Abstract
Diabetes is increasing at an alarming rate and, despite anti-hypertensive and insulin therapies, diabetic patients are still at risk of developing complications such as chronic kidney disease, cardiovascular disease, and retinopathy. There is therefore an urgent need for more effective therapies to prevent the development and progression of diabetic complications. Oxidative stress is a major player in the aetiology of diabetic complications. However, results from clinical trials thus far using general antioxidants have been disappointing. Mechanism-based antioxidants have gained considerable attention due to their more targeted approach at reducing oxidative stress and associated complications in diabetes. The transcription factor, NFE2-related factor 2 (Nrf2), is a master regulator of redox homeostasis and the cellular detoxification response. Instead of relying on a single antioxidant, activation of Nrf2 results in the concerted upregulation of several antioxidant enzymes and cytoprotective genes, making it an attractive therapeutic target for diabetic complications. Several Nrf2 activators have been discovered and have proven effective at activating Nrf2 signalling through different mechanisms in both in vitro and in vivo models of diabetes. This review will address some of the most promising and well-known Nrf2 activators and their roles in preventing the development and progression of diabetic complications. Challenges facing the advancement of this drug class into the clinic will be discussed, as will be the future of Nrf2 activation as a therapeutic strategy in preventing the development of diabetic complications.
Introduction
Diabetes is one of the most common chronic diseases worldwide. It is estimated that there are 366 million people with diabetes globally and this figure is expected to rise to 552 million people by 2030.Citation1 Although intensive blood glucose and blood pressure control have reduced the risk of diabetes-associated microvascular (nephropathy, retinopathy, neuropathy) and macrovascular complications (atherosclerosis, peripheral vascular disease, cerebrovascular disease), diabetes remains a major risk factor for end-stage renal disease, blindness, and cardiovascular complications. There is therefore an urgent need to develop more effective therapies to prevent and/or halt the progression of diabetic complications.
Accumulating evidence suggests that oxidative stress plays a pivotal role in the aetiology of diabetic complications.Citation2,Citation3 More than a decade ago, a unifying hypothesis was proposed by which hyperglycaemia together with excessive free fatty acids leads to elevations in reactive oxygen species (ROS) produced by the mitochondrial electron transport chain. These ROS in turn activate stress-sensitive intracellular signalling pathways that then divert glycolytic intermediates into pathways of hyperglycaemic injury, such as the polyol pathway, the advanced glycation endproducts and receptors (AGE/RAGE) pathway, the protein kinase C (PKC) pathway and the hexosamine pathway,Citation2,Citation4 ultimately leading to insulin resistance, β-cell dysfunction and the development of diabetic complications. Recent advances on the pathophysiology of diabetic complications have revealed that the role of ROS in diabetic complications may be more complicated than initially thought. Schaffer et al.Citation5 have recently revisited the unifying hypothesis of diabetes and found that the original theory neglected to acknowledge the potential influence of cytosolic ROS and the contribution by the protective antioxidant defence system.
Indeed, emerging evidence suggests that glucose may also alter antioxidant defences in endothelial cellsCitation6 and in patients with diabetic complications such as diabetic nephropathy.Citation7,Citation8 Fibroblasts derived from type 1 diabetic patients susceptible to microvascular complications were unable to upregulate their protective antioxidant defences after exposure to high glucose compared with skin fibroblasts from normal subjects, suggesting a failure of antioxidant defences in diabetic patients with nephropathy.Citation6 A decline in the levels of non-enzymatic antioxidants such as vitamins C and E and glutathione (GSH), together with a reduction in the activity of enzymatic antioxidants such as superoxide dismutase (SOD), catalase, glutathione peroxidase (GPx), glutathione S-transferase (GST), and glutathione reductase (GR), were also reported in the diabetic rat kidney.Citation9,Citation10 The gene expression of SOD, GPx, and catalase was also found to be significantly reduced in patients with diabetic retinopathy when compared with non-diabetic patients and diabetic patients without retinopathy.Citation11 These findings suggest that increased ROS levels in diabetes are not only the result of their increased production but also a consequence of impaired antioxidant defences.
ROS have been viewed conventionally as the causal factor of many human diseases. However, ROS also have important regulatory roles in a range of biological processes, including cell signalling and survival.Citation12 Thus far, clinical trials of antioxidants (mainly vitamins E and C) have been disappointing largely due to a lack of mechanistic understanding of oxidant and antioxidant actions to design the right interventions.Citation13 More recently, it is thought that mechanism-based antioxidants may be more effective at preventing and/or treating diabetic complications, particularly since it is now recognized that 2-electron oxidants, such as hydrogen peroxide and peroxynitrite, may be more damaging than 1-electron oxidants,Citation14 the latter being the main substrates of antioxidants such as vitamins E and C.Citation15–Citation19 Indeed, this lack of mechanistic understanding may offer an explanation as to why large scale human trials have proven ineffective. One of the most promising classes of mechanism-based antioxidants is the Nrf2 (NFE2-related factor) activators.Citation20 This review will discuss the therapeutic potential of Nrf2 activators in the context of diabetic complications based on available animal and clinical data and some of the issues facing these antioxidants, mainly due to dose-dependent side effects.
The Nrf2/Keap1 pathway
Nrf2 is a member of the cap ’n’ collar subfamily of the bZIP transcription factor and a master regulator of the cellular detoxification response and redox status. Under normal physiological conditions, Nrf2 is constitutively ubiquitinated and degraded by the proteasome through its interaction with its inhibitor, kelch-like ECH-associated protein 1 (Keap1) ().Citation21 Oxidative stress or electrophilic compounds stabilize Nrf2 by antagonizing the interaction of Keap1 with Nrf2, leading to the rapid translocation of Nrf2 to the nucleus where it binds to antioxidant-responsive elements (ARE) within genes. This in turn leads to an increase in the transcription of genes encoding antioxidants and detoxification enzymes such as NAD(P)H:quinine oxidoreductase 1 (NQO1), heme oxygenase-1 (HO-1), γ-glutamylcysteine synthetase (γ-GCS), and GST. One of the most attractive features of targeting the Nrf2/Keap1 pathway is that instead of relying on a single antioxidant enzyme, Nrf2 activation leads to a concerted upregulation of a range of antioxidant enzymes. It is therefore not surprising that attention has focussed on identifying a number of small molecule activators of Nrf2 to fulfil the unmet clinical need of reducing oxidative stress in diseases such as diabetic complications.
Figure 1. The role of Nrf2 signalling in diabetic complications. Hyperglycaemia is associated with the generation of reactive oxygen species (ROS) by the mitochondria and angiotensin II (Ang II)-induced activation of NOX. Ang II and ROS can activate the NFκB pathway leading to transcription of fibrotic and inflammatory genes such as TGF-β, MCP1 and TNF-α, resulting in diabetic complications. Under normal conditions, Nrf2 is constitutively targeted by its negative regulator, Keap1, for ubiquitination and degradation in the cytosol. On the other hand, hyperglycaemia-induced oxidative stress stabilizes Nrf2 by releasing Keap1 binding, allowing Nrf2 to translocate to the nucleus where it activates ARE-responsive genes, such as HO-1, NQO1, SOD, and CAT. These antioxidant and cytoprotective genes reduce ROS levels, leading to an attenuation of diabetic complications.
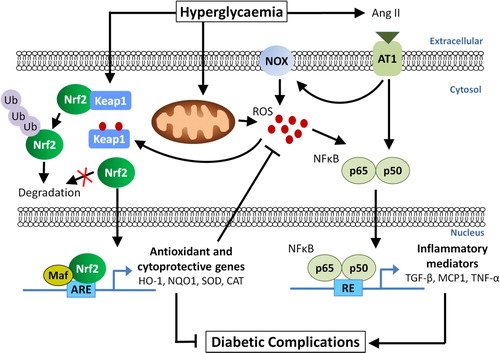
Diabetes is known to induce oxidative stress either through overproduction of superoxide by the mitochondrial electron transport chain,Citation2,Citation4 or activation of angiotensin II-mediated upregulation of nicotinamide adenine dinucleotide phosphate (NADPH)-oxidase (NOX) activity resulting in cytosolic production of ROS (). In agreement with the notion that oxidative stress is elevated in diabetes, the protective Nrf2 pathway is activated under hyperglycaemic conditions in both in vitroCitation22–Citation25 and in vivo experimental models of diabetes.Citation23,Citation26,Citation27 Furthermore, Nrf2 expression and its downstream gene, NQO1, were significantly upregulated in the glomeruli of diabetic nephropathy patients when compared with patients without diabetic nephropathy.Citation23 However, a different finding was observed in human diabetic failing hearts where Nrf2 expression was significantly reduced when compared with normal hearts.Citation28 Interestingly, in patients with diabetic retinopathy, retinal expression of Nrf2 was increased when compared with retina from non-diabetic donors.Citation27 However, retinal expression of the Nrf2 downstream gene, catalytic subunit of glutamylcysteine ligase, was significantly reduced, which is suggestive of defective Nrf2 signalling in diabetic retinopathy.Citation27
Other animal studies have shown that the Nrf2 system is compromised in diabetes, which may contribute to complications such as impaired wound healingCitation29 and diabetic nephropathy.Citation9 More importantly, cardiac expression of Nrf2 was slightly increased 2–3 months after the onset of diabetes in streptozotocin (STZ)-induced diabetic mice, but significantly decreased after 5–6 months of diabetes.Citation28,Citation30 This initial upregulation of Nrf2 suggests that Nrf2 is responding to the early increase in oxidative stress to overcome injury at the early stages of diabetes. At the later stages, however, this adaptive process fails and cardiac antioxidant function is impaired. These findings led the authors to speculate that without this initial adaptive response by Nrf2, the cardiac damage induced by diabetes would have been more prominent.Citation30 Several other studies have shown similar findings in the aorta,Citation31 kidney,Citation32 and heartCitation30 of diabetic mice, where Nrf2 and its downstream regulatory proteins (HO-1, NQO1, and SOD1) were significantly increased after 3 months of diabetes, but significantly reduced at 6 months. Collectively, these findings suggest that the Nrf2 system plays an important role as the body's natural defence against hyperglycaemia-induced damage. However, this initial adaptive response to counteract the diabetes-driven oxidative stress appears to be short-lived, after which the Nrf2 system becomes overwhelmed under chronic glucose stimulation. Thus, activating the Nrf2 signalling pathway with small molecule Nrf2 activators may bolster endogenous antioxidant systems to prevent the development or progression of diabetic complications.
Lessons from Nrf2 knockout studies
Nrf2 knockout (Nrf2−/−) mice show a deficiency in Nrf2-mediated gene responses and display a higher susceptibility to oxidative injury and diabetes.Citation33,Citation34 The role of Nrf2 in diabetic nephropathy was first investigated in a study by Yoh et al.Citation35 In their study, STZ-treated Nrf2−/− mice developed renal impairment earlier than wild-type (WT) mice, with a significant reduction in creatinine clearance rate as early as 6 weeks (compared with 10 weeks for WT mice) after the onset of diabetes and without the hyperfiltration observed in the WT mice.Citation35 Furthermore, urinary excretion of nitric oxide metabolites and the occurrence of 8-nitroguanosine (a biomarker for nitrosative stress) in glomerular lesions were exacerbated in Nrf2−/− mice after STZ treatment. However, in the same study, urinary protein output and renal pathology were not significantly different between knockout and WT mice after 10 weeks of STZ treatment.Citation35
In a longer study of 16 weeks duration, STZ-induced diabetic Nrf2−/− mice exhibited more severe glomerular injury, together with higher ROS production and increased expression of the profibrotic cytokine, transforming growth factor-β (TGF-β), as well as increases in the profibrotic marker fibronectin, when compared with diabetic WT mice.Citation23 These changes were accompanied by a significantly higher urinary albumin to creatinine ratio (UACR), strongly suggesting that Nrf2 plays a protective role in STZ-induced diabetic nephropathy.Citation23 In human renal mesangial cells (HRMCs), high glucose induced an increase in ROS production with concomitant elevations in the expression of Nrf2 and its downstream genes including NQO1, HO-1, and γ-GCS and these responses were diminished when expression of Nrf2 was reduced by siRNA.Citation23 Furthermore, induction of endogenous Nrf2 with tert-butylhydroquinone inhibited the promoter activity of TGF-β1 in a dose-dependent manner, and knockdown of Nrf2 exacerbated high glucose-induced TGF-β and fibronectin expression in HRMCs.Citation23
Ungvari et al.Citation24,Citation36 were the first to characterize the vasoprotective role of Nrf2 in diabetes using Nrf2−/− mice. They showed that expression of Nrf2 downstream genes was significantly upregulated in diabetic Nrf2+/+ mice, but not in diabetic Nrf2−/− mice, when compared with appropriate non-diabetic mice in an experimental model of type 2 diabetes (T2D). Additionally, high fat diet (HFD)-induced increases in vascular ROS levels were significantly greater in Nrf2−/− than Nrf2+/+ mice, accompanied by a more severe endothelial dysfunction as shown by the significantly diminished acetylcholine (ACh)-induced relaxation of aorta in these mice, which was accompanied by increased inflammatory gene expression, such as intercellular adhesion molecule-1 and tumour necrosis factor-α (TNF-α).Citation24
Neonatal and adult cardiomyocytes isolated from Nrf2−/− mice exhibited higher ROS under basal conditions compared with WT controls and high glucose further markedly increased ROS production, accompanied by an increase in cardiomyocyte apoptosis.Citation22 Furthermore, adult cardiomyocytes from diabetic Nrf2−/− mice exhibited dramatically increased ROS levels and severely damaged contractility compared with WT controls, demonstrating a critical in vivo role for Nrf2 in the defence against high glucose-mediated damage of cardiomyocytes.Citation22 More recently, it was found that diabetes further exacerbated oxidative damage to DNA as measured by an increase in 8-OHdG in the diabetic Nrf2−/− heart.Citation37
Nrf2 activators
To date, several small molecule activators of Nrf2 have been identified. The most well known is sulforaphane, derived from cruciferous vegetables such as broccoli. Other natural Nrf2 activators that are widely used in complementary medicine include cinnamic aldehyde (main constituent of cassia and cinnamon bark oils), resveratrol (found in the skin of grapes), curcumin (from turmeric), and quercetin (from tea, berries, apples, and onions).Citation38 Less well known Nrf2 activators include MG132, the specific, potent, reversible, and cell-permeable proteasome inhibitor,Citation39 and ebselen, the synthetic mimetic of the antioxidant enzyme, glutathione peroxidase-1 (GPx).Citation40 Our group previously has shown that ebselen reduced oxidative stress in animal models of T1D and attenuated diabetes-associated atherosclerosis and diabetic nephropathy.Citation41,Citation42 In addition, some of the most promising Nrf2 activators are a series of triterpenoids derived from oleanic acid. The first to be described was 2-cyano-3,12-dioxooleana-1,9,-dien-28-oic acid (CDDO), and since then other synthetic triterpenoids have been developed.Citation43,Citation44 This review will focus on Nrf2 activators that have shown therapeutic benefits in animal models of diabetic complications and have the most potential for translation into the clinic.
Sulforaphane
Sulforaphane acts by releasing Nrf2 from Keap1 through the modification of critical cysteine thiol residues on Keap1, thereby allowing Nrf2 to translocate to the nucleus and activate ARE-responsive genes ().Citation45 An early study by Xue et al.Citation25 investigated the molecular mechanisms behind the protection afforded by sulfurophane in preventing high glucose-induced damage in human microvascular HMEC-1 endothelial cells. They not only showed that sulforaphane increased the translocation of Nrf2 to the nucleus and increased ARE-responsive gene expression, but that it also attenuated hyperglycaemia-mediated activation of the hexosamine and PKC pathways as well as lowered ROS and methylglyoxal levels.Citation25 Of note, the authors discussed the potential cytotoxic side effects of sulforaphane and related isothiocyanates to endothelial cells and other cell types at higher concentrations, possibly through their interaction with death receptors and apoptotic signalling pathways, independent of the disruption of the Keap1–Nrf2 complex.Citation25
Figure 2. The proposed mechanisms of Nrf2 activators. Bardoxolone methyl, dh404, and sulforaphane interact with cysteine thiol residues on Keap1, the negative regulator of Nrf2. This interaction leads to the dissociation and stabilization of Nrf2, allowing it to translocate to the nucleus and bind to the antioxidant response element (ARE) in the promoter region of antioxidant and cytoprotective genes, leading to the transcription of these genes. On the other hand, MG132 inhibits proteasomal activity thereby preventing the degradation of Nrf2 leading to the increased accumulation of Nrf2 in the nucleus and activation of ARE-responsive genes. The mechanism of resveratrol is less well understood but it is proposed to increase expression and translocation of Nrf2 to the nucleus, possibly by decreasing the expression of Keap1.
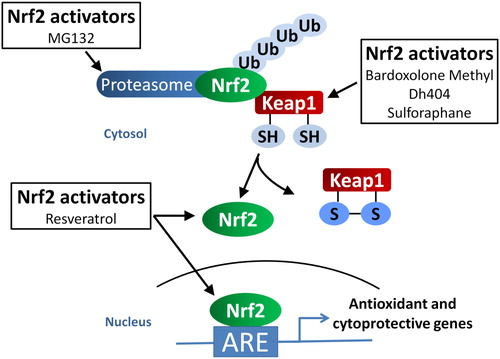
In HRMCs, sulforaphane has been shown to prevent TGF-β-mediated signalling and hypertrophy under hyperglycaemic conditions.Citation26 Furthermore, siRNA silencing of Nrf2 reversed the sulforaphane and/or high glucose-induced Nrf2 and HO-1 expression in human kidney proximal tubular (HK 11)Citation32 and cardiac H9c2 cells.Citation30 In addition, silencing of Nrf2 reversed sulforaphane-mediated protection against high-glucose-induced connective tissue growth factor expression.Citation32 These studies strongly suggest the requirement of functional Nrf2 for sulforaphane-mediated protection against high glucose-induced renal and cardiac fibrotic injury.
In preclinical studies in mice, pretreatment with sulforaphane (at 40 µg/kg for 3 days) prevented STZ-mediated islet destruction and restored insulin-secreting islet cells to levels similar to control mice, possibly through suppression of the redox-sensitive NFκB pathway.Citation46 More recently, sulforaphane (125 mg/kg) and cinnamic aldehyde (25 and 50 mg/kg) have been shown to improve kidney function, alleviate the pathological alterations within the glomerulus and reduce the upregulation of TGF-β and its downstream effectors, fibronectin, collagen IV, and p21 in the kidneys of diabetic mice.Citation26 Importantly, these effects of sulforaphane and cinnamic aldehyde were not seen in Nrf2−/− mice, thus providing further evidence that sulforaphane and cinnamic aldehyde function specifically through activation of the Nrf2 pathway ().
Furthermore, 0.5 mg/kg of sulforaphane given subcutaneously for 3 months was effective in preventing diabetes-induced aortic fibrosis and remodelling in STZ-treated mice, which was associated with an upregulation of Nrf2 expression and its downstream genes NQO1 and HO-1.Citation31 Of interest, this aortic protection by sulforaphane was not only observed at the end of the 3-month period, but also 3 months after terminating the treatment, indicative of epigenetic modifications as a potential mechanism of sulforaphane.Citation31 In addition, the cardioprotective effects of sulforaphane were examined in STZ-induced diabetic mice with the same treatment regimen. In this instance, 0.5 mg/kg sulforaphane was found to attenuate cardiac dysfunction and remodelling after 3 months of treatment, which additionally persisted 3 months after treatment.Citation30 Even more intriguing was the observation that the cardioprotective effect of sulforaphane was more evident 3 months after treatment. However, this sustained effect was not seen in the kidney of STZ-induced diabetic mice with the same treatment regimen since the renoprotective effects of sulforaphane were lost 3 months after termination of the treatment.Citation32 These data suggest that treatment with sulfophane may elicit differential responses in various tissues.
Translation of sulforaphane to the clinic has been in the form of broccoli sprout powder which contains sulforaphane and the administration to patients with T2D.Citation47,Citation48 In these studies, 5 or 10 g of broccoli sprout powder was given to T2D patients. Equivalent doses of corn starch containing chlorophyll as placebo were administered to a separate group of patients for 4 weeks. At each dose, 5 and 10 g broccoli sprout powder translated into 112 and 225 mmol of sulforaphane isothiocyanates, respectively. Administration of broccoli powder at 10 g daily for 4 weeks decreased plasma malondialdehyde and oxidized low-density lipoprotein (oxLDL), and significantly increased the total antioxidant capacity.Citation49 However, there was no change in the total oxidant status. Cardiovascular risk factors such as serum triglycerides (TG), oxLDL/LDL-C ratio and atherogenic index of plasma (log of TG/HDL-C ratio) were also significantly reduced in patients administered 10 g of broccoli sprout powder when compared with placebo and those receiving 5 g of powder.Citation47 Furthermore, inflammatory markers which included high-sensitive C reactive protein and interleukin-6 (IL-6) were significantly reduced in the 10 g group of patients.Citation48 Of note, only minor adverse events were reported in these studies.Citation47 However, limitations of the study include the short treatment period and limited doses and parameters examined. The effects of sulforaphane in diabetic patients and their potential to limit diabetic complications thus require further studies with longer duration, various doses and more clinically relevant cardiovascular or renal outcomes.
Resveratrol
Resveratrol is a polyphenol that protects plants against fungal infection. It is found in the skin of grapes, red wine, berries, and many other plants. Resveratrol exerts its antioxidant properties through activation of Nrf2 signalling,Citation36 although the exact mechanism is still unclear; downregulation of Keap1 expressionCitation50 and activation of the protein deacetylase, Sirtuin 1 (Sirt 1) leading to increased nuclear accumulation of Nrf251 are two proposed mechanisms ().
In cultured human coronary arterial endothelial cells (CAECs), resveratrol significantly increased the transcriptional activity of Nrf2 in a dose-dependent manner, with subsequent upregulation of Nrf2 genes such as HO-1, NQO1, and γ-GCS.Citation24,Citation36 Resveratrol treatment also significantly attenuated high glucose-induced mitochondrial and cellular oxidative stress and apoptosis in CAECS. These effects were prevented by downregulation of Nrf2 expression either by siRNA or overexpression of Keap1.Citation36 In HFD-fed Nrf2+/+ mice, resveratrol treatment attenuated oxidative stress (assessed by the Amplex red assay), improved ACh-induced vasodilatation, and inhibited apoptosis (assessed by measuring caspase-3 activity and DNA fragmentation) in branches of the femoral artery.Citation36 In contrast, the aforementioned endothelial protective effects of resveratrol were diminished in HFD-fed Nrf2−/− mice, further reinforcing the notion that resveratrol exerts its vasoprotective effects, at least partly, through Nrf2-dependent pathways.Citation36
In preclinical studies, in a model of type 1 diabetes (T1D) using STZ-nicotinamide-induced diabetic rats, resveratrol (5 mg/kg) given for 30 days, significantly attenuated oxidative stress (superoxide, OH, NO, lipid peroxide, hydroperoxide, protein carbonyl) in the diabetic rat kidney and restored the activities of antioxidants such as catalase, GPx, SOD, GST, and GR, as well as that of non-enzymatic antioxidants such as vitamins C, E and GSH.Citation9 Additionally, Nrf2 and its downstream target proteins, HO-1 and γ-GCS, were reduced in the diabetic rat kidney with concomitant elevations in Keap1, and all of these changes were normalized by resveratrol. Importantly, resveratrol also attenuated glomerular, tubular, and interstitial injury in these diabetic rats, with an improvement in kidney function as assessed by an increase in creatinine clearance.Citation9 Although higher doses of resveratrol (20 mg/kg/day for 8 weeks) have been reported to attenuate albuminuria and glomerular mesangial expansion in the STZ-induced diabetic rat kidney, the mechanism behind these improvements may differ since the diabetes-induced upregulation of the Nrf2 downstream gene, GST Mu (GSTM), was attenuated.Citation52 This effect of resveratrol was dose dependent as demonstrated in rat mesangial cells treated with high glucose, and higher doses of resveratrol also were associated with higher rates of apoptosis in these cells.Citation52
To date, there are only limited data available on the pharmacological activities of resveratrol in humans. Supplementary resveratrol at relatively low doses has been shown to be well tolerated in healthy subjects and was able to suppress high-fat, high-cholesterol meal-induced elevations of plasma endotoxins and the expression of pro-oxidants and inflammatory markers such as p47phox, Keap1, IL-1β, and TNF-α with concomitant elevations of Nrf2-binding activity and the expression of NQO1 and GST.Citation53 In T2D patients, insulin sensitivity was improved after 4 weeks of resveratrol treatment (given twice daily in gelatine capsule containing 5 mg resveratrol), possibly due to resveratrol-induced decreases in oxidative stress that leads to more efficient insulin signalling via the Akt pathway.Citation54 However, the effects of resveratrol in diabetic complications have not been reported thus far in humans.
Beside Nrf2 signalling, resveratrol has been known to exert its effect through modulations of other signal transduction pathways such as the cAMP/cGMP pathway, the MAP kinase signalling PI3K/AKT pathway and cell cycle and cell death signalling (reviewed by Pervaiz and HolmeCitation55). Furthermore, resveratrol is also involved in autophagy and preconditioning, anti-fibrotic and anti-inflammatory effects, and activation of estrogen receptors having similar chemical properties to estrogen in the diabetic heart (reviewed by Turan et al.Citation56). Although many pre-clinical studies have shown the benefits of resveratrol in combating diabetic complications as discussed earlier, resveratrol is also being investigated as a potential anti-cancer drug due to its pro-oxidant activity leading to oxidative breakage of cellular DNA.Citation57,Citation58 The fine balance between the anti-oxidant and cytotoxic properties of resveratrol is largely depended on the concentration, the redox environment of the cell and the presence of transition metals such as copper.Citation59 Based on the published data thus far, there is not enough evidence to strongly justify a recommendation for the administration of resveratrol to humans beyond the dose which can be obtained from dietary sources.Citation60 However, encouraging data from animal models of diabetic complications using resveratrol warrant further investigations with the potential for translation to clinical settings.
MG132
Under physiological conditions, Nrf2 is targeted for ubiquitination and subsequent proteasomal degradation via a mechanism that includes redox-sensitive Keap1 in the cytosol (). Diabetes is associated with an increase in proteasomal activity.Citation61–Citation63 Importantly, increased proteasomal activity is linked to a reduction in the expression and activation of Nrf2 in diabetes.Citation10,Citation64 Thus, it has been hypothesized that proteasomal inhibition will be beneficial in preventing and/or treating diabetic complications by preventing the degradation of Nrf2, thereby leading to increased Nrf2 accumulation in the nucleus and subsequent activation of Nrf2 target gene transcription. Several animal studies have shown that MG132, a cell-permeable proteasome inhibitorCitation39, attenuates diabetes-associated renalCitation10,Citation65, cardiac,Citation64 and aortic diseasesCitation66 through the upregulation of Nrf2 expression, as well as transcription of downstream genes. Indeed, MG132-mediated upregulation of antioxidant enzymes and the protection against H2O2-mediated oxidative stress is completely lost in neonatal cardiomyocytes isolated from Nrf2−/− mice.Citation39 Furthermore, silencing of Nrf2 abolishes MG132-mediated protection against high glucose-induced profibrotic responses in HK 11 cells.Citation65 These findings provide further proof that MG132 mediates its beneficial effects in diabetic complications, at least partly, through the activation of Nrf2 signalling.
Furthermore, NFκB, a nuclear transcription factor that regulates proinflammatory cytokine expression, is usually inhibited by IκB via formation of a complex with NFκB. However, IκB is degraded by proteasome ubiquitination resulting in the release of NFκB and nuclear translocation to mediate the inflammatory response. In the diabetic heart, inhibition of proteasome activity by MG132 also inhibited the degradation of IκB, thus allowing IκB to bind to NFκB, thereby inhibiting its transcriptional activity and reducing the inflammatory response.Citation64
However, one concern for the use of MG132 in preventing and/or treating diabetic complications is related to the hormetic effect often associated with antioxidants. The partial inhibition of proteasomal activity by MG132 at low doses may be therapeutically beneficial in diabetic complications; however, at higher doses it could exacerbate oxidative stress in the cellCitation67 and thereby induce apoptotic cell death.Citation65
Bardoxolone methyl and its analogues
Bardoxolone methyl (methyl 2-cyno-3,12-dioxooleana1,9(11)dien-28-oate, CDDO-Me, or RTA 402) is a synthetic triterpenoid derived from the natural product oleanolic acid and is a member of a class of antioxidant inflammation modulators (AIMs) that are the most potent activators of Nrf2 available.Citation68,Citation69 CDDO-Me interacts with specific cysteine residues on Keap1, allowing Nrf2 to accumulate and translocate to the nucleus leading to upregulation of ARE-responsive genes ().Citation68 There are limited published preclinical studies on CDDO-Me due to rodent-specific metabolism of CDDO-Me to toxic metabolites, making studies in rodents requiring longer-term progression, like diabetic complications, difficult. However, the emergence of closely related analogues of CDDO-Me without such limitations such as CDDO-ethyl amide (RTA-450) and dihydro-CDDO-trifluoroethyl amide (dh404) has opened up new opportunities to further explore AIMs in preclinical models of diabetes. Similar to its parent compound, dh404 interacts with specific cysteine residues on Keap1, allowing Nrf2 to translocate to the nucleus to activate Nrf2-driven gene transcription.Citation70
Indeed, treatment of STZ-induced diabetic mice with dh404 for 2 weeks at 10 mg/kg every alternate day significantly increased the expression of cardiac Nrf2 expression and prevented 3-nitrotyrosine (NT) accumulation (a marker for peroxynitrite-induced tissue damage) and ERK1/2 phosphorylation, and preserved normal insulin signalling in the diabetic heart.Citation28 However, in another study, Zoja et al. found that treatment with RTA 405 resulted in significant weight loss, reduced food intake, increases in blood pressure and dyslipidaemia in an animal model of T2D, the Zucker diabetic fatty (ZDF) rats.Citation71 Treatment of ZDF rats with RTA 405 for 3 months at 50 and 100 mg/kg/day also resulted in a worsening of proteinuria, glomerulosclerosis and tubular damage, with early elevations in the levels of alanine aminotransferase (ALT) and aspartate aminotransferase, followed by morphologically visible liver damage. As the formation of toxic metabolites is a known problem in rodents given CDDO-Me, it was suspected that these detrimental effects of RTA 405 could be due to toxic impurities of the compound. Thus, these studies were repeated with dh404 with the added advantage that the fluorine substituent on dh404 provides protection against the possible formation of rodent-specific toxic metabolites.Citation71 Similar to RTA 405, dh404 treatment was associated with body weight reduction at 25 mg/kg/day but not at 5 mg/kg/day. Unlike RTA 405, dh404 had no effects on blood pressure or lipids. After 3 months of treatment the authors observed no beneficial effects on renal disease of ZDF rats by dh404, but rather a trend towards increased proteinuria, glomerulosclerosis, tubular casts, and interstitial inflammation especially at the higher dose; although these changes were not significantly different to ZDF rats treated with vehicle alone.Citation71 Lesions reminiscent of a renal cell pseudotumour also were observed in three out of 20 rats (15%) treated with dh404.
Contrary to the findings of Zoja et al.,Citation71 treatment with RTA 405 or dh404 for 6 weeks was not associated with any adverse impact on survival, body weight, or liver enzymes and morphology in ZDF rats at 3, 10, 20, or 50 mg/kg/day in a subsequent study.Citation72 RTA 405 and dh404 did not exacerbate kidney pathology beyond that typically observed in ZDF rats. RTA 405 also was well tolerated, with no adverse findings in two additional animal models, the STZ-induced diabetic rat and HFD-induced obese mice.Citation72 Importantly, RTA 405 improved renal function and glucose control and reduced adiposity in these animals. The reasons for the discrepancy between the two studies remain unclear, however several factors that may have contributed include drug product impurities or degradation products that differed between the two studies, the duration of treatments, inherent differences in the ZDF rat colonies used and the appropriateness of the ZDF rat for renal studies.Citation72 These studies further emphasize the importance of preclinical testing in animals for drug efficacy and safety before translation to humans.
Of note, in the study of Zoja et al., dh404 at 5 mg/kg, but not 25 mg/kg, increased the estimated glomerular filtration rate (eGFR) in ZDF rats,Citation71 as seen in patients who received CDDO-Me.Citation73 While CDDO-Me and its analogues may improve renal filtration through the amelioration of inflammation-induced fibrosis in the glomeruli as initially proposed, it is possible that they also may have a hemodynamic effect by causing afferent arteriolar dilatation and increased intraglomerular pressure as recently proposed.Citation74,Citation75 This hemodynamic effect may result in short-term improvements yet long-term accelerated decline in the GFR. However, a recent study by Ding et al. concluded that RTA 405 and CDDO-Me most likely increase GFR by inhibiting angiotensin II-induced mesangial cell contraction, thereby increasing the glomerular capillary surface area for filtration.Citation76 They also demonstrated that RTA 405 is unlikely to exert its effects via hemodynamic changes as no significant change in blood pressure or renal plasma flow was observed in rats given RTA 405 by oral gavage at a dose of 100 mg/kg body weight for three consecutive days.Citation76 Results from this latter study may alleviate some of the concerns related to the hemodynamic effects of these compounds; however, the jury is still out regarding their use in the treatment of diabetic nephropathy. In particular, any adverse dose-related events need careful evaluation, and it needs to be determined at what stage of disease progression the compounds are most effective with minimal side effects.Citation77 These questions need to be answered with rigorous preclinical testing and appropriate testing in humans to ensure safety and efficacy.
CDDO-Me first entered clinical trials as an anticancer agent, however, in addition to the observed antitumour activity, significant improvements were noted in the eGFR suggesting that CDDO-Me might be beneficial in chronic kidney disease (CKD).Citation78 Indeed, CDDO-Me showed promise in initial trials in patients with T2D and CKD. In the first of two phase 2 clinical trials, this compound showed a dose-dependent increase in eGFR, initially after 56 days of treatment.Citation79 In the second phase 2 clinical trial (BEAM trial),Citation73 227 adults with T2D and CKD were given CDDO-Me at a target dose of 25, 75, and 150 mg once daily.Citation80 Although the primary outcome of the trial was as predicted, with significant increases in the mean eGFR at 24 and 56 weeks, several minor adverse events were reported in patients treated with CDDO-Me. The most common adverse event was muscle spasm, while others included hypomagnesaemia and increases in ALT and gastrointestinal effects. Other concerns included the rate of compliance with the assigned dose, particularly at higher doses, which presumably was due to the increased incidence of these adverse events, such that many patients did not reach their target dose. The therapeutic potential and problems facing CDDO-Me in the clinical setting is further discussed by McCullough and Ali.Citation74 In addition, the much anticipated phase 3 BEACON study with a larger cohort of patients with stage 4 CKD and T2D was terminated prematurely due to safety concerns as a consequence of adverse cardiovascular events and mortality in the CDDO-Me arm. Importantly, a recent study investigating the outcome of the BEACON trial,Citation81 has shown that these events are linked to fluid overload in patients at higher risk of cardiovascular events. Identification of at risk patient populations would be an important first step for future studies with this drug class.
Concluding remarks
The recently terminated phase 3 clinical trial using the Nrf2 activator CDDO-Me in T2D patients with stage 4 CKD has highlighted the need for further in-depth analysis of the regulation of Nrf2 in human diseases. A better understanding of both the protective and deleterious effects of Nrf2 activation is equally important for its successful translation into clinically relevant therapies. The pre-clinical evidence so far seems to point to a window within which these activators offer effective protection. Indeed, too much activation of Nrf2 signalling appears to be detrimental. For instance, Keap1−/− mice mostly die within 3 weeks after birth, indicating that constitutive activation of Nrf2 signalling results in serious adverse effects.Citation82 Furthermore, disruption in Nrf2/Keap1 signalling has been associated with carcinogenesis and a further worrying aspect is the development of resistance by cancer cells to chemotherapy, which has been attributed to increased Nrf2 activation.Citation83
In the diabetes context, the overexpression of Nrf2 as a result of genetic knockdown of Keap1, has been shown to aggravate insulin resistance, impair lipid accumulation in adipose tissue and increase hepatic steatosis in a mouse model of obesity.Citation84 Indeed, ROS are known to promote the conversion of preadipocytes to mature adipocytes and to facilitate insulin action. These ROS-mediated physiological signalling pathways could be adversely affected by enhanced Nrf2 activation, resulting in reduced adipogenesis and insulin resistance.Citation85 Furthermore, if exposed to increased oxidative stress, beta cells adapt by activating cellular adaptive responses such as the Nrf2 system, thereby keeping oxidative damage/cell death-related impairment of glucose-stimulated insulin secretion (GSIS) at a minimum. However, persistent induction of endogenous antioxidants (for example, with Nrf2 activators) could attenuate glucose-dependent ROS signalling leading to reduced GSIS.Citation86 Based on these findings, it is clear that additional studies are required to further elucidate the role of Nrf2 activators in the prevention and/or treatment of diabetes and its associated complications.
In addition, clinical findings of the use of antioxidants showing increased incidence of all-cause death is especially worrying.Citation87 In light of this, questions facing researchers should not only focus on which antioxidants are best, but also how much of a good thing can actually be bad, not only in the context of diabetes and its complications but also in relation to other diseases. As outlined in this review, several Nrf2 activators that showed promising results in preventing the development of diabetic complications in animal studies, presented with potential side effects which appeared to be dose dependent but, as revealed by the in-depth analysis of the BEACON trial, may also depend on the patient cohorts themselves. Thus, future preclinical and clinical studies on the therapeutic potential of Nrf2 activators should focus on dosing and patient cohorts as much as on the mechanism of action of these agents.
References
- Whiting DR, Guariguata L, Weil C, Shaw J. IDF diabetes atlas: global estimates of the prevalence of diabetes for 2011 and 2030. Diabetes Res Clin Pract 2011;94(3):311–21.
- Brownlee M. Biochemistry and molecular cell biology of diabetic complications. Nature 2001;414(6865):813–20.
- Evans JL, Goldfine ID, Maddux BA, Grodsky GM. Oxidative stress and stress-activated signaling pathways: a unifying hypothesis of type 2 diabetes. Endocr Rev 2002;23(5):599–622.
- Giacco F, Brownlee M. Oxidative stress and diabetic complications. Circ Res 2010;107(9):1058–70.
- Schaffer SW, Jong CJ, Mozaffari M. Role of oxidative stress in diabetes-mediated vascular dysfunction: unifying hypothesis of diabetes revisited. Vascul Pharmacol 2012;57(5–6):139–49.
- Ceriello A, Dello Russo P, Amstad P, Cerutti P. High glucose induces antioxidant enzymes in human endothelial cells in culture; Evidence linking hyperglycemia and oxidative stress. Diabetes 1996;45(4):471–7.
- Ceriello A, Morocutti A, Mercuri F, Quagliaro L, Moro M, Damante G, et al. Defective intracellular antioxidant enzyme production in type 1 diabetic patients with nephropathy. Diabetes 2000;49(12):2170–7.
- Hodgkinson AD, Bartlett T, Oates PJ, Millward BA, Demaine AG. The response of antioxidant genes to hyperglycemia is abnormal in patients with type 1 diabetes and diabetic nephropathy. Diabetes 2003;52(3):846–51.
- Palsamy P, Subramanian S. Resveratrol protects diabetic kidney by attenuating hyperglycemia-mediated oxidative stress and renal inflammatory cytokines via Nrf2-Keap1 signaling. Biochim Biophys Acta 2011;1812(7):719–31.
- Luo ZF, Qi W, Feng B, Mu J, Zeng W, Guo YH, et al. Prevention of diabetic nephropathy in rats through enhanced renal antioxidative capacity by inhibition of the proteasome. Life Sci 2011;88(11–12):512–20.
- El-Bab MF, Zaki NS, Mojaddidi MA, Al-Barry M, El-Beshbishy HA. Diabetic retinopathy is associated with oxidative stress and mitigation of gene expression of antioxidant enzymes. Int J Gen Med 2013;19(6):799–806.
- Ray PD, Huang B-W, Tsuji Y. Reactive oxygen species (ROS) homeostasis and redox regulation in cellular signaling. Cell Signal 2012;24(5):981–90.
- Winterbourn CC. Reconciling the chemistry and biology of reactive oxygen species. Nat Chem Biol 2008;4(5):278–86.
- Terentis AC, Thomas SR, Burr JA, Liebler DC, Stocker R. Vitamin E oxidation in human atherosclerotic lesions. Circ Res 2002;90(3):333–9.
- Song Y, Cook NR, Albert CM, Van Denburgh M, Manson JE. Effects of vitamins C and E and β-carotene on the risk of type 2 diabetes in women at high risk of cardiovascular disease: a randomized controlled trial. Am J Clin Nutr 2009;90(2):429–37.
- Mayer-Davis EJ, Monaco JH, Marshall JA, Rushing J, Juhaeri MS. Vitamin C intake and cardiovascular disease risk factors in persons with non-insulin-dependent diabetes mellitus. Prev Med 1997;26(3):277–83.
- Tessier DM, Khalil A, Trottier L, Fülöp T. Effects of vitamin C supplementation on antioxidants and lipid peroxidation markers in elderly subjects with type 2 diabetes. Arch Gerontol Geriatr 2009;48(1):67–72.
- Yusuf S, Dagenais G, Pogue J, Bosch J, Sleight P. Vitamin E supplementation and cardiovascular events in high-risk patients. The Heart Outcomes Prevention Evaluation Study Investigators. N Engl J Med 2000;342(3):154–60.
- Lonn E, Yusuf S, Hoogwerf B, Pogue J, Yi Q, Zinman B, et al. Effects of vitamin E on cardiovascular and microvascular outcomes in high-risk patients with diabetes: results of the HOPE study and MICRO-HOPE Substudy. Diabetes Care 2002;25(11):1919–27.
- de Haan JB. Nrf2 activators as attractive therapeutics for diabetic nephropathy. Diabetes 2011;60(11):2683–4.
- McMahon M, Itoh K, Yamamoto M, Hayes JD. Keap1-dependent proteasomal degradation of transcription factor Nrf2 contributes to the negative regulation of antioxidant response element-driven gene expression. J Biol Chem 2003;278(24):21592–600.
- He X, Kan H, Cai L, Ma Q. Nrf2 is critical in defense against high glucose-induced oxidative damage in cardiomyocytes. J Mol Cell Cardiol 2009;46(1):47–58.
- Jiang T, Huang Z, Lin Y, Zhang Z, Fang D, Zhang DD. The protective role of Nrf2 in streptozotocin-induced diabetic nephropathy. Diabetes 2010;59(4):850–60.
- Ungvari Z, Bailey-Downs L, Gautam T, Jimenez R, Losonczy G, Zhang C, et al. Adaptive induction of NF-E2-related factor-2-driven antioxidant genes in endothelial cells in response to hyperglycemia. Am J Physiol Heart Circ Physiol 2011;300(4):H1133–40.
- Xue M, Qian Q, Adaikalakoteswari A, Rabbani N, Babaei-Jadidi R, Thornalley PJ. Activation of NF-E2–Related Factor-2 reverses biochemical dysfunction of endothelial cells induced by hyperglycemia linked to vascular disease. Diabetes 2008;57(10):2809–17.
- Zheng H, Whitman SA, Wu W, Wondrak GT, Wong PK, Fang D, et al. Therapeutic potential of Nrf2 activators in streptozotocin-induced diabetic nephropathy. Diabetes 2011;60(11):3055–66.
- Zhong Q, Mishra M, Kowluru RA. Transcription factor Nrf2-mediated antioxidant defense system in the development of diabetic retinopathy. Invest Ophthalmol Vis Sci 2013;54(6):3941–8.
- Tan Y, Ichikawa T, Li J, Si Q, Yang H, Chen X, et al. Diabetic downregulation of Nrf2 activity via ERK contributes to oxidative stress-induced insulin resistance in cardiac cells in vitro and in vivo. Diabetes 2011;60(2):625–33.
- Bitar MS, Al-Mulla F. A defect in Nrf2 signaling constitutes a mechanism for cellular stress hypersensitivity in a genetic rat model of type 2 diabetes. Am J Physiol Endocrinol Metab 2011;301(6):E1119–29.
- Bai Y, Cui W, Xin Y, Miao X, Barati MT, Zhang C, et al. Prevention by sulforaphane of diabetic cardiomyopathy is associated with up-regulation of Nrf2 expression and transcription activation. J Mol Cell Cardiol 2013;57:82–95.
- Miao X, Bai Y, Sun W, Cui W, Xin Y, Wang Y, et al. Sulforaphane prevention of diabetes-induced aortic damage was associated with the up-regulation of Nrf2 and its down-stream antioxidants. Nutr Metab (Lond) 2012;9(1):84.
- Cui W, Bai Y, Miao X, Luo P, Chen Q, Tan Y, et al. Prevention of diabetic nephropathy by sulforaphane: possible role of Nrf2 upregulation and activation. Oxid Med Cell Longev 2012; Sep; ID: 821936, 1–12.
- Chan K, Han X-D, Kan YW. An important function of Nrf2 in combating oxidative stress: detoxification of acetaminophen. Proc Natl Acad Sci 2001;98(8):4611–6.
- Aleksunes LM, Reisman SA, Yeager RL, Goedken MJ, Klaassen CD. Nuclear factor erythroid 2-related factor 2 deletion impairs glucose tolerance and exacerbates hyperglycemia in type 1 diabetic mice. J Pharmacol Exp Ther 2010;333(1):140–51.
- Yoh K, Hirayama A, Ishizaki K, Yamada A, Takeuchi M, Yamagishi S-i, et al. Hyperglycemia induces oxidative and nitrosative stress and increases renal functional impairment in Nrf2-deficient mice. Genes Cells 2008;13(11):1159–70.
- Ungvari Z, Bagi Z, Feher A, Recchia FA, Sonntag WE, Pearson K, et al. Resveratrol confers endothelial protection via activation of the antioxidant transcription factor Nrf2. Am J Physiol Heart Circ Physiol 2010;299(1):H18–24.
- He X, Ma Q. Redox regulation by nuclear factor erythroid 2-related factor 2: gatekeeping for the basal and diabetes-induced expression of thioredoxin-interacting protein. Mol Pharmacol 2012;82(5):887–97.
- Thomas M. A preliminary evaluation of bardoxolone methyl for the treatment of diabetic nephropathy. Expert Opin Drug Metab Toxicol 2012;8(8):1015–22.
- Dreger H, Westphal K, Weller A, Baumann G, Stangl V, Meiners S, et al. Nrf2-dependent upregulation of antioxidative enzymes: a novel pathway for proteasome inhibitor-mediated cardioprotection. Cardiovasc Res 2009;83(2):354–61.
- Tamasi V, Jeffries JM, Arteel GE, Falkner KC. Ebselen augments its peroxidase activity by inducing nrf-2-dependent transcription. Arch Biochem Biophys 2004;431(2):161–8.
- Chew P, Yuen DYC, Koh P, Stefanovic N, Febbraio MA, Kola I, et al. Site-specific antiatherogenic effect of the antioxidant ebselen in the diabetic apolipoprotein E–deficient mouse. Arterioscler Thromb Vasc Biol 2009;29(6):823–30.
- Chew P, Yuen DYC, Stefanovic N, Pete J, Coughlan MT, Jandeleit-Dahm KA, et al. Antiatherosclerotic and renoprotective effects of ebselen in the diabetic apolipoprotein E/GPx1-double knockout mouse. Diabetes 2010;59(12):3198–207.
- Honda T, Rounds BV, Gribble GW, Suh N, Wang Y, Sporn MB. Design and synthesis of 2-cyano-3,12-dioxoolean-1,9-dien-28-oic acid, a novel and highly active inhibitor of nitric oxide production in mouse macrophages. Bioorg Med Chem Letters 1998;8(19):2711–4.
- Honda T-i, Rounds BV, Bore L, Favaloro FG Jr, Gribble GW, Suh N, et al. Novel synthetic oleanane triterpenoids: a series of highly active inhibitors of nitric oxide production in mouse macrophages. Bioorg Med Chem Lett 1999;9(24):3429–34.
- Hong F, Freeman ML, Liebler DC. Identification of sensor cysteines in human Keap1 modified by the cancer chemopreventive agent sulforaphane. Chem Res Toxicol 2005;18(12):1917–26.
- Song MY, Kim EK, Moon WS, Park JW, Kim HJ, So HS, et al. Sulforaphane protects against cytokine- and streptozotocin-induced β-cell damage by suppressing the NF-κB pathway. Toxicol Appl Pharmacol 2009;235(1):57–67.
- Bahadoran Z, Mirmiran P, Hosseinpanah F, Rajab A, Asghari G, Azizi F. Broccoli sprouts powder could improve serum triglyceride and oxidized LDL/LDL-cholesterol ratio in type 2 diabetic patients: a randomized double-blind placebo-controlled clinical trial. Diabetes Res Clin Pract 2012;96(3):348–54.
- Mirmiran P, Bahadoran Z, Hosseinpanah F, Keyzad A, Azizi F. Effects of broccoli sprout with high sulforaphane concentration on inflammatory markers in type 2 diabetic patients: a randomized double-blind placebo-controlled clinical trial. J Functional Foods 2012;4(4):837–41.
- Bahadoran Z, Mirmiran P, Hosseinpanah F, Hedayati M, Hosseinpour-Niazi S, Azizi F. Broccoli sprouts reduce oxidative stress in type 2 diabetes: a randomized double-blind clinical trial. Eur J Clin Nutr 2011;65(8):972–7.
- Liu Y, Chan F, Sun H, Yan J, Fan D, Zhao D, et al. Resveratrol protects human keratinocytes HaCaT cells from UVA-induced oxidative stress damage by downregulating Keap1 expression. Eur J Pharmacol 2011;650(1):130–7.
- Huang K, Huang J, Xie X, Wang S, Chen C, Shen X, et al. Sirt1 resists advanced glycation end products-induced expressions of fibronectin and TGF-β1 by activating the Nrf2/ARE pathway in glomerular mesangial cells. Free Radic Biol Med 2013;65:528–40.
- Jiang B, Guo L, Li BY, Zhen JH, Song J, Peng T, et al. Resveratrol attenuates early diabetic nephropathy by down-regulating glutathione S-transferases Mu in diabetic rats. J Med Food 2013;16(6):481–6.
- Ghanim H, Sia CL, Korzeniewski K, Lohano T, Abuaysheh S, Marumganti A, et al. A resveratrol and polyphenol preparation suppresses oxidative and inflammatory stress response to a high-fat, high-carbohydrate meal. J Clin Endocrinol Metab 2011;96(5):1409–14.
- Brasnyó P, Molnár GA, Mohás M, Markó L, Laczy B, Cseh J, et al. Resveratrol improves insulin sensitivity, reduces oxidative stress and activates the Akt pathway in type 2 diabetic patients. Br J Nutr 2011;106(3):383–9.
- Pervaiz S, Holme AL. Resveratrol: its biologic targets and functional activity. Antioxid Redox Signal 2009;11(11):2851–97.
- Turan B, Tuncay E, Vassort G. Resveratrol and diabetic cardiac function: focus on recent in vitro and in vivo studies. J Bioenerg Biomembr 2012;44(2):281–96.
- Khan HY, Zubair H, Faisal M, Ullah MF, Farhan M, Sarkar FH, et al. Plant polyphenol induced cell death in human cancer cells involves mobilization of intracellular copper ions and reactive oxygen species generation: a mechanism for cancer chemopreventive action. Mol Nutr Food Res 2013 Oct; [Epub ahead of print].
- Zheng LF, Wei QY, Cai YJ, Fang JG, Zhou B, Yang L, et al. DNA damage induced by resveratrol and its synthetic analogues in the presence of Cu (II) ions: mechanism and structure-activity relationship. Free Radic Biol Med 2006;41(12):1807–16.
- de la Lastra CA, Villegas I. Resveratrol as an antioxidant and pro-oxidant agent: mechanisms and clinical implications. Biochem Soc Trans 2007;35(5):1156–60.
- Vang O, Ahmad N, Baile CA, Baur JA, Brown K, Csiszar A, et al. What is new for an old molecule? systematic review and recommendations on the use of resveratrol. PLoS ONE 2011;6(6):e19881.
- Hu J, Klein JD, Du J, Wang XH. Cardiac muscle protein catabolism in diabetes mellitus: activation of the ubiquitin-proteasome system by insulin deficiency. Endocrinology 2008;149(11):5384–90.
- Marfella R, Filippo CD, Portoghese M, Siniscalchi M, Martis S, Ferraraccio F, et al. The ubiquitin–proteasome system contributes to the inflammatory injury in ischemic diabetic myocardium: the role of glycemic control. Cardiovasc Pathol 2009;18(6):332–45.
- Wang X, Hu Z, Hu J, Du J, Mitch WE. Insulin resistance accelerates muscle protein degradation: activation of the ubiquitin-proteasome pathway by defects in muscle cell signaling. Endocrinology 2006;147(9):4160–8.
- Wang Y, Sun W, Du B, Miao X, Bai Y, Xin Y, et al. Therapeutic effect of MG-132 on diabetic cardiomyopathy is associated with its suppression of proteasomal activities: roles of Nrf2 and NF-κB. Am J Physiol Heart Circ Physiol 2013;304(4):H567–78.
- Cui W, Li B, Bai Y, Miao X, Chen Q, Sun W, et al. Potential role for Nrf2 activation in the therapeutic effect of MG132 on diabetic nephropathy in OVE26 diabetic mice. Am J Physiol Endocrinol Metab 2013;304(1):E87–99.
- Miao X, Cui W, Sun W, Xin Y, Wang B, Tan Y, et al. Therapeutic effect of MG132 on the aortic oxidative damage and inflammatory response in OVE26 type 1 diabetic mice. Oxid Med Cell Longev. 2013 March; ID: 879516, 1–12.
- Guo N, Peng Z. MG132, a proteasome inhibitor, induces apoptosis in tumor cells. Asia Pac J Clin Oncol 2013;9(1):6–11.
- Dinkova-Kostova AT, Liby KT, Stephenson KK, Holtzclaw WD, Gao X, Suh N, et al. Extremely potent triterpenoid inducers of the phase 2 response: correlations of protection against oxidant and inflammatory stress. Proc Natl Acad Sci USA 2005;102(12):4584–9.
- Sporn MB, Liby KT, Yore MM, Fu L, Lopchuk JM, Gribble GW. New synthetic triterpenoids: potent agents for prevention and treatment of tissue injury caused by inflammatory and oxidative stress. J Nat Prod 2011;74(3):537–45.
- Ichikawa T, Li J, Meyer CJ, Janicki JS, Hannink M, Cui T. Dihydro-CDDO-trifluoroethyl amide (dh404), a novel Nrf2 activator, suppresses oxidative stress in cardiomyocytes. PLoS ONE 2009;4(12):e8391.
- Zoja C, Corna D, Nava V, Locatelli M, Abbate M, Gaspari F, et al. Analogs of bardoxolone methyl worsen diabetic nephropathy in rats with additional adverse effects. Am J Physiol Renal Physiol 2013;304(6):F808–19.
- Chin M, Lee C-YI, Chuang J-C, Bumeister R, Wigley WC, Sonis ST, et al. Bardoxolone methyl analogs RTA 405 and dh404 are well tolerated and exhibit efficacy in rodent models of Type 2 diabetes and obesity. Am J Physiol Renal Physiol 2013;304(12):F1438–46.
- Pergola PE, Raskin P, Toto RD, Meyer CJ, Huff JW, Grossman EB, et al. Bardoxolone methyl and kidney function in CKD with type 2 diabetes. N Engl J Med 2011;365(4):327–36.
- McCullough PA, Ali S. Cardiac and renal function in patients with type 2 diabetes who have chronic kidney disease: potential effects of bardoxolone methyl. Drug Des Devel Ther 2012;6:141–9.
- Upadhyay A, Sarnak MJ, Levey AS. Bardoxolone methyl, chronic kidney disease, and type 2 diabetes. N Engl J Med 2011;365(18):1746.
- Ding Y, Stidham RD, Bumeister R, Trevino I, Winters A, Sprouse M, et al. The synthetic triterpenoid, RTA 405, increases the glomerular filtration rate and reduces angiotensin II-induced contraction of glomerular mesangial cells. Kidney Int [Commentary] 2013;83(5):845–54.
- Abboud HE. Synthetic oleanane triterpenoids: magic bullets or not? Kidney Int 2013;83(5):785–7.
- Hong DS, Kurzrock R, Supko JG, He X, Naing A, Wheler J, et al. A phase I first-in-human trial of bardoxolone methyl in patients with advanced solid tumors and lymphomas. Clin Cancer Res 2012;18(12):3396–406.
- Pergola PE, Krauth M, Huff JW, Ferguson DA, Ruiz S, Meyer CJ, et al. Effect of bardoxolone methyl on kidney function in patients with T2D and Stage 3b-4 CKD. Am J Nephrol 2011;33(5):469–76.
- McMahon G, Forman J. Bardoxolone methyl, chronic kidney disease, and type 2 diabetes. N Engl J Med 2011;365(18):1746.
- de Zeeuw D, Akizawa T, Audhya P, Bakris GL, Chin M, Christ-Schmidt H, et al. Bardoxolone methyl in type 2 diabetes and stage 4 chronic kidney disease. N Engl J Med 2013;369(26):2492–503.
- Wakabayashi N, Itoh K, Wakabayashi J, Motohashi H, Noda S, Takahashi S, et al. Keap1-null mutation leads to postnatal lethality due to constitutive Nrf2 activation. Nat Genet 2003;35(3):238–45.
- Suzuki T, Motohashi H, Yamamoto M. Toward clinical application of the Keap1–Nrf2 pathway. Trends Pharmacol Sci 2013;34(6):340–6.
- Xu J, Kulkarni SR, Donepudi AC, More VR, Slitt AL. Enhanced Nrf2 activity worsens insulin resistance, impairs lipid accumulation in adipose tissue, and increases hepatic steatosis in leptin-deficient mice. Diabetes 2012;61(12):3208–18.
- Xue P, Hou Y, Chen Y, Yang B, Fu J, Zheng H, et al. Adipose deficiency of Nrf2 in ob/ob mice results in severe metabolic syndrome. Diabetes 2013;62(3):845–54.
- Pi J, Zhang Q, Fu J, Woods CG, Hou Y, Corkey BE, et al. ROS signaling, oxidative stress and Nrf2 in pancreatic beta-cell function. Toxicol Appl Pharmacol 2010;244(1):77–83.
- Bjelakovic G, Nikolova D, Gluud L, Simonetti RG, Gluud C. Mortality in randomized trials of antioxidant supplements for primary and secondary prevention: systematic review and meta-analysis. JAMA 2007;297(8):842–57.