Abstract
Objectives
The subject of this study was the hexahydropyridoindole compound SMe1EC2 with reported antioxidant and neuroprotective effects and low toxicity. In this study, the antioxidant action of SMe1EC2 was investigated in a greater detail in the system of isolated rat erythrocytes.
Methods
First, the compound was subjected to the DPPH test. Second, the overall antioxidant action of the compound was studied in the cellular system of isolated rat erythrocytes oxidatively stressed by free radicals derived from either the hydrophilic azoinitiator AAPH or the lipophilic t-BuOOH, and compared with reference antioxidants.
Results
The DPPH test revealed significant antiradical activity of SMe1EC2 comparable with that of the standard trolox. In the cellular system, SMe1EC2 protected red blood cells against free radical-initiated hemolysis. The overall antioxidant efficacy of SMe1EC2 relative to the reference antioxidant stobadine was strongly affected by the lipophilicity of the initiating free radical species.
Conclusions
The results proved high antiradical efficacy of SMe1EC2. In the system of t-BuOOH/isolated erythrocytes, a model cellular system of endogenously generated peroxyl radicals, SMe1EC2 significantly exceeded the parent stobadine in its antioxidant action. Considering the reported results of preclinical studies of SMe1EC2 showing its profound neuroprotective effects and low toxicity, the compound represents an example of a potential pharmacologically practicable antioxidant drug.
Introduction
Novel substituted hexahydropyridoindoles, designed as substitution derivatives of the antioxidant drug stobadine,Citation1–Citation4 were recently synthesized and characterized as efficient chain-breaking antioxidants.Citation5–Citation7 Modification of the stobadine molecule by aromatic electron-donating substitution was reported to enhance the intrinsic free radical scavenging activity, while variations of the N2 substituent afforded a synthetically accessible way to modulate the biological availability by affecting both lipophilicity and basicity of the molecule, without changing significantly the free radical scavenging activity.Citation5–Citation7
Among the novel derivatives, the compound (±)-cis-8-methoxy-2,3,4,4a,5,9b-hexahydro-1H-pyrido[4,3-b] indole-2-carboxylic acid ethyl ester (SMe1EC2) () has been subject of extensive preclinical studies. It revealed significant neuroprotection in the murine model of acute head traumaCitation6,Citation8 and in vitro rat hippocampal slices exposed to transient hypoxia/reoxygenation.Citation9–Citation11 Under conditions of experimental diabetes of rats, SMe1EC2 attenuated endothelial injury and restored the reduced endothelium-mediated relaxation in diabetic animals.Citation12 SMe1EC2 improved the viability of HT22 neuronal cells in culture exposed to high glucose and attenuated indices of oxidative stress.Citation13 The compound protected efficiently rat pancreatic INS-1E β cell cultures against cytotoxic effects of hydrogen peroxide.Citation14 Preclinical toxicology tests, revealed a remarkably low acute toxicity of SMe1EC2 in mice, regardless the way of administration.Citation6 Contrary to stobadine, SMe1EC2 did not possess any alpha-adrenolytic action.Citation6 In a prenatal developmental toxicity study in rats, SMe1EC2 demonstrated neither embryotoxic nor teratogenic effects on rat fetuses and no signs of maternal toxicity were found.Citation15
Figure 1. Chemical structure of stobadine (−)-cis-2,8-dimethyl-2,3,4,4a,5,9b-hexahydro-1H-pyrido[4,3b]indole (a) and SMe1EC2 (±)-cis-8-methoxy-2,3,4,4a,5,9b-hexahydro-1H-pyrido[4,3-b]indole-2-carboxylic acid ethyl ester (b).
![Figure 1. Chemical structure of stobadine (−)-cis-2,8-dimethyl-2,3,4,4a,5,9b-hexahydro-1H-pyrido[4,3b]indole (a) and SMe1EC2 (±)-cis-8-methoxy-2,3,4,4a,5,9b-hexahydro-1H-pyrido[4,3-b]indole-2-carboxylic acid ethyl ester (b).](/cms/asset/1b4a4cc6-25f4-43d4-881d-f4411dd299e3/yrer_a_11700784_f0001_b.jpg)
In this study, the antioxidant action of SMe1EC2 was investigated in a greater detail. First, the compound was subjected to the 1,1′-diphenyl-2-picrylhydrazyl (DPPH) test, which reflects the intrinsic chemical reactivity of a compound with the stable free radical of DPPH. Second, the overall antioxidant action of the compound was studied in the cellular system of isolated rat erythrocytes oxidatively stressed by free radicals derived from hydrophilic azoinitiator 2,2′-azobis(2-amidinopropane) hydrochloride (AAPH) or lipophilic tert-butyl hydroperoxide (t-BuOOH). Finally, consequences of structural changes relative to the parent stobadine are discussed in relation to the antiradical activity, acidobasic behavior, and cellular availability.
Materials and methods
Chemicals and instruments
SMe1EC2 and stobadine () were synthesized at the Institute of Experimental Pharmacology and Toxicology, Slovak Academy of Sciences, and were available as hydrochlorides. AAPH was obtained from FLUKA Chemie GmbH (Steinheim, Germany), t-BuOOH and trolox were purchased from Sigma-Aldrich Chemie GmbH (Steinheim, Germany). Melatonin, carnosine, DPPH were obtained from Sigma Chemical Co. (St Louis, MO, USA). Other chemicals were purchased from local commercial sources and were of analytical grade quality. Spectrophotometric analysis was performed using a Hewlett-Packard Diode Array Spectrophotometer 8452A.
Animals
Male Wistar rats, 8–9 weeks old, weighing 200–250 g, were used. The animals came from the Breeding Facility of the Institute of Experimental Pharmacology, Dobra Voda (Slovak Republic). This study was approved by the Ethics Committee of the Institute and performed in accordance with the Principles of Laboratory Animal Care (NIH publication 83–25, revised 1985) and the Slovak law regulating animal experiments (Decree 289, Part 139, 9 July 2003).
DPPH test
To investigate the antiradical activity of the pyridoindole derivatives and trolox, ethanolic solution of DPPH (50 µM) was incubated in the presence of the compound tested (50 µM; water solution) at laboratory temperature. The absorbance decrease at λmax = 518 nm during the first 1-minute interval was taken as a measure of antiradical activity.
Preparation of packed erythrocytes
The animals in light ether anesthesia were killed by exsanguination of the carotid artery. The blood was collected in 3.8% sodium citrate (1 vol. of sodium citrate: 9 vol. of blood) and centrifuged at 500 g for 15 minutes at 4°C. Plasma and white blood cells were removed by aspiration. The retrieved erythrocytes were washed three times with 6 vol. of ice-cold phosphate buffered saline (PBS, pH 7.4, 1.9 mM NaH2PO4, 8.1 mM Na2HPO4, and 150 mM NaCl). The entire procedure was conducted at 0–4°C. After the last washing, the red blood cells were used for further studies. The erythrocyte suspensions used in the experiments were prepared daily.
Hemolysis measurements
The hemolysis studies were performed in rat erythrocyte suspensions in PBS with the hematocrit of 1.5%. SMe1EC2, stobadine, melatonin, carnosine, and AAPH were dissolved in PBS directly. Trolox was dissolved in distilled water and titrated with 5 mM KOH. Further dilutions were made with PBS. The compounds studied were added from stock solutions in PBS to the erythrocyte suspensions to the final concentrations as reported below. Controls received an equivalent volume of PBS alone. Samples were then incubated for 30 minutes at 37°C. AAPH (30 mM final concentration) or t-BuOOH (250 µM final concentration) were added to samples and incubation continued at 37°C up to 4 hours. Aliquots were withdrawn after different time periods. The incubations were terminated by cooling the suspensions in an ice bath followed by centrifugation at 700 g for 10 minutes. The degree of hemolysis was estimated by spectrophotometry of the hemoglobin released into the supernatant fraction as described by Winterbourn.Citation16 The results were calculated as percentage of hemolysis. Total hemolysis (100%) was obtained by incubation of control erythrocytes in 10 mM hypotonic phosphate buffer, pH 7.4 (1.9 mM NaH2PO4, 8.1 mM Na2HPO4) at 37°C for 1 hour. Lag period was determined as an x-axis intersection of the ascending part of the semilogarithmic plot ‘hemolysis (%) vs. log t’.
Computations
Predictions of partition coefficients (log P), distribution ratios (log D), and dissociation constants (pKa) were made by Pallas software Version 3.1.1.2 (CompuDrug International, Sedona, AZ, USA).
Results
DPPH test
In order to determine the radical-scavenging potential of the compounds tested, the reactivity toward the stable free radical DPPH was measured by continual absorbance decrease of ethanol solution of DPPH containing the derivative tested at 518 nm (). The initial velocity of DPPH decolorization by 50 µM SMe1EC2 (0.507 ± 0.003 OD (optical density)/minute) was comparable with that of equimolar trolox (0.494 ± 0.009 OD/minute). The parent stobadine at 50 µM concentration was about three times less efficient (0.156 ± 0.019 OD/minute).
Hemolysis of rat erythrocytes in vitro
As shown in , rat erythrocytes exposed to AAPH or t-BuOOH underwent progressive hemolysis, determined by measuring the release of hemoglobin. The onset of free radical-induced hemolysis was shifted from the starting zero point by the time interval assigned as a lag period. In the presence of the compounds studied, the lag period increased.
Figure 3. Typical hemolysis curves induced by (a) AAPH or (b) t-BuOOH. Erythrocyte suspensions (1.5%) were incubated (a) with 30 mM AAPH in the presence of 10 µM SMe1EC2 (-•-) or (b) with 250 µM tBuOOH in the presence of 2.5 µM SMe1EC2 (-•-). Control incubations, (-Δ-). Each value is the mean ± SD from three to six experiments.
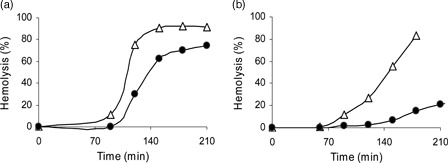
summarizes the antioxidant effects of SMe1EC2 and stobadine in isolated rat erythrocytes oxidatively stressed by AAPH- or t-BuOOH-derived peroxyl radicals. In the first case, when red blood cells were treated with hydrophylic AAPH azoinitiator, SMe1EC2 was found to be less protective than equimolar stobadine both at 10 and 25 µM concentration. Antioxidant activity of 25 µM SMe1EC2 (lag time 124.0 ± 9.6 minutes) was comparable with that of the reference trolox at 100 µM concentration (lag time 129.1 ± 10.1 minutes). On the other hand, when lipophilic t-BuOOH was applied, SMe1EC2 protected red blood cells more efficiently than equimolar stobadine both at 2.5 and 10 µM concentration. SMe1EC2 at 2.5 µM concentration was more efficient (lag time 129.9 ± 5.5 minutes) than 10 mM carnosine (lag time 105.7 ± 7.7 minutes) used as a reference. Another standard, melatonin, at 100 µM concentration executed no effect.
Figure 4. SMe1EC2 (▪) and stobadine (□) protect rat erythrocytes against (a) AAPH- or (b) t-BuOOH-induced hemolysis. Results are presented as means ± SD from three to six experiments. (a) ***P < 0.001 vs. (C) and (▪),###P < 0.001 vs. (C), parametric Student's t-test for independent samples; (b) ***P < 0.001 vs. (C) and (□), ###P < 0.001 vs. (C), parametric Student's t-test for independent samples.
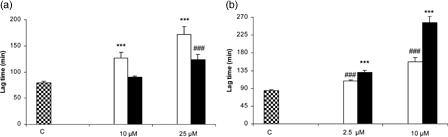
Discussion
The novel substituted hexahydropyridoindoles, the structural congeners of the antioxidant drug stobadine, have been postulated as chain-breaking antioxidants.Citation5,Citation7,Citation17–Citation21 The molecule center of the antioxidant activity was identified to reside at the indolic nitrogen.Citation7 Structural alterations in the close proximity of the indolic nitrogen, especially aromatic substitution in positions o and p, were found to influence the antioxidant efficacy.Citation5,Citation7 Moreover, alteration in the synthetically accessible position N2 provides the opportunity to vary basicity and lipophilicity of the compounds, thus modifying bioavailability, without affecting the intrinsic chemical reactivity with free radicals.Citation5
The subject of this study was SMe1EC2. The compound was projected with the aim to increase the free radical scavenging activity compared to the parent stobadine by substituting the aromatic methyl group with the more electron-donating methoxy group. At the same time, for the sake of bioavailability improvement, the basicity decrease was achieved by replacement of the methyl substituent in position N2 by an appropriate acyl substituent designed so that the lipophilicity of the parent molecule would not change significantly.
DPPH, as a weak hydrogen atom abstractor, is considered a good kinetic model for peroxyl radicals.Citation22 SMe1EC2 was found to rapidly react with DPPH, its activity being about three times higher than that of equimolar stobadine and comparable with that of the standard trolox. In homogeneous cell-free systems, antioxidant activity reflects an intrinsic chemical reactivity towards radicals. In membranes, however, the relative reactivity of antioxidants may be different since it is determined also by additional factors such as mutual location of the antioxidant and radicals at the membrane, ruled predominantly by their actual distribution ratios between water and lipid compartments.
In this study, isolated rat erythrocytes were used as a cellular model. Peroxidation of plasma membrane eventually resulting in hemolysis was induced by the hydrophilic AAPH,Citation23 which simulates an attack by free peroxyl radicals from the outside aqueous region. In addition, lipophilic t-BuOOH generating peroxyl radical intracellularly,Citation24,Citation25 was used.
At the concentrations studied, SMe1EC2 showed an inhibition period (lag phase) in the hemolysis kinetic curves (). The presence of distinct lag phase is indication of a much higher reaction rate of antioxidant molecules with initiating radicals compared to the rate of chain propagation. In the case of AAPH-induced hemolysis, SMe1EC2 was found significantly less efficient than stobadine (a). Yet, SMe1EC2 did exceed stobadine in its antioxidant action when lipophilic t-BuOOH was used to initiate the hemolysis (b). To account for the apparent discrepancy, the variance of basicity of SMe1EC2 vs. stobadine should be taken into consideration as indicated below.
Obviously, when considering biological availability of a drug in general, the molecule has not only to be lipophilic enough, as characterized by corresponding log P, but also neutral at the actual pH, to be able to penetrate into the lipid phase. Based on partition coefficients of SMe1EC2 and stobadine, their molecules are of a very similar lipophilicity, characterized by the respective log P values of 1.95 and 1.79 (calculated by the Pallas software). At pH 7.4, however, with regard to the variance of basicity of the proton-binding center represented by the piperidine (N2) nitrogen, their actual distribution ratios may differ profoundly. The indoline nitrogen, with very low pKa (for stobadine pKa1 = 3.226), remains unprotonated at pH 7.4.
For stobadine, as a high basicity N-methyl derivative with pKa2 = 8.5,Citation26 an acidobasic equilibrium with respect to the piperidine nitrogen is expected to be strongly shifted to its protonation at pH 7.4 (92.1% participation of the protonated form as calculated for its pKa2 = 8.5). The high degree of protonation of the N-methyl derivatives at physiological pH is expected to be reflected by low actual distribution ratios in spite of rather high partition coefficients (e.g. for stobadine, calculated log D = −0.05 vs. log P = 1.95; experimental log D = 0.57 ± 0.0320 at pH 7.4). In contrast, for the SMe1EC2 molecule, the acyl substituent at N2 lowers the basicity of this site profoundly. According to calculations, its pKa value corresponding to piperidinic nitrogen may be expected to be around –3.7, while pKa of the indoline nitrogen remains as low as 5.4. Therefore the protonation of both nitrogens in SMe1EC2 is negligible at physiological pH, which is reflected by high actual distribution ratios at pH 7.4, reaching almost the value of the partition coefficient (calculated log D = 1.78 vs. log P = 1.79).
The above-mentioned acidobasic equilibria of the compounds studied may have major consequences for their overall antioxidant action at cellular level. When peroxyl radicals were generated in the medium outside the red blood cells by thermal decomposition of hydrophilic AAPH initiator, stobadine was found to be more efficient than SMe1EC2. On the other hand, when peroxyl radicals were generated inside the red blood cells, by degradation of the lipophilic t-BuOOH, it was SMe1EC2 that was found more protective compared to stobadine.
Conclusion
The results proved high antiradical efficacy of SMe1EC2. In the system of t-BuOOH/isolated erythrocytes, which is considered a useful model for membrane perturbations characteristic of endogenously generated oxidative disorders, SMe1EC2 significantly exceeded the parent stobadine in its antioxidant action. Considering the reported results of preclinical studies of SMe1EC2 showing its profound neuroprotective effects and low toxicity, the compound represents an example of potential pharmacologically practicable antioxidant drug. In addition, the present outcomes in the context of preceding findings indicate that the hexahydropyridoindole skeleton represents an interesting scaffold. Its modification may yield congeners tailored according to specific requirements for antiradical efficacy, lipophilicity, and basicity, meeting the aim of providing a pharmacologically practicable antioxidant drug.
Acknowledgements
This study was supported by the grant VEGA 2/0067/11. We are thankful to Dr Anna Tsantili-Kakoulidou for the permission to use the Pallas program.
References
- Horakova L, Sies H, Steenken S. Antioxidant action of stobadine. Methods Enzymol 1994;234:572–80.
- Horakova L, Stolc S. Antioxidant and pharmacodynamic effects of pyridoindole stobadine. Gen Pharmacol 1998;30:627–38.
- Juranek I, Horakova L, Rackova L, Stefek M. Antioxidants in treating pathologies involving oxidative damage: an update on medicinal chemistry and biological activity of stobadine and related pyridoindoles. Curr Med Chem 2010;17:552–70.
- Juranek I, Rackova L, Stefek M. Stobadine – an indole type alternative to the phenolic antioxidant reference trolox. In: , Ekinci D, (ed.) Biochemistry. Rijeka: InTech; 2012. p. 443–52.
- Rackova L, Snirc V, Majekova M, Majek P, Stefek M. Free radical scavenging and antioxidant activities of substituted hexahydropyridoindoles. Quantitative structure-activity relationships. J Med Chem 2006;49:2543–8.
- Stolc S, Povazanec F, Bauer V, Majekova M, Wilcox AL, Snirc V, et al. Pyridoindole derivatives with antioxidant properties: synthesis, therapy and pharmaceutical remedies. Slovak Patent Agency; 2010: P 287506.
- Rackova L, Stefek M, Majekova M. Structural aspects of antioxidant activity of substituted pyridoindoles. Redox Rep 2002;7(4):207–14.
- Stolc S, Snirc V, Majekova M, Gasparova Z, Gajdosikova A, Stvrtina S. Development of the new group of indole-derived neuroprotective drugs affecting oxidative stress. Cell Mol Neurobiol 2006;26(7–8):1495–504.
- Gasparova Z, Janega P, Babal P, Snirc V, Stolc S, Mach M, et al. Effect of the new pyridoindole antioxidant SMe1EC2 on functional deficits and oedema formation in rat hippocampus exposed to ischaemia in vitro. Neuro Endocrinol Lett 2009;30:574–81.
- Gasparova Z, Ondrejickova O, Gajdosikova A, Gajdosik A, Snirc V, Stolc S. Oxidative stress induced by the Fe2+/ascorbic acid system or model ischemia in vitro: effect of carvedilol and pyridoindole antioxidant SMe1EC2 in young and adult rat brain tissue. Interdiscip Toxicol 2010;3:122–6.
- Gasparova Z, Snirc V, Stolc S. The new pyridoindole antioxidant SMe1EC2 and its intervention in hypoxia/hypoglycemia-induced impairment of longterm potentiation in rat hippocampus. Interdiscip Toxicol 2010;4:56–61.
- Sotnikova R, Nedelcevova J, Navarova J, Nosalova V, Drabikova K, Szocs K, et al. Protection of the vascular endothelium in experimental situations. Interdiscip Toxicol 2011;4:20–6.
- Rackova L, Snirc V, Jung T, Stefek M, Karasu C, Grune T. Metabolism-induced oxidative stress is a mediator of glucose toxicity in HT22 neuronal cells. Free Radic Res 2009;43:876–86.
- Rackova L, Cumaoglu A, Bagriacik EU, Stefek M, Maechler P, Karasu C. Novel hexahydropyridoindole derivative as prospective agent against oxidative damage in pancreatic β cells. Med Chem 2011;7:711–7.
- Ujhazy E, Dubovicky M, Ponechalova V, Navarova J, Brucknerova I, Snirc V, et al. Prenatal developmental toxicity study of the pyridoindole antioxidant SMe1EC2 in rats. Neuro Endocrinol Lett 2008;29(5):639–43.
- Winterbourn CC. Oxidative reactions of hemoglobin. Meth Enzymol 1990;186:265–72.
- Stefek M, Benes L. Pyridoindole stobadine is a potent scavenger of hydroxyl radicals. FEBS Lett 1991;294:264–6.
- Steenken S, Sunquist AR, Jovanovic SV, Crockett R, Sies H. Antioxidant activity of the pyridoindole stobadine. Pulse radiolytic characterization of one-electron-oxidized stobadine and quenching of singlet molecular oxygen. Chem Res Toxicol 1992;5:355–60.
- Stefek M, Masarykova M, Benes L. Inhibition of cumene hydroperoxide-induced lipid peroxidation by a novel pyridoindole antioxidant in rat liver microsomes. Pharmacol Toxicol 1992;70:407–11.
- Kagan VE, Tsuchiya M, Serbinova E, Packer L, Sies H. Interaction of the pyridoindole stobadine with peroxyl, superoxide and chromanoxyl radicals. Biochem Pharmacol 1993;45:393–400.
- Stefek M, Trnkova Z. The pyridoindole antioxidant stobadine prevents alloxan-induced lipid peroxidation by inhibiting its propagation. Pharmacol Toxicol 1996;78:77–81.
- Blois MS. Antioxidant determinations by the use of a stable free radical. Nature 1958;181:1199–200.
- Niki E. Free radicals initiators as source of water- or lipid-soluble peroxyl radicals. Methods Enzymol 1990;186:100–8.
- Trotta RJ, Sullivan SG, Stern A. Lipid peroxidation and haemoglobin degradation in red blood cells exposed to t-butyl hydroperoxide. The relative roles of haem- and glutathione-dependent decomposition of t-butyl hydroperoxide and membrane lipid hydroperoxides in lipid peroxidation and haemolysis. Biochem J 1983;212(3):759–72.
- Thornalley PJ, Trotta RJ, Stern A. Free radical involvement in the oxidative phenomena induced by tert-butyl hydroperoxide in erythrocytes. Biochim Biophys Acta 1983;759(1–2):16–22.
- Stefek M, Benes L, Zelnik V. N-oxygenation of stobadine, a gamma-carboline antiarrhytmic and cardioprotective agent: the role of flavin-containing monooxygenase. Xenobiotica 1989;19:143–50.