvent influence on the kinetics of the photodynamic degradation of Trolox, a water-soluble model compound for vitamin E. J Photoch Photobiol B 1995;29:157–62.
- Bauer AN, Kirby WNM, Sherris JC, Turk M. Antibiotic suceptibility-testing by a standarized single disk method. Am J Clin Pathol 1966;45:493–6.
- Neckers DC. Rose Bengal. J Photoch Photob A 1989;47:1–29.
- Kusama Y, Bernier M, Hearse DJ. Singlet oxygen-induced arrhythmias. Dose- and light-response studies for photoactivation of rose bengal in the rat heart. Circ J 1989;80:1432–48.
- Criado S, Bertolotti SG, García NA. Kinetics aspects of the rose bengal-sensitized photo-oxygenation of tryptophan alkyl esters. Ground state anf photopromoted dye-tryptophan derivative interactions. J Photoch Photobiol B 1996;34:79–86.
- Wilkinson F, Helman W, Ross AB. Rate constants for the decay of the lowest electronically excited singlet state molecular oxygen in solution. An expanded and revised compilation. J Phys Chem Ref Data 1995;24:663–1021.
- Nonell S, Gonzalez M, Trull F. 1H-Phenalen-1-one-2-sulfonic acid: an extremely efficient singlet molecular oxygen sensitizer for aqueous media. Afinidad 1993;448:445–50.
- Srinivasan VS, Podolski D, Westrick NJ, Neckers DC. Photochemical generation of by rose Bengal and Ru (bpy)32+. J Am Chem Soc 1978;100:613–5.
- Lee PCC, Rodgers MAJ. Laser flash photokinetic studies of rose Bengal sensitized photodynamic interactions of nucleotides and DNA. Photoch Photobiol 1987;45:79–86.
- Lambert CR, Kochevar IE. Electron transfer quenching of the Rose Bengal triplet state. Photoch Photobiol 1997;66:15–25.
- Baxter RM, Carey JH. Evidence for photochemical generation of superoxide ion in humic waters. Nature 1983;306:575–6.
- Zang LY, Misra HP. Superoxide radical production during the autoxidation of 1-methyl-4-phenyl-2,3-dihydripyridinium perchlorate. J Biol Chem 1992;267:17547–52.
- Tratnyk PG, Hoigné J. Oxidation of substituted phenols in the environment. A QSAR analysis of rate constants for reaction with singlet oxygen. Environ Sci Technol 1991;25:1596–1604.
- Afanaśev IB. Superoxide ion: chemistry and biological implications. Vol. I. Boca ratón, Fl: CRC Press; 1989.
- Chemie VF. New methods for determination of β-lactam antibiotics by means of diffuse reflectance spectroscopy using polyurethane foam as a sorbent [PhD thesis]. Germany: Duisburg University; 2005.
- Connors KA, Amidon GL, Stella JV. Chemical stability of pharmaceuticals: a handboock for pharmacists part two: stability monograpes. Vol. 1, 2a Ed. England: Wiley, J. and Sons; 1986.
- Gorman AA, Gould IR, Hamblett I, Standen MC. Reversible exciplex formation between singlet oxygen and vitamin E. Solvent and temperature effects. J Am Chem Soc 1984;106:6956–9.
- Gorman AA, Lovering G, Rodgers MAJ. The entropy-controlled reactivity of singlet oxygen (1Δg) toward Furans and Indoles in toluene. A variable-temperature study by pulse radiolysis. J Am Chem Soc 1979;101:3050–4.
- Gorman AA, Gould IR, Hamblett I. Time-resolved study of solvent and temperature dependence of singlet oxygen (1Δg) reactivity toward enol ethers: reactivity parameters typical of rapid reversible exciplex formation. J Am Chem Soc 1982;104:7098–104.
- Mensa J, Gatell JM, Azanza JR, Dominguez-Gil A, García JE, Jiménez de Anta MT, et al. Guía terapéutica antimicrobiana. Capítulo I: Características de los Antimicrobianos. 18a Edición. Barcelona: Editorial Elsevier Masson; 2008.
- Wilkinson F, Brummer JG. Rate constants for the decay and reactions of the lowest electronically excited state of molecular oxygen in solution. J Phys Chem Ref Data 1981;10:809–1000.
- Frola ID. Trabajo final en Microbiología. Perfiles de susceptibilidad a antimicrobianos de cepas de Staphylococcus coagulasa positivos y negativos aisladas de leche de tambos de la región Centro-Sur de la provincia de Córdoba. Dpto. de Microbiología e Inmunología de la UNRC; 2008.
- Díaz M, Luiz M, Bertolotti S, Miskoski S, García NA. Scavening of photogenerated singlet molecular oxygen and superoxide radical anion by sulpha drugs-kinetics and mechanism. Can J Chem 2004;82:1752–9.
- Xekoukoulotakis NP, Xinidis N, Chroni M, Mantzavinos D, Venieri D, Hapeshi E, et al. UV-A/TiO2 photocatalytic decomposition of erytromycin in water: factors affecting mineralization and antibiotic activity. Catalysis Today 2010;151:29–33.