Abstract
Malignant mesothelioma (MM) is a fatal neoplastic disease with no therapeutic option. Therefore, the search for novel therapies is of paramount importance.
Methods
Since mitochondrial targeting of α-tocopheryl succinate (α-TOS) by its tagging with triphenylphosphonium enhances its cytotoxic effects to cancer cells, we tested its effect on MM cells and experimental mesotheliomas.
Results
Mitochondrially targeted vitamin E succinate (MitoVES) was more efficient in killing MM cells than α-TOS with IC50 lower by up to two orders of magnitude. Mitochondrial association of MitoVES in MM cells was documented using its fluorescently tagged analogue. MitoVES caused apoptosis in MM cells by mitochondrial destabilization, resulting in the loss of mitochondrial membrane potential, generation of reactive oxygen species, and destabilization of respiratory supercomplexes. The role of the mitochondrial complex II in the activity of MitoVES was confirmed by the finding that MM cells with suppressed succinate quinone reductase were resistant to MitoVES. MitoVES suppressed mesothelioma growth in nude mice with high efficacy.
Discussion
MitoVES is more efficient in killing MM cells and suppressing experimental mesotheliomas compared with the non-targeted α-TOS, giving it a potential clinical benefit.
Introduction
Malignant mesothelioma (MM), a fatal type of neoplasia,Citation1 is associated with asbestos inhalation. The lack of early diagnosis together with the aggressive nature of MM is the main reason for short survival of patients after diagnosis.Citation2–Citation4 It is therefore important to search for novel therapeutic approaches that would be efficient against MM while being selective for tumour cells. In this context, mitochondria have emerged as an intriguing target for anti-cancer agents.Citation5,Citation6 We have defined mitocans, a group of compounds with anti-cancer activity acting via mitochondrial destabilization, often in a cancer-specific manner, and classified them into several groups based on their molecular mode of activity.Citation7 Of these, group 5 mitocans target the electron transfer chainCitation8 with vitamin E (VE) analogues as prime examples.Citation9
VE analogues are epitomized by the redox-silent agent α-tocopheryl succinate (α-TOS), which suppresses several types of tumours in animal models.Citation9–Citation13 The agent acts by mitochondrial destabilization, resulting in the generation of reactive oxygen species (ROS).Citation14,Citation15 The molecular targets of α-TOS are ubiquinone (UbQ)-binding sites of the mitochondrial complex II (CII).Citation16,Citation17 Interaction of α-TOS with CII's UbQ sites results in the generation of superoxide anion radicals that activate the Mst1 kinase, followed by phosphorylation of the transcription factor FoxO1 and upregulation of the Noxa protein with ensuing departure of Mcl-1 from Bak, the latter forming pores in the mitochondrial outer membrane.Citation18,Citation19 The notion that α-TOS targets the UbQ sites in CII prompted us to modify it by tagging with the mitochondria-targeting triphenylphosphonium (TPP+) group, as initially accomplished for antioxidants like UbQ.Citation20 We have generated mitochondrially targeted VE succinate (MitoVES) that is considerably more efficient in killing cancer cells as well as suppressing experimental carcinomas compared with the parental compound.Citation21,Citation22
Given the grim prognosis of MM patients and the finding that α-TOS is efficient against experimental MM,Citation12,Citation13 we now tested the effect of MitoVES on a range of MM cell lines and on experimental mesotheliomas. Here, we show that the agent is considerably more efficient than its untargeted counterpart, giving it clinical significance.
Materials and methods
Cell culture and treatment
Human Ist-Mes-2, MM-BI cells, Meso-2, and H28 cells,Citation23 and murine AE17 MM cellsCitation24 were maintained in Dulbecco's modified Eagle's medium (DMEM) or RPMI media supplemented with 10% fetal calf serum and antibiotics at 37°C in a humidified atmosphere of 5% CO2. Cells were seeded and allowed to reach 60–70% confluency before addition of VE analogues (). They include α-TOS (Sigma, St Louis, MO, USA), MitoVES with different length of the chain spanning the TPP+ group and the tocopheryl group, and an analogue of α-TOS with the TPP+ group esterified to the free carboxylate of the succinyl moiety via a 4-C spacer.Citation21,Citation22 Unless specified, MitoVES refers to the 11-C chain homologue. Fluorescently labelled MitoVES (MitoVES-F) and α-TOS (α-TOS-F) were synthesized as described.Citation21,Citation22
Cell death and viability assay
Cell death was assessed as detailed elsewhereCitation14 using the annexin V-FITC/propidium iodide (PI) method. Cell death is expressed as the sum of cells in the stage of apoptosis and post-apoptotic necrosis. Viability was estimated using the crystal violet method according to a standard procedure. In brief, MM cells were seeded in 96-well plates at 104 cells per well, allowed to adhere, and treated with VE analogues for 24 hours. The cells were then fixed with formalin and stained with 0.05% crystal violet for 1 hour at room temperature. Absorbance at 595 nm was determined using the Tecan Infinity plate reader.
Assessment of mitochondrial potential (ΔΨm,i) and generation of ROS
The ΔΨm,i-sensitive fluorescent probe tetramethylrhodamine methyl ester (TMRM, Sigma) was utilized, and the cells were evaluated by flow cytometry. The mitochondrial uncoupler carbonyl cyanide 4-(trifluoromethoxy)phenyl-hydrazone (CCCP, Sigma) was used as a control to determine non-specific TMRM loading.
ROS were evaluated using the probes dihydroethidium (DHE), 2′,7′-dichlorodihydrofluorescein diacetate (DCF), or MitoSOX (Sigma). The cells were incubated with either probe for 15 minutes under normal culture conditions, harvested, and the fluorescence of the oxidized probes was assessed by flow cytometry.
Preparation of SDHC-deficient cells
Ist-Mes-2 cells were seeded in 24-well plates at 2 × 105 cells per well in antibiotic-free DMEM with 10% fetal calf serum and allowed to recuperate overnight. The SureSilencing human SDHC shRNA or non-silencing (NS) plasmid (SuperArray Biosciences, Doncaster, Victoria, Australia) was combined with the Fugene HD transfection reagent and added to the cells. After 48 hours, the cells were incubated with 4 µg/ml of puromycin for 2 weeks to select the transfected cells. Successful transfection was detected by assessing the level of the SDHC protein using western blotting (WB).
Native blue electrophoresis and western blotting
Cells were harvested, resuspended in the Tris/ethylene glycol tetraacetic acid buffer containing 200 mM sucrose, and homogenized on ice with a glass-Teflon homogenizer. Mitochondria were isolated by differential centrifugation and solubilized in the extraction buffer (1.5 M aminocaproic acid, 50 mM Bis-Tris, 0.5 M ethylenediaminetetraacetic acid (EDTA), pH 7) with 1.3% lauryl maltoside. Samples containing 30–60 µg of protein were combined with the sample buffer (0.75 M aminocaproic acid, 50 mM Bis-Tris, 0.5 M EDTA, pH 7, 5% Serva-Blue G-250, 12% glycerol), loaded on pre-cast NativePAGE Novex 4–16% Bis-Tris gels, and run overnight at 25 V. Separated protein complexes were transferred to polyvinylidene difluoride membranes using the Transblot system (Invitrogen, Mulgrave, Victoria, Australia). Individual complexes were detected using the following antibodies (Abcam, Cambridge, MA, USA): CI, anti-NDUFS1 (79 kDa); CII, anti-SDHA (70 kDa); CIII, anti-UQCRC2 (48 kDa); CIV, anti-MTCO2 (26 kDa); CV, anti-ATP5A (60 kDa). Anti-VDAC IgG (Abcam) was used as a loading control.
For WB following sodium dodecyl sulphate–polyacrylamide gel electrophoresis, the anti-SDHC IgG (Santa Cruz Biotechnology, Dallas, TX, USA) was used. Anti-actin IgG (Sigma) was used as a loading control.
In-gel CI and CII activity assay
Isolated mitochondria were lysed and subjected to clear native electrophoresis, run as above but without the blue dye. CI activity was assessed on 3–12% gel strips using the NADH:NTB reductase assay. This gel strip was evaluated in freshly prepared NADH:NTB reductase assay buffer (25 mg NTB and 100 µl of NADH, 10 mg/ml, added to 10 ml 5 mM Tris/HCl, pH 7.4); after 10–15 minutes, the gel was transferred to 5 mM Tris/HCl, pH 7.4 and scanned. CII activity was evaluated on 4–16% gel strips using the succinate:NTB reductase assay. The gel strip was incubated for 10–20 minutes in freshly prepared succinate:NTB reductase assay buffer (25 mg NTB, 200 µl 1 M sodium succinate, 8 µl of 250 mM phenazine methosulphate dissolved in dimethylsulfoxide, added to 10 ml 5 mM Tris/HCl, pH 7.4). The gel was immediately transferred to 5 mM Tris/HCl, pH 7.4 and scanned.
Mitochondrial respiration and CII activity
Cellular respiration was evaluated using the Oxygraph-2 k apparatus (Oroboros Instruments) as published.Citation25 Experiments evaluating intact cell respiration were performed with MM cells suspended in the complete RPMI medium. Experiments evaluating digitonin-permeabilized cell oxygen consumption were performed with MM cells suspended in the mitochondrial respiration medium MiR05. Respiration via CI and CII was evaluated in the presence of the proper substrates and inhibitors of the other complex. Increasing concentrations of the tested compounds were added to the Oxygraph chamber and the total oxygen concentration and consumption were recorded. The results were related to the number of cells.
The succinate quinone reductase (SQR) and succinate dehydrogenase (SDH) activities of CII were assessed as detailed elsewhere.Citation21
Confocal microscopy
Cells were seeded at 105 cells per chamber slide (Nunc, Rochester, NY, USA) and allowed to attach overnight. The cells were then incubated with 100 nM TMRM and 5 µg/ml DAPI for 1 hour. The cells were then incubated with 5 µM MitoVES-F or α-TOS-F for 5 minutes. Imaging was performed using the Olympus FV1000 fluorescent confocal microscope. In some cases, the cells were pre-treated with 2 µM CCCP.
Animal experiments
Athymic Balb-c nu/nu mice were injected subcutaneously with freshly harvested 2 × 106 Ist-Mes-2 cells suspended in 100 µl of saline. After about 15 days when tumours established (∼30 mm3), the mice were injected into the peritoneum twice per week with 15 µmol α-TOS or 1 µmol MitoVES dissolved in corn oil after solubilization in ethanol (EtOH) (the final concentration of EtOH, 4% v/v).Citation26 Tumours were visualized and quantified non-invasively using the ultrasound imaging (USI) instrument Vevo770 (VisualSonics, Toronto, Ontario, Canada) fitted with a 30 µm resolution 80 MHz scan-head.Citation26 All experiments were performed according to the guidelines of the Australian and New Zealand Council for the Care and Use of Animals in Research and Teaching and were approved by the Griffith University Animal Ethics Committee.
Statistical analysis
Experimental data are presented as mean ± SD Comparisons between groups were performed using the Mann–Whitney U-test for unpaired samples and Kruskall–Wallis analysis for multiple comparisons. Statistical calculations were performed using the SPSS statistical package version 12.0F. Statistical differences of at least P < 0.05 were considered statistically significant.
Results and discussion
We report on the effect of a novel anti-tumour agent MitoVESCitation21,Citation22 on MM cells and experimental mesotheliomas. This follows from earlier findings that the parental compound of MitoVES, α-TOS, suppresses tumours in multiple models of cancerCitation9–Citation13 and that it relays its effect via mitochondria by the interaction with the UbQ sites of CII.Citation16,Citation17 Addition of the TPP+ group to the parental compound caused a higher killing of tumour cells, considerably enhancing its efficacy.Citation21,Citation22 Since MM is a fatal disease,Citation1–Citation3,Citation27 MitoVES has now been tested as a potentially useful MM therapeutic. MM cell lines H28, Meso-2, Ist-Mes-2, MM-BI, and AE17 were exposed to α-TOS and MitoVES. documents that the IC50 values were by up to two orders of magnitude lower for MitoVES than for α-TOS. While MitoVES killed almost all cells within 24 hours at >2.5 µM, similar efficacy of killing was achieved with 50–100 µM α-TOS (A and B).
Figure 2. MitoVES is toxic to MM cells and causes ROS generation and ΔΨm,i dissipation. Ist-Mes-2, MM-BI, Meso-2, H28, and AE17 cells were seeded and allowed to reach 60–70% confluency. The cells were then incubated with α-TOS or MitoVES for 24 hours at the concentrations shown, and viability assessed by the crystal violet (A) and cell death by the annexin V/PI method (B). Panels (C–E) show increase in the level of ROS, evaluated as mean fluorescence intensity (MFI), assessed in MM cells exposed to MitoVES (5 µM) and α-TOS (100 µM) using the fluorescent probes DHE, DCF, and MitoSOX, respectively, and flow cytometry. Panel (F) reveals the relative levels of ΔΨm,i in MM cells exposed to MitoVES (5 µM) and α-TOS (100 µM). The data are mean values ± SD (n = 3).
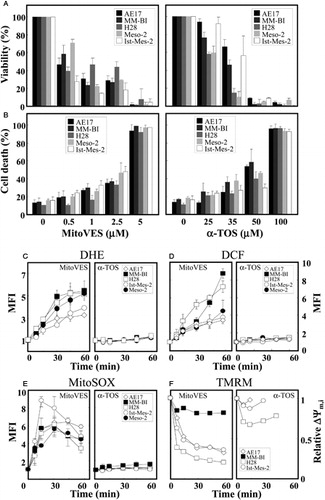
Table 1. IC50 values for α-TOS and MitoVES for MM cell lines*
α-TOS and MitoVES exert their effect on cancer cells largely via mitochondria.Citation11,Citation14,Citation15,Citation21,Citation22 We tested whether the agents cause generation of ROS and dissipation of ΔΨm,i in MM cells. At 5 µM, MitoVES generated high levels of ROS within <10 minutes, consistent with earlier data for neuroblastoma cells transfected with a redox-sensitive plasmid.Citation22 α-TOS generated very low levels of ROS in MM cells at 100 µM and at 60 minutes (C–E). MitoVES caused rapid dissipation of ΔΨm,i in MM cell lines (F). However, the drop of ΔΨm,i was mild in MM-BI cells (F) and it increased in Meso-2 cells (not shown). We found that exposure of AE17 cells to the uncoupler CCCP (2 µM) resulted in a decrease of ΔΨm,i from the initial relative level of 1 to 0.34, for MM-BI, H28, and Ist-Mes-2 cells to 0.76, 0.24, and 0.31, respectively, which corresponds to its maximum decrease when cells were exposed to MitoVES. In Meso-2 cells, CCCP caused a mild increase in ΔΨm,i, from the relative level of 1–1.09. α-TOS caused only low and transient dissipation of ΔΨm,i (F). The differences in the effect of MitoVES and α-TOS on the level of ROS generation and ΔΨm,i indicate potentially different modes of action of the two agents. While MitoVES is directly targeted to mitochondria, α-TOS associates with a variety of lipophilic structures (cf. Dong et al.Citation21,Citation22, and see below). α-TOS may trigger apoptosis both by a direct effect on mitochondria and on lysosomes.Citation28 The effect of α-TOS on signalling pathways, such as shown for the FGF2 autocrine loop in MM cellsCitation12 or the c-jun pathway in leukaemia and breast cancer cellsCitation29,Citation30 can also play a role. Finally, it appears that MitoVES is capable of causing ROS generation even at initial low ΔΨm,i as long as it can be modulated by the agent.
Since the TPP+ group targets small molecules to mitochondria, we tested mitochondrial association of MitoVES in MM cells. Cells grown on cover slips were incubated with fluorescent analogues of MitoVES and α-TOS together with TMRM. A–D shows exclusive mitochondrial localization of MitoVES while α-TOS localized to non-mitochondrial compartments as well as to mitochondria. Dissipation of ΔΨm,i with 2 µM CCCP prior to incubation of Ist-Mes-2 cells with MitoVES caused a failure of exclusive mitochondrial localization of the agent (E) and suppressed apoptosis induced by MitoVES (F). This documents the importance of ΔΨm,i for efficient killing of MM cells.
Figure 3. Association of MitoVES with mitochondria and its cytotoxic activity in MM cells depend on their ΔΨm,i and on the structure of the agent. MM-BI (A), Meso-2 (B), Ist-Mes-2 (C), and H28 cells (D) were seeded on cover slips and incubated for 30 minutes with TMRM and MitoVES-F or α-TOS-F (both 5 µM). (E) Ist-Mes-2 cells were pre-treated for 10 minutes with 2 µM CCCP before the addition of VE analogues. The cells were then mounted in DAPI-containing Vectashield and images acquired in a confocal microscope. (F) Ist-Mes-2 cells were seeded, allowed to reach 60–70% confluency, pre-treated (where shown) with 2 µM CCCP for 10 minutes, exposed to α-TOS or MitoVES for 24 hours at the concentrations shown (μM), and evaluated for apoptosis using the annexin V/PI method. Ist-Mes-2 cells at 60–70% confluency were exposed to MitoVES analogues (5 µM) as shown for 60 minutes and evaluated for SQR activity (G), ROS generation using the DHE probe (H), and dissipation of ΔΨm,i using TMRM (I). Cell death induction was evaluated using the annexin V/PI method after 24-hour incubation (J). The images are representative of three independent experiments, data are mean values ± SD (n = 3); the symbol ‘*’ indicates significant differences with P < 0.05.
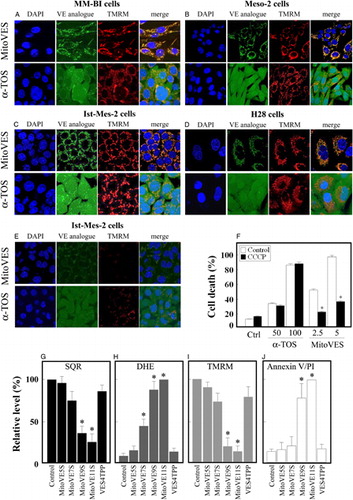
Figure 4. MitoVES suppresses mitochondrial respiration. AE17, MM-BI, H28, Ist-Mes-2, and Meso-2 cells were seeded and allowed to reach 60–70% confluency. (A) The cells were harvested and placed in the Oxygraph chamber at 106 per ml in the original medium. Oxygen consumption was assessed following addition of increasing concentration of α-TOS or MitoVES. (B) The cells were harvested, permeabilized, and placed in the Mir05 medium in the Oxygraph chamber at 2 × 106 per ml. Oxygen consumption linked to CI or CII was evaluated in the presence of increasing concentration of α-TOS or MitoVES following inhibition of CII or CI, respectively, and using the appropriate substrates as detailed in the Materials and methods section. The data are mean values ± SD (n = 3).
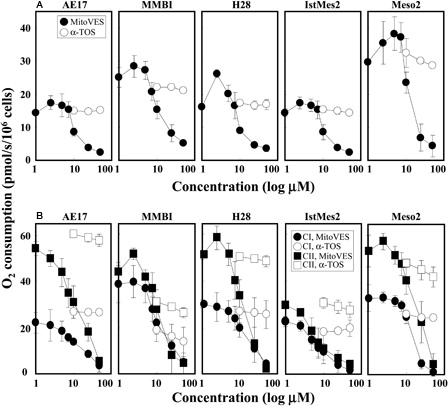
Mammalian cells comprise two complex II UbQ-binding sites, the proximal (Qp) and distal (Qd) site.Citation31 We proposed that while α-TOS can interact with both sites,Citation16 MitoVES, due to its localization with the charged TPP+ group in the matrix and the tocopheryl succinyl group in the mitochondrial inner membrane, can reach only the Qp site.Citation21 To assess importance of the length of the aliphatic chain for efficient activity of MitoVES towards MM, we tested homologues of MitoVES with a shorter aliphatic chain by two carbons at a time. The agents were evaluated for their effect on the SQR activity as well as their efficacy to cause ROS generation, ΔΨm,i dissipation, and apoptosis induction. G–J documents that the short-chain homologues were less active, with the C-9 homologue almost as efficient as the full-length MitoVES. We also tested the premise that a free carboxylate of the succinyl moiety of MitoVES is important for its activity.Citation9 G–J shows that MitoVES4TPP was inefficient, documenting that the free carboxyl group is needed for the interaction of MitoVES with the Qp site (serin 68)Citation31 of CII, as proposed earlier.Citation16,Citation21
The effect of small anti-cancer compounds on mitochondria can involve suppression of their respiration.Citation7 We tested this for α-TOS and MitoVES in MM cells, first assessing the effect of the two agents on routine respiration, i.e. total oxygen consumption by whole cells in the cultivation medium in the absence of an uncoupler. A documents much higher efficacy for MitoVES than that of α-TOS, which was relatively inefficient. Interestingly, low levels of MitoVES (1–2 µM) caused an initial increase in respiration, most likely due to mitochondrial uncoupling. This is consistent with experiments documenting uncoupling activity of MitoVES at low concentrations in intact cells and isolated mitochondria, with inhibition of respiration at its higher levels.Citation32 This inhibition is related to ROS generation in response to MitoVES and is compatible with CII as a source of radicals.Citation21,Citation22,Citation33,Citation34 We next tested the effect of MitoVES and α-TOS on the contribution of CI and CII to mitochondrial respiration in permeabilized cells. B indicates that MM cells respire predominantly via CII and reveals a superior effect of MitoVES, which at lower concentrations uncoupled CII-dependent respiration in several of the cell lines, followed by its sharp inhibition. The agent also exerted an effect on CI at higher levels, reminiscent of a recent paper showing inhibition of the CI activity by α-TOS in leukaemia cells.Citation35
In the next experiments, we tested the effect of the VE analogues on the assembly of mitochondrial (super)complexes. A reveals that mitochondrial complexes form supercomplexes in Ist-Mes-2 and H28 cells, referred to as respirasomes.Citation36 While the assembly of CII, the target for MitoVES, was not affected, the agent destabilized at 5 µM and in 1 hour, in particular, the higher forms of supercomplexes. This is obvious for the CI + CIII2 + CIV respirasome in H28 cells, which is thought to allow for direct transfer of electrons from CI to CIII and to CIV, and is the most active form of the three complexes,Citation36,Citation37 and the CI + CIII2 supercomplex in Ist-Mes-2 cells. α-TOS was much less efficient (A). Further, we found that MitoVES suppressed CII and CI activity using the in-gel assay following the separation of the mitochondrial fraction by clear native electrophoresis (B). Thus, MitoVES efficiently affects the assembly of mitochondrial supercomplexes in MM cells. This may be explained by the interaction of MitoVES with CII, which results in ROS generation and subsequent effect on mitochondrial (super)complexes, although the exact mechanism is yet to be established.
Figure 5. MitoVES affects the mitochondrial complexes assembly. Ist-Mes-2 and H28 cells were allowed to reach 60–70% confluency, after which they were exposed to α-TOS at 50 µM for 0.5 hours (1), 1 hour (2), or 2 hours (3) or at 25 µM for 24 hours (4), or to MitoVES at 5 µM for 0.5 hours (1), 1 hour (2), or 2 hours (3) or at 1 µM for 24 hours (4), and the mitochondrial fraction was prepared. (A) The mitochondrial fraction was subjected to native blue electrophoresis as detailed in the Materials and methods section. The text on the right indicates the position of (super)complexes and partially assembled complexes. (B) The mitochondrial fraction was separated using clear native electrophoresis and the CI and CII in-gel activities were assessed as detailed in the Materials and methods section. The activity related to the position of (super)complexes, as well as partially assembled complexes is indicated by the text on the right. The images are representative of three independent experiments.
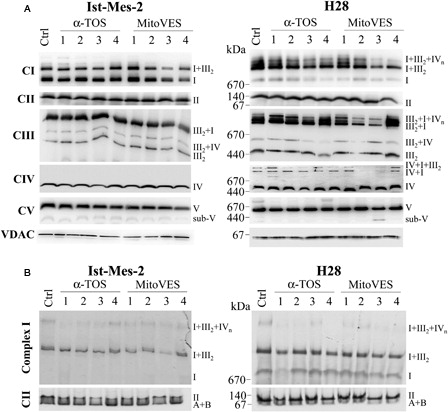
To unequivocally determine the importance of CII for the activity of MitoVES, we prepared MM cells in which its function was compromised by down-regulating the SDHC subunit of CII in Ist-Mes-2 cells by SDHC shRNA. A reveals a very low level of the SDHA protein and low SQR activity in the SDHC knock-down cells, while the SDH activity was only marginally lower. As expected, the CII-compromised cells respired less via CII compared with the control cells (B). Importantly, C shows low level of apoptosis in the SDHC knock-down cells, clearly pointing to CII as the molecular target of MitoVES in MM cells.
Figure 6. MitoVES kills MM cells by targeting CII and suppresses experimental MMs. Ist-Mes-2 cells were stably transfected with NS or SDHC shRNA as described in the Material and methods section, and evaluated for the level of the SDHC protein by WB and the activity of SQR and SDH (A), for respiration via CI and CII using the Oxygraph (B), and for the effect of MitoVES (5 µM) on cell death using the annexin V/PI method (C). (D) Nude mice were injected subcutaneously with 2 × 106 Ist-Mes-2 cells per animal. The mice were treated intraperitoneally with 15 µmol α-TOS or 1 µmol MitoVES dissolved in 4% EtOH in corn oil or with 100 µl of the excipient (control mice) twice per week. Tumours were quantified using USI and expressed relative to the initial tumour volume (∼20–30 mm3). The images are representative of three independent experiments, the data in panels (A–C) are mean values ± SD (n = 3). The symbol ‘*’ indicates significant differences with P < 0.05. The data in panel (D) are mean values ± SD (n = 3), the symbol ‘*’ indicates significant differences between values from control and MitoVES-treated animals, ‘**’ between α-TOS- and MitoVES-treated animals, with P < 0.05.
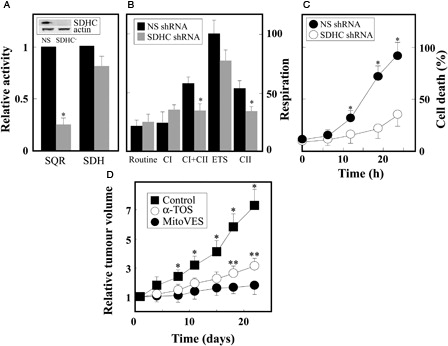
We next tested the effect of MitoVES on experimental MM, i.e. xenografts prepared in nude mice subcutaneously grafted with Ist-Mes-2 cells. D documents the superior effect of MitoVES compared with α-TOS, which was shown to inhibit MM earlier.Citation12,Citation13 At 1 µmol per dose per mouse given twice per week, MitoVES suppressed tumour progression by >90%, while α-TOS at 15 µmol was less efficient, with ∼80% tumour growth inhibition. We did not observe any obvious toxicity of the agent during the course of the experiment. The data on the effect of MitoVES on MM cells and, in particular, its high cytotoxic efficacy compared with that of the parental α-TOS, can be reconciled with our recent paper, in which we documented the development of resistance in cancer cells during their long-term exposure to α-TOS.Citation38 We found that escalating doses of α-TOS resulted in their resistance due to increased expression of the ABCA1 protein. This resistance was overcome by exposing the cells to MitoVES, which enters cells on the basis of their plasma membrane potential, bypassing the ABCA1 protein.Citation38 Recently, we treated a single MM patient with topical α-TOS. The patient survived by 4 years following diagnosis (as opposed to the predicted ∼6 months) with the tumour getting smaller. However, the tumour started to grow and the patient succumbed to the disease. It is possible that at this stage, the tumour developed resistance to α-TOS, which could be potentially overcome by MitoVES. Given our findings on α-TOS and MitoVES, we believe that the novel mitochondrial target, CII,Citation8,Citation16,Citation17,Citation21,Citation22 will be used in the future for such difficult-to-treat malignancies as is MM. In conclusion, since MM is a fatal malignancy with very few if any options beyond palliative therapy, our results on MitoVES are highly encouraging and of potential clinical benefit.
Acknowledgements
This project was supported in part by grants from the Australian Research Council of Australia, National Health and Medical Research Council of Australia, and Cancer Council Queensland to JN, and by grants P301/10/1937 and P305/12/1708 from Czech Science Foundation to J.N. and J.T., respectively. J.S. was supported in part by the Apoptosis Research Group of the School of Medical Science, Griffith University.
References
- Robinson BWS, Musk AW, Lake AR. Malignant mesothelioma. Lancet 2005;366(9483):397–408.
- Fennell DA, Gaudino G, O'Byrne KJ, Mutti L, van Meerbeeck J. Advances in the systemic therapy of malignant pleural mesothelioma. Nat Clin Pract Oncol 2008;5(3):136–47.
- Tomasetti M, Amati M, Santarelli L, Alleva R, Neuzil J. Malignant mesothelioma: biology, diagnosis and new therapeutic approaches. Curr Mol Pharmacol 2009;2(2):190–206.
- Hollevoet K, Reitsma JB, Creaney J, Grigoriu BD, Robinson BW, Scherpereel A, et al. Serum mesothelin for diagnosing malignant pleural mesothelioma: an individual patient data meta-analysis. J Clin Oncol 2012;30(13):1541–9.
- Gogvadze V, Orrenius S, Zhivotovsky B. Mitochondria in cancer cells: what is so special about them? Trends Cell Biol 2008;18(4):165–73.
- Fulda S, Kroemer G. Mitochondria as therapeutic targets for the treatment of malignant disease. Antioxid Redox Signal 2011;15(12):2937–49.
- Neuzil J, Dong LF, Rohlena J, Truksa J, Ralph SJ. Classification of mitocans, anti-cancer drugs acting on mitochondria. Mitochondrion 2013;13(3):199–208.
- Kluckova K, Bezework-Geleta A, Rohlena J, Dong L, Neuzil J. Mitochondrial complex II, a novel intriguing target for anti-cancer agents. Biochim Biophys Acta 2013;1827(5):552–64.
- Neuzil J, Weber T, Schröder A, Lu M, Ostermann G, Gellert N, et al. Induction of cancer cell apoptosis by α-tocopheryl succinate: molecular pathways and structural requirements. FASEB J 2001;15(2):403–15.
- Zhang Y, Ni J, Messing EM, Chang E, Yang CR, Yeh S. Vitamin E succinate inhibits the function of androgen receptor and the expression of prostate-specific antigen in prostate cancer cells. Proc Natl Acad Sci USA 2002;99(11):7408–13.
- Yu W, Sanders BG, Kline K. RRR-α-tocopheryl succinate-induced apoptosis of human breast cancer cells involves Bax translocation to mitochondria. Cancer Res 2003;63(10):2483–91.
- Stapelberg M, Gellert N, Swettenham E, Tomasetti M, Witting PK, Procopio A, et al. α-Tocopheryl succinate inhibits malignant mesothelioma by disrupting the FGF autocrine loop: the role of oxidative stress. J Biol Chem 2005;280(27):25369–76.
- Tomasetti M, Gellert N, Procopio A, Neuzil J. A vitamin E analogue suppresses malignant mesothelioma in a preclinical model: a future drug against a fatal neoplastic disease? Int J Cancer 2004;109(5):641–2.
- Weber T, Dalen H, Andera L, Nègre-Salvayre A, Augé N, Sticha M, et al. Mitochondria play a central role in apoptosis induced by α-tocopheryl succinate, an agent with anticancer activity. Comparison with receptor-mediated pro-apoptotic signaling. Biochemistry 2003;42(14):4277–91.
- Gogvadze V, Norberg E, Orrenius S, Zhivotovsky B. Involvement of Ca2+ and ROS in α-tocopheryl succinate-induced mitochondrial permeabilization. Int J Cancer 2010;127(8):1823–32.
- Dong LF, Low P, Dyason J, Wang XF, Prochazka L, Witting PK, et al. α-Tocopheryl succinate induces apoptosis by targeting ubiquinone-binding sites in mitochondrial respiratory complex II. Oncogene 2008;27(31):4324–35.
- Dong LF, Freeman R, Liu J, Zobalova R, Marin-Hernandez A, Stantic M, et al. Suppression of tumor growth in vivo by the mitocan α-tocopheryl succinate requires respiratory complex II. Clin Cancer Res 2009;15(5):1593–600.
- Prochazka L, Dong LF, Valis K, Freeman R, Ralph SJ, Turanek J, et al. α-Tocopheryl succinate causes mitochondrial permeabilization by preferential formation of Bak channel. Apoptosis 2010;15(7):782–94.
- Valis K, Prochazka L, Boura E, Chladova M, Obsil T, Rohlena J, et al. Hippo/Mst1 stimulates transcription of NOXA in a FoxO1-dependent manner. Cancer Res 2011;71(3):946–54.
- Murphy MP, Smith RA. Targeting antioxidants to mitochondria by conjugation to lipophilic cations. Annu Rev Pharmacol Toxicol 2007;47:629–56.
- Dong LF, Jameson VJA, Tilly D, Cerny J, Mahdavian E, Marín-Hernández A, et al. Mitochondrial targeting of vitamin E succinate enhances its pro-apoptotic and anti-cancer activity via mitochondrial complex II. J Biol Chem 2011;286(5):3717–28.
- Dong LF, Jameson VJA, Tilly D, Prochazka L, Rohlena J, Valis K, et al. Mitochondrial targeting of α-tocopheryl succinate enhances its pro-apoptotic efficacy: a new paradigm of efficient anti-cancer therapy. Free Radic Biol Med 2011;50(11):1546–55.
- Pass HI, Stevens EJ, Oie H, Tsokos MG, Abati AD, Fetsch PA, et al. Characteristics of nine newly derived mesothelioma cell lines. Ann Thorac Surg 1995;59(4):835–44.
- Jackaman C, Bundell CS, Kinnear BF, Smith AM, Filion P, van Hagen D, et al. IL-2 intratumoral immunotherapy enhances CD8+ T cells that mediate destruction of tumor cells and tumor-associated vasculature: a novel mechanism for IL-2. J Immunol 2003;171(10):5051–63.
- Pesta D, Gnaiger E. High-resolution respirometry: OXPHOS protocols for human cells and permeabilized fibers from small biopsies of human muscle. Methods Mol Biol 2012;810:25–58.
- Wang XF, Birringer M, Dong LF, Veprek P, Low P, Swettenham E, et al. A peptide adduct of vitamin E succinate targets breast cancer cells with high erbB2 expression. Cancer Res 2007;67(7):3337–44.
- Leigh J, Driscoll T. Malignant mesothelioma in Australia, 1945–2002. Int J Occup Environ Health 2003;9(3):206–17.
- Neuzil J, Zhao M, Ostermann G, Sticha M, Gellert N, Weber C, et al. α-Tocopheryl succinate, an agent with in vivo anti-tumour activity, induces apoptosis by causing lysosomal instability. Biochem J 2002;362(Pt 3):709–15.
- Qian M, Kralova J, Yu W, Bose HR Jr, Dvorak M, Sanders BG, et al. c-Jun involvement in vitamin E succinate induced apoptosis of reticuloendotheliosis virus transformed avian lymphoid cells. Oncogene 1997;15(2):223–30.
- Yu W, Liao QY, Hantash FM, Sanders BG, Kline K. Activation of extracellular signal regulated kinase and c-Jun-NH2-terminal kinase but not p38 mitogen-activated protein kinases is required for RRR-α-tocopheryl succinate-induced apoptosis of human breast cancer cells. Cancer Res 2001;61(17):6569–76.
- Sun F, Huo X, Zhai Y, Wang A, Xu J, Su D, et al. Crystal structure of mitochondrial respiratory membrane protein complex II. Cell 2005;121(7):1043–57.
- Rodríguez-Enríquez S, Hernández-Esquivel L, Marín-Hernández A, Dong LF, Akporiaye ET, Neuzil J, et al. Molecular mechanism of the selective effect of vitamin E analogues on cancer cell mitochondrial function. Biochim Biophys Acta 2012;1817(9):1597–607.
- Moreno-Sánchez R, Hernández-Esquivel L, Rivero-Segura NA, Marín-Hernández A, Neuzil J, Ralph SJ, et al. Reactive oxygen species are generated by the respiratory complex II: evidence for lack of contribution of the reverse electron flow in complex I. FEBS J 2013;280(3):927–38.
- Quinlan CL, Orr AL, Perevoshchikova IV, Treberg JR, Ackrell BA, Brand MD. Mitochondrial complex II can generate reactive oxygen species at high rates in both the forward and reverse reactions. J Biol Chem 2012;287(32):27255–64.
- dos Santos GA, Abreu e Lima RS, Pestana CR, Lima AS, Scheucher PS, Thomé CH, et al. (+)α-Tocopheryl succinate inhibits the mitochondrial respiratory chain complex I and is as effective as arsenic trioxide or ATRA against acute promyelocytic leukemia in vivo. Leukemia 2012;26(3):451–60.
- Acín-Pérez R, Fernández-Silva P, Peleato ML, Pérez-Martos A, Enriquez JA. Respiratory active mitochondrial supercomplexes. Mol Cell 2008;32(4):529–39.
- Lapuente-Brun E, Moreno-Loshuertos R, Acín-Pérez R, Latorre-Pellicer A, Colás C, Balsa E, et al. Supercomplex assembly determines electron flux in the mitochondrial electron transport chain. Science 2013;340(6140):1567–70.
- Prochazka L, Koudelka S, Dong LF, Stursa J, Goodwin J, Neca J, et al. Mitochondrial targeting overcomes ABCA1-dependent resistance of lung carcinoma to vitamin E succinate. Apoptosis 2013;18(3):286–99.