Abstract
Purpose
Quantitative monitoring of the redox status is the foundation for redox-related treatment. The purpose of this study was to evaluate the reliability of a new depolarization curve method for plasma redox potential (ORP) monitoring.
Methods
Using the new method, we performed redox determinations for the first time under different sample-handling conditions, including redox titration experiments using KMnO4 and vitamin C and dynamic redox monitoring in burn patients. The relative ORP value (ΔORP) method (improved traditional method) was used as the reference.
Results
The new method's better reliability, electrochemical specificity and practicability, and known group validity, which are closely associated with the redox-related pathological processes of severe burns, were confirmed. Furthermore, bidirectional change in the redox status in severe burn patients was also observed for the first time.
Conclusions
This simple, stable new method could be a better practical tool for making the dynamic monitoring of the redox status feasible and for providing useful quantitative information for the judgment of redox-related pathological process, thus improving corresponding individualized treatments that rely on quantitative adjustments to the redox status.
Introduction
The stability of the redox status is necessary for normal metabolism.Citation1 The extracellular redox environment is dynamically adjusted by the intracellular metabolism, exerting a profound effect on cell–cell communication and function. Changes in redox status triggered by neutrophil respiratory bursts also have an effect on the mitochondria of other cells.Citation2 Therefore, monitoring the real-time changes in the extracellular redox status would be very helpful in clinical management.Citation3 Owing to the multiplicity and additivity of oxygen radical reactions, it is of significant practical importance to monitor the overall redox status.Citation4
An oxidation–reduction system involves redox reactions that include the transfer of electrons from a reductant to an oxidant. At equilibrium, the redox potential (ORP) can be calculated according to the Nernst equation. The ORP has been shown to be informative as a quantitative tool for monitoring the environmental redox status.Citation5 Specifically, the ORP reflects the comprehensive effects from all of the redox buffer systems, which vary with the total concentrations of the reductants and oxidants in a particular system: the higher the ORP, the more oxidizing the system. Based on the Nernst equation, for every 30-mV change in the ORP, a 10-fold shift in the equilibrium between reductants and oxidants is expected.Citation1 In consideration of the electrochemical characteristics of the ORP and individual differences among the reactants, dynamic monitoring of the ORP should also have a greater clinical application value.
There have been some reports about the applications of the ORP in clinical medicine.Citation6 However, for the traditional ORP-measuring method, a long time must be spent establishing the equilibrium of the potential to determine the ORP due to the limitations of the electrochemical properties of the electrodes and the complicated blood redox reaction system. Furthermore, it is laborious and difficult to maintain the conditioned electrodes to reduce accidental error.Citation7 As a result, actual clinical monitoring has not been conducted.
It has been reported that the stability of ORP determinations could be improved by pretreatment of the platinum working electrode.Citation8 For the depolarization curve method, the polarization process is helpful for reducing interference by removing any oxide film or other adsorbate on the surface of the working electrode, which accelerates the redox reaction speed and shortens the measuring time. This approach has been well applied in the fields of oceanography and soil science.Citation9,Citation10
The purpose of this study was to evaluate whether the depolarization curve method could be used as a simple and reliable determination method for clinical monitoring of the blood redox status (because blood is the critical extracellular environmental medium for normal metabolism). For practical clinical applications, monitoring of the plasma ORP would be preferential because it would not be influenced by blood cells. The traditional method was used as the reference. To reduce the accidental error in the traditional method due to the instability of the electrode, the improved relative ORP (ΔORP) method was employed in this study.
The erythrocytic methemoglobin (Met-Hb) and uric acid (UA) levels were also used to validate the new method. Met-Hb is the oxidized form of hemoglobin in red blood cells and reflects the intracellular redox status.Citation11 UA is the most abundant aqueous antioxidant in humans and contributes as much as two-thirds of all of the free radical-scavenging capacity of plasma,Citation12 closely corresponding to changes in the redox status following ischemia-reperfusion injury.Citation13
Materials and methods
Subjects
In severe burn patients, a characteristic hypovolemic shock frequently occurs within 48–72 hours after injury due to increased capillary permeability and evaporative losses. The ischemia-reperfusion following the shock results in oxidative stress, and the severe sepsis caused by wound or lung infections, accompanied by the activation of polymorphonuclear neutrophils (respiratory bursts), is also associated with oxidative stress.Citation14 In this study, 50 formerly healthy burn patients (40 male and 10 female) ranging from 30 to 45 years old (including 30 severe burn cases with 7 cases of death and 23 survivors burned by hot water and fires, and 20 cases with mild burns) from the Department of Burn Surgery of the Second Affiliated Hospital of Zhejiang University School of Medicine were enrolled (Tables and ). One healthy male (37 years old) also participated in this study. All of the severe burn patients were assessed using the Abbreviated Burn Severity Index and the Sepsis-related Organ Failure Assessment upon admission and had more than 40%TBSA (total body surface area) burns (grade II–III°). The 20 patients with mild burns had no shock symptoms and had less than 10%TBSA burns (grade I–II°). The 23 surviving severe burn patients (18 male; 5 female) had a mean age of 37.5 years ± 5.07 SD. In 15 cases, there was no early operation, and in 8 cases, early escharectomy was performed on the third or fourth day after injury to remove the eschar (approximately 20-30% TBSA). These patients experienced shock symptoms (confusion, loss of consciousness, low urine output, low mean arterial pressure, increased blood lactic acid, insufficient perfusion of the skin, rapid pulse, etc.) without distinct inhalational injuries, gastrointestinal dysfunction and wound infections, but they retained basic normal liver and kidney function. The patients' blood sugar levels were controlled to within normal limits using intravenous insulin therapy. Early enteral nutrition support and antibiotic treatment to prevent infection were also administered. Routine anti-shock fluid therapy was administered after admission, approximately 6 hours after injury, and shock symptoms were alleviated within 3 days following injury. The alterations in the redox status from admission to 7 days after injury were observed. Five of the severe burn patients died of advanced Pseudomonas aeruginosa sepsis (4 male and 1 female, mean age: 40 years ± 5.52 SD), and the changes in the redox status prior to death were also observed. Two other severe burn patients died due to severe malnutrition and multi-organ failure without active treatments. All of the surviving patients with severe burns were discharged after basic cure (residual burn wound: less than 1% TBSA). The ORP was also measured at discharge. All of the clinical diagnoses and treatments were in accordance with Chinese Medical Association criteria.Citation15 This study was conducted according to the Declaration of Helsinki, and it was approved by the hospital ethics committee. Informed written consent was obtained.
Table 1. Demographic and clinical characteristics of the cohort of 30 severe burn patients
Table 2. Demographic and clinical characteristics of the cohort of 20 mild burn patients
Materials and instruments
A PH211 pH/mV/temperature meter, ORP electrode (HI3131B), pH electrode (HI1413B) (Hanna Co., Ltd. Italy) and FJA-4 depolarization redox potential automatic cryoscope (Soil Technical Service Center of Nanjing Institute of Soil Science of Academia Sinica, China) were used in this study. The RAPID Lab 1265 System (Bayer Health Care Co., Ltd. Germany) was also used to measure the intracellular Met-Hb levels, and the AU5400 Chemistry System (Beckman Coulter, Inc. USA) was used to measure the UA levels.
The ORP was measured using the depolarization curve method based on the potential relative to a standard hydrogen electrode, which was equivalent to the value measured using the HI3131B (traditional method) plus the standard electrode potential of the Ag/AgCL electrode (reference electrode of HI3131B).
ORP measurement using the depolarization curve method
Measurements were taken in a closed tube from which the air was removed. The time and voltage of the polarizations and depolarizations were set to be appropriate. For each measurement, triplicate determinations were performed for each sample (the variation of the measured values of three consecutive determinations should have been less than 10 mV); the average of the three values was rounded-off without a decimal point and served as the final ORP value. The electrode was calibrated using the redox reference solution (HI7021L) before each determination.
Femoral venous blood was withdrawn using a BD Vacutainer® containing heparin lithium (BD Vacutainer, New Jersey, USA) at the same time of day, and the patient's body temperature was maintained at 37–38°C. Nutrition support and known antioxidant therapy were not administered for half an hour before blood withdrawal. Similarly, arterial blood gas analysis was performed to confirm that the pH and oxygen partial pressure were within normal limits. Blood samples were centrifuged at room temperature at 2500 RPM for 5 minutes to separate the plasma without hemolysis. Diluted plasma (1 ml plasma + 3 ml NS: normal saline) was used for the analyses to decrease the amount of blood needed.
Relative ORP (ΔORP) method by traditional electrode
As a blank solution, 5 ml Lactated Ringer's solution (LR) was used after its pH was adjusted to be equivalent to plasma using 5% sodium bicarbonate. After the potential value of the blank solution was measured, 1 ml of plasma was added, and the potential value was measured again. The difference between the measurements was termed the ΔORP. Determination was performed similarly using the depolarization curve method with the HI3131B electrode under airtight conditions.
KMnO4/vitamin C redox titration experiments
KMnO4 and vitamin C injections were administered for the in vitro redox titration experiments to estimate the electrochemical specificity of the new method. A standard KMnO4 solution was prepared according to the Analytical Chemistry method (fifth edition, China Higher Education Press). The concentration of the KMnO4 solution was calibrated using the Na2C2O4 method.
Statistical analysis
The data were analyzed using a computerized statistical software package (SPSS version 16.0, SPSS Inc., Chicago, USA), including the calculation of the coefficients of variation (CVs) to assess the stability of the new method, the Friedman test to evaluate the variations in the plasma ORP/ΔORP measurements during the shock stage and at discharge, and the Spearman test to evaluate the correlation between the plasma ORP/ΔORP values and the Met-Hb and UA levels. P values of <0.05 were considered significant.
Results
The stability of the new method
Ten continuous OPR determinations (over 40 minutes) were carried out using plasma samples from one healthy male and 8 mild burn patients under the same conditions. The variations in pH of the test samples before and after each determination were less than 0.1.
For the redox reference solution (HI7021L), plasma samples and diluted plasma samples, all of the CVs of the measured OPR/ΔORP values were lower than 2.7%, even after one day of storage at −80°C (Tables ).
Table 3. Stability of measurements using the depolarization curve method and improved traditional method using blood samples from a healthy person or redox reference solution
Table 4. Stability of measurements of the depolarization curve method using plasma samples from eight mild burn patients
Table 5. Stability of measurements of the depolarization curve method using diluted plasma samples from eight mild burn patients
The ORP of the plasma was slightly lower than the ORP of the blood by approximately 20 mV. The measurements of the ΔORP also showed the same trend.
The electrochemical specificity of the new method
Within the scope of the changes in the ORP of the clinical observations of the severe burn patients (approximately <100 mV) and excluding the influence of pH and the oxygen content, differing results for the redox titration experiments using a diluted plasma sample from one mild burn patient and NS were revealed using low-concentration solutions of KMnO4 and vitamin C (Tables and ).
Table 6. Electrochemical specificity of the depolarization curve method in KMnO4/vitamin C redox titration experiments
Table 7. Electrochemical specificity of the improved traditional method in KMnO4/Vitamin C redox titration experiments
Known group validity of the new method
The close relationship between the ORP (or ΔORP) and the level of oxidative stress during ischemia-reperfusion injury in severe burn patients in the shock stage
Approximately 5 days after injury, the ORP in each patient increased continuously to a peak and then declined gradually (A). The changes in the ΔORP and UA level were inversely correlated with the ORP (P < 0.05), reflecting changes in the antioxidant buffering capacity (B and C). The Met-Hb levels showed a transient rise one day after injury (D). Consistent with the ΔORP, the ORP in each patient at discharge was significantly lower than the peak value during the shock stage (P < 0.05). Furthermore, even for the eight surviving patients who underwent early operation, similar dynamic changes in the redox status were also observed (E and F).
Figure 1. (A) Changes in the ORP in 15 severe burn patients without early Escher-shaving operation during the shock stage. (B) Changes in the ΔORP in 15 severe burn patients without early Escher-shaving operation during the shock stage. (C) Changes in the UA levels in 15 severe burn patients without early Escher-shaving operation during the shock stage. (D) Changes in the methemoglobin (Met-Hb) levels in 23 severe burn patients (with and without early Eschar-shaving operation) during the shock stage. (E) Changes in the ORP in 8 severe burn patients who underwent early Eschar-shaving operations during the shock stage. (F) Changes in the ΔORP in eight severe burn patients who underwent early Eschar-shaving operations during the shock stage. The known group validity according to dynamic monitoring of the ORP, ΔORP, uric acid level and methemoglobin (Met-Hb) content of plasma samples from 23 severe burn patients (with and without early Escher-shaving operation) during the shock stage is shown. For the ORP, diluted plasma samples (1 ml of plasma + 3 ml of normal saline) were used. For the ΔORP, uric acid level and methemoglobin (Met-Hb) content, plasma samples were used. Plasma samples were consecutively collected from 23 severe burn patients for approximately 7 days before and after fluid treatment during the shock stage (mean ± SD). The data are presented according to the time of day: d0 (within six hours after injury, no anti-shock fluid treatment had been administered), d1 (1 day after injury and anti-shock fluid treatment), and d3, d5, d7, and d9 (3, 5, 7, and 9 days after injury and anti-shock fluid treatment, respectively). Discharge represents the data at discharge. The abscissa axis is time (day). The close correlations between the plasma ORP and ΔORP measurements and the UA levels were confirmed (P < 0.05).
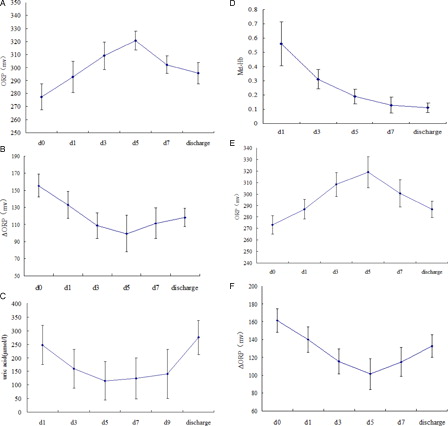
However, for mild burn patients, the ORP and UA levels displayed either only a transient change, similar to the Met-Hb levels, or slight fluctuations during the early stages after injury.
The close relationship between the ORP (or ΔORP) and the level of oxidative stress during sepsis in severe burn patients
The dynamic changes in the plasma ORP and the intracellular Met-Hb levels in five of the severe burn patients in late sepsis who eventually died showed that as the disease progressed, the values increased simultaneously and continuously (A and B), and a significant correlation (P < 0.05) was found between them.
Figure 2. (A) Dynamic changes in the ORP (compared with d0) in five severe burn patients in late sepsis. (B) Dynamic changes in the Met-Hb levels in five severe burn patients in late sepsis. (C) Dynamic changes in the ΔORP in five severe burn patients in late sepsis. (D) Dynamic changes in the uric acid levels in five severe burn patients in late sepsis. The known group validity according to the dynamic monitoring of the ORP, ΔORP, uric acid level and methemoglobin (Met-Hb) content of plasma samples of five severe burn patients in late sepsis prior to death is shown. For the ORP, diluted plasma samples (1 ml of plasma + 3 ml normal saline) were used. For the ΔORP, uric acid level and methemoglobin (Met-Hb) content, plasma samples were used. Plasma samples were consecutively collected from five severe burn patients in late sepsis prior to death. The data are presented by the time of day: day 1 (one day before death), day 3 (the third day before death), and day 5 (the fifth day before death). The abscissa axis is time (day). The change trend along with progress of disease is shown.
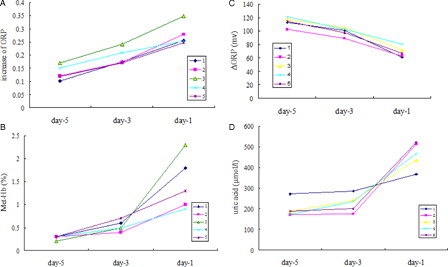
Similarly to the shock stage, the opposite change tendency between the ORP and ΔORP was found (C). But, a rapid increase in the UA level during late sepsis was also observed (D).
For the other two burn patients who eventually died without active treatments, their plasma ORP gradually declined (showing approximately 40 mV of variation between the third day and fifth day before death).
Discussion
In this study, the reliability of the clinical dynamic monitoring of the ORP using a new determination method was evaluated. To adapt it to clinical applications, which were different than the method's original applications, further improvements were made: a fixed time for blood sampling, the usage of anticoagulation tubes containing heparin lithium to reduce the influence of sampling on blood cell components, airtight determinations to reduce the influence of outside substances such as oxygen, and slow swinging movements to simulate the internal milieu and accelerate the electrochemical reactivity. Therefore, stable results could be obtained within 3–10 min.
The fairly low CV values from the continuous determinations of a duration of more than 40 minutes suggested that the new method was more stable compared with the traditional method. For the blood samples, the electrochemical specificity of the new method was also verified through redox titration experiments in vitro. Diluted plasma samples showed a higher redox buffering capacity than NS, which was consistent with their electrochemical characteristics.
The result for the differences between the ORP measurements in plasma and blood was also consistent with a previous report.Citation8
Regarding the known group validity, the plasma ORP values of the 15 surviving severe burn patients who did not undergo early operation increased during the shock stage and then significantly decreased after recovery, reflecting different redox statuses under different pathological conditions. Furthermore, similar dynamic changes were also observed in the eight patients who underwent operative trauma, which suggested that although the etiology during the early stages of severe burns was multi-factorial, ischemia-reperfusion injury was likely to be the major factor causing the abnormal redox status. These results were also in close agreement with previous animal experimentsCitation13 and were consistent with past pathophysiology investigations,Citation14 which together indicated the clinical specificity of the new method and its potential for clinical applications.
Although the functions of the liver and kidneys were near normal, the dynamics of the UA levels were generally opposite the ORP changes, and a transient abnormal increase in the intracellular Met-Hb levels also indicated the existence of oxidative stress during the shock stage. A opposite dynamic change trend in the ΔORP further suggested the accuracy of the ORP determination using the new method during the shock stage.
In fact, the manifestations above did not appear during the early stages for mild burn patients without shock symptoms, but transient changes or slight fluctuations in the ORP and ΔORP and the UA levels were observed, which also reflected the sensitivity of the new method.
Furthermore, the clinical data on the ORP/ΔORP and Met-Hb levels in severe burn patients in late sepsis were also consistent with previous redox studies.Citation16 These data reflected the gradually aggravated, excessive levels of oxidative stress owing to serious infections and inflammatory reactions, suggesting the clinical usefulness of ORP measurements. The excessive accumulation of UA during late sepsis might be due to the gradually worsening renal function.
Of note, the other two severe burn patients who died experienced serious malnutrition and multi-organ failure and showed gradually reduced ORP before death, which might have been associated with their hypothermia and lowered metabolic status.Citation17 Bidirectional change in redox status in severe burn patients further demonstrated the importance of quantitative monitoring of the redox status.
Interestingly, the results of the vitamin C injection titration experiment in vitro using blood samples from one severe burn patient in the shock stage were similar to recent reports on high-dose vitamin C therapy for shock following burn injury.Citation18
As mentioned above, the new depolarization curve method appears to be a reliable, feasible method for actual clinical monitoring, providing a reference for the development of an instrument to determine clinical redox potentials. Of course, its miniaturization is also possible.Citation19
For the ΔORP method, the lack of cumbersome electrode processing reduced the number of accidental errors caused by the imperfections in electrode processing, and taking LR as a blank solution avoided the influence of the traditional redox titrant on the blood components. The ΔORP method also gave a higher value than direct determination using the traditional method, which might make it possible for to standardize the measurement of the ORP. In addition, the ΔORP method would take more time (approximately 10 min compared with the depolarization method).
The significance of the redox status in clinical medicine is not fully understood, and pathophysiological analysis of a larger sample would further clarify the clinical significance of the ORP, providing specific, valuable information for timely clinical management.
Acknowledgements
This work was supported by the Scientific Research Starting Foundation for Returned Overseas Chinese Scholars and the Science Technology Department of Zhejiang Province (Qianjiang project: 2011R10071). The authors thank professor Fang JianAn (Nanjing Institute of Soil Science, Academia Sinica, China) for support and guidance with electrochemical techniques, and professor Han ChunMao (Medical College of Zhejiang University, China) for expertise in burn pathophysiology.
References
- Circu ML, Aw TY. Redox biology of the intestine. Free Radic Res 2011;45(11–12):1245–66.
- Banerjee R. Redox outside the box: linking extracellular redox remodeling with intracellular redox metabolism. J Biol Chem 2012;287(7):4397–402.
- Huang KC, Hsu SP, Yang CC, Ou-Yang P, Lee KT, Morisawa S, et al. Electrolysed-reduced water dialysate improves T-cell damage in end-stage renal disease patients with chronic haemodialysis. Nephrol Dial Transplant 2010;25(8):2730–7.
- Manning P, McNeil CJ. Electrochemical and optical sensing of reactive oxygen species: pathway to an integrated intracellular and extracellular measurement platform. Biochem Soc Trans 2011;39(5):1288–92.
- Aeschbacher M, Sander M, Schwarzenbach RP. Novel electrochemical approach to assess the redox properties of humic substances. Environ Sci Technol 2010;44(1):87–93.
- Rael LT, Bar-Or R, Aumann RM, Slone DS, Mains CW, Bar-Or D. Oxidation–reduction potential and paraoxonase–arylesterase activity in trauma patients. BBRC 2007;361(2):561–5.
- Topcu A, McKinnon I, McSweeney PL. Measurement of the oxidation-reduction potential of cheddar cheese. J Food Sci 2008;73(3):198–203.
- Goldin MM, Blanchard GJ, Evseev AK, Kolesnikov VA, Goldfarb YS, Volkov AG, et al. Redox potential measurement in aqueous solutions and biological media. 212th ECS Meeting; 2007 October 7–12, Washington, DC: 2007. p. 815.
- Luo XX, Xing ZQ. Comparative study on characteristics and influencing factors of soil respiration of reed wetlands in yellow river estuary and liaohe river estuary. Procedia Environmental Sciences 2010;2:888–95.
- Liu ZG, Yu TR. Depolarization of a platinum electrode in soils and its utilization for the measurement of redox potential. Eur J Siol Sci 1984;35(3):469–79.
- Keszler A, Piknova B, Schechter AN, Hogg N. The reaction between nitrite and oxyhemoglobin: a mechanistic study. J Biol Chem 2005;283(15):9615–22.
- So A, Thorens B. Uric acid transport and disease. J Clin Invest 2010;120(6):1791–9.
- Mittal A, Göke F, Flint R, Loveday BP, Thompson N, Delahunt B, et al. The redox status of experimental hemorrhagic shock as measured by cyclic voltammetry. Shock 2010;33(5):460–6.
- Parihar A, Parihar MS, Milner S, Bhat S. Oxidative stress and anti-oxidative mobilization in burn injury. Burns 2008;34(1):6–17.
- The Chinese Medical Association. Clinical diagnosis and treatment guidelines: volume of burn surgery. Peking: People's medical publishing house; 2007.
- Biswal S, Remick DG. Sepsis: redox mechanisms and therapeutic opportunities. Antioxid Redox Signal 2007;9(11):1959–61.
- Kowaltowski AJ. Caloric restriction and redox state: does this diet increase or decrease oxidant production? Redox Rep 2011;16(6):237–41.
- Kahn SA, Beers RJ, Lentz CW. Resuscitation after severe burn injury using high-dose ascorbic acid: a retrospective review. J Burn Care Res 2011;32(1):110–7.
- Lee JH, Seo Y, Lim TS, Bishop PL, Papautsky I. MEMS needle-type sensor array for in situ measurements of dissolved oxygen and redox potential. Environ Sci Technol 2007;41(22):7857–63.