Abstract
Objectives
To assess the cardioprotective effect of the Salsola kali aqueous extract against adriamycin (ADR)-induced cardiotoxicity in male Swiss albino mice.
Methods
The aqueous extract of S. kali was phytochemically screened by traditional methods for different classes and further evaluated for antioxidant activity in vitro. In vivo, cardioprotective evaluation of the extract was designed to have four groups of mice: (1) control group (distilled water, orally; normal saline, intraperitoneally (i.p.)); (2) ADR group (15 mg/kg, i.p.); (3) aqueous S. kali extract (200 mg/kg, orally); and (4) ADR + S. kali group. ADR (5 mg/kg) was injected three times over 2 weeks while S. kali was orally administered daily for 3 weeks (1 week before and 2 weeks during ADR treatment). Cardioprotective properties were assessed using biochemical and histopathological approaches.
Results
ADR caused a significant increase in serum enzymes (lactate dehydrogenase, creatine phosphokinase, aspartate aminotransferase, and alanine aminotransferase). Myocardial levels of malondialdehyde, nitric oxide, and reduced glutathione, as well as the activities of superoxide dismutase and catalase increased while the activities of glutathione peroxidase and glutathione S-transferase declined. Histopathological examination of heart sections revealed that ADR caused myofibrils loss, necrosis and cytoplasmic vacuolization.
Discussion
Pretreatment with S. kali aqueous extract normalized serum and antioxidant enzymes minimized lipid peroxidation and cardiac damage. These results have suggested that the extract has antioxidant activity, indicating that the mechanism of cardioprotection during ADR treatment is mediated by lowering oxidative stress.
Introduction
Adriamycin (ADR) is an anthracycline-based anti-cancer drug that has been widely used for the treatment of leukemia, lymphomas, and various solid tumors of the lung, breast, thyroid, and ovary.Citation1,Citation2 However, its therapeutic use has been limited due to the incidence of cardiotoxicity, the risk of delayed cardiovascular manifestations,Citation3 and other toxicities including renal, hematological, and testicular toxicities.Citation4
ADR cardiotoxicity can develop after an acute dose as well as cumulative doses.Citation5 The acute side effects may develop within minutes after intravenous administration of the drug in which they are reversible and clinically manageable.Citation6 The chronic cardiotoxicity is characterized by dilated cardiomyopathy and congestive heart failure.Citation7 It is considered largely irreversible due to recurrent damage of cardiomyocytes.Citation8
Several molecular mechanisms for the ADR-induced cardiotoxicity have been reported, including lipid peroxidation (LPO), free radical formation, mitochondrial damage,Citation9 impairment of adrenergic stimulation, alteration in calcium homeostasis, infiltration of inflammatory cells, accumulation of fat,Citation10 and interference with various pro-survival kinases.Citation11
The oxidative stress implicated in ADR cardiotoxicity involves one-electron reduction of a quinone localized in the anthracycline moiety of ADR, catalyzed by NADPH as a reducing agent to generate a semiquinone free radical, which then regenerates the parent compound by oxidization with molecular oxygen.Citation12 The metal ions (Fe+2/+3) can take part in reactive oxygen species (ROS) generation especially the highly reactive hydroxyl radical (OH•) via the reduction of hydrogen peroxide (H2O2).Citation13
The first targets of ROS are cellular membranes, which are rich in lipids prone to peroxidation causing the production of many relatively stable and highly toxic aldehydes, such as malondialdehyde (MDA). These aldehydes can easily cause damage to lipids, DNA, and proteins. Also, they can attack macromolecular targets far from where they were generated, acting as second cytotoxic messengers.Citation14 Therefore, protective strategies have focused on administering drugs or natural products that scavenge ADR-derived ROS. This approach has been validated in vivo, in which acute and/or chronic cardiotoxicity were reduced utilizing different natural products.Citation15
In plants, several secondary metabolite classes have demonstrated antioxidative properties such as vitamins, terpenoids, alkaloids, and polyphenols.Citation16,Citation17 Salsola species (family: Chenopodiaceae) are well known in folk medicine for their anti-hypertensive, diuretic, anti-cancer, emollient, diuretic, purgative, anti-ulcer and anti-inflammatory properties.Citation18,Citation19 S. foetida contains phenolic compounds that exhibit tyrosinase inhibitory activity, and are used in the treatment of dermatological disorders associated with melanin hyperpigmentation.Citation20 It has been suggested that S. oppositifolia, S. soda, and S. tragus alkaloid extracts have potential roles in the treatment of Alzheimer's disease; S. tragus alkaloid extract exerts a high inhibitory activity against both acetylcholinesterase and butyrylcholinesterase, while S. soda and S. oppositifolia alkaloid extracts exhibit a selective inhibitory activity against butyrylcholinesterase.Citation21 The significant antioxidant activities of many species like S. baryosma, S. foetida, S. komarovii, and others have been attributed to the different antioxidants they contain such as poly-oxygenated triterpenes, phenolic acids, acetophenones, coumarins, sterols, and flavonoids.Citation19,Citation20,Citation22,Citation23
Salsola kali, an annual herb, is commonly found in arid and semi-arid areas such as regions of North Africa, South Europe, USA, and Asia. It has been used in traditional medicinal systems in the treatment of hypertension as well as acting as an angiotensin-converting enzyme inhibitor.Citation19,Citation24 In addition to the use in traditional medicine, the plant has been investigated for its antioxidant and antibacterial activities.Citation25 De la Rosa et al. have reported the production of low-molecular weight thiols by S. kali, such as reduced glutathione (GSH), as well as the increase in the antioxidative enzyme catalase (CAT) in response to cadmium uptake associated oxidative stress. These changes were considered as potential mechanisms underlying cadmium uptake and its associated resistance to high cadmium concentrations.Citation26 Novel isoflavones have been successfully isolated from the roots of other Chenopodiaceae species.Citation27
Recently, 11 phenolic acids have been identified from the herb and root of S. kaliCitation28 including the well-known antioxidant, ferulic acid, in which it has been found to be the most abundant.Citation29 Furthermore, two isochinolin alkaloids: salsoline and salsolidine, were found to represent a small composition of the herb (about 0.2%).Citation30 Also, some antioxidant fatty acids like linolenic, arachidonic, palmitic and stearic acids have been identified together with 4-desmethylsterols derivatives.Citation31,Citation32 All these promising antioxidant data reported for S. kali and the abundance of S. kali in our area, and since ADR-induced cardiotoxicity is linked to oxidative stress, we have investigated the possible effect of S. kali aqueous extract against ADR-induced cardiotoxicity and also the changes in oxidant–antioxidant status of an experimental mice model.
Materials and methods
Chemicals
The ADR hydrochloride (Adricin®, 50 mg) vial was purchased from EIMC United Pharmaceuticals, Egypt. All other chemicals and solvents were of the highest purity and analytical grade (Sigma, USA). Aspartate aminotransferase (AST), alanine aminotransferase (ALT), glutathione peroxidase (GPx), superoxide dismutase (SOD), nitric oxide (NO), glutathione S-transferase (GST), MDA, GSH, and CAT diagnostic kits were purchased from Biodiagnostic Co. (Egypt), creatine phosphokinase (CPK) and lactate dehydrogenase (LDH) kits were purchased from ELITech Group, France.
S. kali collection and extraction
Whole shrubs (root, stem, leaves, and flowers) were collected from the salty grounds along a coastal road in New Damietta City, Damietta governorate, Egypt. The crude aqueous extract of S. kali was prepared as previously reported.Citation33 The whole parts of S. kali were washed and dried in oven at 45°C for 72 hours, then ground into a fine powder. Twenty grams of this powder was soaked in 100 ml of distilled water at 50°C and then kept for 24 hours with occasional shaking. Finally, the extract was allowed to cool then centrifuged at 6000 rpm for 45 minutes. The supernatant was collected and stored at −20°C for further use.
Phytochemical screening
The aqueous extract was chemically tested by standard procedures to investigate the presence of saponins, terpenoids, tannins, flavonoids, steroids, phlobatannins and cardiac glycosides.Citation34
Total flavonoids content
According to the method of Chang et al., aluminium chloride forms acid complexes with flavonoids in which their colors are measured at 415 nm.Citation35 The flavonoids content was expressed as standard grams of quercetin equivalents per 100 g dry weight of plant (g QE/100 g dry weight). Sample was assayed in triplicate.
Total phenolics content
The Folin-Ciocalteu reagent was used to determine the phenolic content of the extract.Citation36 The total phenolics content was expressed as grams of gallic acid equivalents per 100 g dry weight of plant (g GAE/100 g dry weight). Sample was assayed in triplicate.
Reducing power assay
This method was used to measure the capability of extract to reduce Fe3+/ferricyanide complex to the ferrous form (Fe2+). The Fe2+ was monitored by measuring the formation of blue color at 700 nm. The higher absorbance of the reaction mixture indicates greater reducing power,Citation37 ascorbic acid was used for the construction of the standard curve. The concentration was expressed as millimole of ascorbic acid equivalents per gram of dry weight of plant (AAE/g dry weight). Sample was assayed in triplicate.
Superoxide-dismutase like (mimetic) activity
The ability of the extract to inhibit phenazine methosulphate-mediated reduction of nitroblue tetrazolium dye was evaluated.Citation38 The percentage inhibition of superoxide anion generation was calculated for different concentrations of the extract against a reagent blank containing distilled water in place of extract. The absorbance values were then plotted against the different concentrations of the aqueous S. kali extract. Each extract concentration was assayed in triplicate ().
Animals and experimental design
Animals
Male Swiss albino mice (mean weight 22–25 g) were purchased from Theodor Bilharz research institute, Egypt. The animals were acclimatized to the animal house conditions for 1 week and maintained in a well-ventilated animal quarter with 12 hours light/dark exposure. The mice were fed a standard mice pellet diet and drinking water ad libitum.
Acute toxicity test of the extract
Male Swiss albino mice were randomly divided into 10 groups (10 animals per group). The first group received only distilled water. The other nine groups received 1, 2, 3, 4, 5, 6, 7, 8, and 9 g extract/kg body weight respectively by intragastric intubation. Then, all mice groups were observed systematically for 24 hours for intoxication syndromes and number of deaths.Citation39 The numbers of dead mice were recorded and used for the calculation of the median lethal dose (LD50) of S. kali extract.
Animal treatment
The animals were split into four groups of 10 animals each. The first group (normal control) received distilled water (intragastrically, daily for 3 weeks) and normal saline (intraperitoneally (i.p.), three times during the last two weeks). The second group was i.p. injected with ADR (5 mg/kg body weight), three times over the last 2 weeks for a cumulative dose of 15 mg/kg, a dose that is well known to induce cardiotoxicity.Citation40 This group also received distilled water intragastrically under the same conditions as the control group. The third group received S. kali extract (200 mg/kg body weight intragastrically, daily for 3 weeks). This dose was chosen according to the LD50 of the extract. The fourth group received S. kali extract daily as a pre- and post-treatment (7 days before the first ADR injection, and daily thereafter throughout the study) along with ADR injection (dosage and duration were as same as the second group). At the end of the third week, and 24 hours after the last intragastric administration, the animals were sacrificed. Blood samples and heart specimens were collected. Sera were separated for subsequent assays. Heart specimens were washed with ice-cold normal saline and homogenized quickly in ice-cold phosphate buffer (pH 7.40) containing 1.15% KCl to yield 10% homogenate. Also, formalin-fixed heart specimens were used for histopathological examination.
Sample collection and biochemical assays
Serum was used for the kinetic determination of LDH activity according to the recommendations of the French Society of Clinical Biology.Citation41 CPK activity was assayed according to the method of Hørder et al.Citation42 AST and ALT activities were colorimetrically measured.Citation43
In heart tissues, MDA was assayed according to Kei method.Citation44 The activity of CAT was determined by a colorimetric method.Citation45 SOD activity was determined by the method of Nishikimi et al.Citation38 GPx activity was assayed by a kinetic method.Citation46 GST activity was assayed spectrophotometerically using 1-chloro-2,4-dinitrobenzene and glutathione.Citation47 GSH was determined by Ellman method, based on the reaction of thiols with the chromogenic 5,5′-dithiobis-2-nitrobenzoate whereby a yellow dianion of 5-thio-2-nitrobenzoic acid was formed and colorimetrically measured.Citation48 Cardiac content of nitrite was estimated as an index of NO level.Citation49 Finally, the protein content of different fractions resulting from ultracentrifugation of heart homogenate was determined by the method of Lowry et al.Citation50
Histopathological examination of heart sections
Formalin-fixed heart sections were embedded in paraffin wax, serially sectioned (5 µm), and stained with hematoxylin and eosin for assessment of histopathological changes. Sections were examined and assigned grades according to Billingham et al.Citation51 Grade 0: no change from normal; Grade 1: less than 5% of myofibrillar loss; Grade 2: 5–30% of myofibrillar loss (marked myofibrillar loss and cytoplasmic vacuolization); Grade 3: >30% of myofibrillar loss (diffuse cell damage).
Statistical analysis
Results were represented as mean ± standard deviation (SD). Comparison between different groups was carried out by one-way analysis of variance test and LSD test to identify differences between the groups. Statistical significance was acceptable to a level of P < 0.05.
Results
Acute toxicity of S. kali extract in mice
The acute toxicity data indicated that the LD50 value for the oral dose of S. kali aqueous extract was 5.416 ± 0.012 g/kg body weight.
Phytochemical screening of the plant extract, content of total flavonoids, total phenolics, and reducing power activity
The phytochemical screening of the plant confirmed that the extract contains flavonoids, terpenoids, saponins, cardiac glycosides, and tannins but it was negative for the presence of phlobataninns and steroids. The total flavonoids content of the aqueous S. kali extract was 0.697 ± 0.01 g QE/100 g dry weight of plant, whereas the phenolic content was 1.36 ± 0.02 g GAE/100 g dry weight of plant. In addition, the extract was found to be capable of reducing Fe3+ to Fe2+ when compared with ascorbic acid as a reference compound. This reducing activity was equal to 9.67 ± 0.01 mmolar AAE/g dry weight of plant.
Superoxide-dismutase like (mimetic) activity
The SOD mimetic activity of the extract was directly proportional to the extract concentration (). The percentage of inhibition was the maximum (100%) at a concentration of 28.71 ± 0.00 mg/ml. This result showed the ability of the extract to quench superoxide radicals in a concentration dependent behavior.
Effects of ADR and S. kali treatment on serum LDH, CPK, ALT, and AST activities
In , the activities of marker enzymes (LDH, CPK, ALT, and AST) in the sera of ADR group were significantly increased compared with those of the control group. In addition, the oral administration of S. kali extract significantly (P < 0.001) prevented the ADR damage to heart tissues compared with the mice which were injected with ADR alone (group 2).
Table 1. Effect of Salsola kali extract on the activities of diagnostic marker enzymes (LDH, CPK, ALT, and AST) in the serum of control and experimental groups of mice
Effects of ADR and S. kali treatment on cardiac MDA, GSH, NO levels and SOD, CAT, GST, and GPx activities
The results of these parameters are summarized in Tables and . MDA and NO levels were significantly increased in cardiac tissues of the mice injected with ADR alone compared with those of the control group (P < 0.001). Also, cardiac GSH level was significantly increased (P < 0.05). On the other hand, the heart tissues of mice treated with S. kali alone showed a high decrease in the cardiac MDA content (P < 0.001) compared with that of the control group. Therefore, pre- and post-treatment with S. kali extract provided a marked normalization in MDA, NO, and GSH concentrations compared with the group treated with ADR alone (P < 0.001).
Table 2. Effect of Salsola kali extract on the concentrations of oxidative, nitrosative markers (MDA, NO), and GSH in the heart homogenate of control and experimental groups of mice
Table 3. Effect of Salsola kali extract on the activities of antioxidant enzymes (CAT, GST, GPx, and SOD) in the heart homogenate of control and experimental groups
Cardiac CAT and SOD activities were significantly increased in ADR-treated group (P < 0.01 and <0.001, respectively) in comparison with those of the control group. On the other hand, the activities of these two enzymes were decreased in heart tissues of S. kali + ADR group compared with those of ADR group, but this decrease was only significant in case of SOD (P < 0.001). Non-significant decrease in GPx activity was observed in ADR injected mice in comparison with control mice. This decrease was normalized in S. kali + ADR-treated group. On the other hand, GST was significantly decreased (P < 0.01) in ADR group, but pretreatment with S. kali caused its increase in S. kali + ADR-treated mice group (P < 0.01) compared with ADR group.
Effects of ADR and S. kali treatment on heart histology
Histological changes in the heart tissues were microscopically examined, evaluated, and the results were presented in . Normal histological findings were observed in the control group and S. kali groups (grade 0) (A and B). On the other hand, histopathological changes were found in the heart tissues of ADR-treated group such as loss of myofibrils and focal cytoplasmic vacuolization in about 15% of cells (grade 2). Necrosis, lymphocytic infiltration, and perinuclear vacuolization were also observed (C and D). The myofibrillar loss was reduced to less than 5% of cells in S. kali + ADR group in comparison with that of ADR group (grade 1) (E).
Figure 2. Photomicrograph of mouse heart from (A) control mice showing normal morphology (magnification: ×40) (scale bar = 500 µm); (B) heart section of S. kali-treated mice showing normal morphology (magnification: ×20) (scale bar = 500 µm). (C) Heart section of ADR-treated mice showing necrosis (N) and lymphocytic infiltration (Ly) (magnification: ×20) (scale bar = 1000 µm); (D) Heart section of ADR-treated mice showing perinuclear vacuolization (V) (magnification: ×20) (scale bar = 1000 µm); (E) Heart section of S. kali + ADR treated mice showing no significant necrosis of muscle fiber with mild edema (magnification: ×20) (scale bar = 1000 µm).
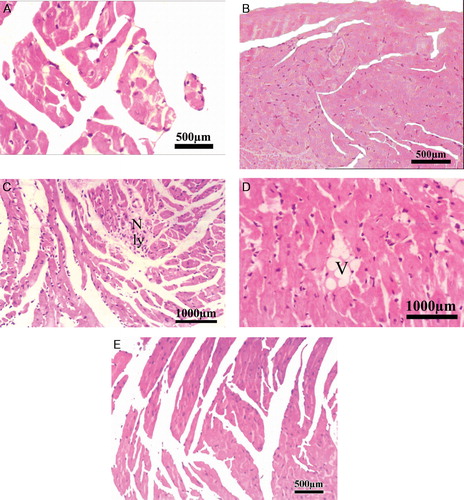
Table 4. Grading of histopathological changes in the heart of mice treated with ADR and Salsola kali aqueous extract
Discussion
Oxidative stress resulting from ROS generation was proposed to be the major cause of ADR-induced cardiotoxicity. This hypothesis is supported by the protection of cardiomyocytes from death by the administration of antioxidants.Citation52,Citation53 Adult myocytes are terminally differentiated cells with highly oxidative metabolism and limited oxidative defenses. Thus, the heart is very sensitive to free radical damage.Citation53 ADR is an anticancer drug that has shown cardiotoxicity. In this study, we evaluated the chronic cardiotoxicity of ADR in mice. We found that a cumulative dose of ADR (15 mg/kg) induced chronic cardiotoxicity. It was demonstrated by an increase in serum LDH, CPK, AST, and ALT activities, high LPO and change of oxidant–antioxidant status in the heart. This was further confirmed by cardiac histopathological changes including myofibrillar loss and cytoplasmic vacuolization. Olson and Copen have hypothesized that ADR binds to the membrane lipid (cardiolipin) that alters the membrane fluidity, modifies calcium transport system and facilitates the loss of intracellular enzymes from injured myocardium.Citation54 This explains the high activities of serum enzymes (LDH, CPK, AST, and ALT) in ADR-injected mice. These results were consistent with earlier studies.Citation10,Citation55 According to Abd-Allah and co-authors, we have suggested that ADR may induce generalized toxicity in mice causing elevated levels of various enzymes including ALT and AST.Citation56 The histopathological changes observed in the heart sections of ADR-injected mice confirmed the previous enzymatic changes as well as cardiotoxicity.
Moreover, we found a significant increase in MDA and NO levels in ADR-injected mice. This supports the hypothesis that ADR-induced cardiotoxicity is caused by free radicals mediated mechanisms including LPO.Citation14 Several studies have demonstrated the capacity of ADR to induce reactive nitrogen species (RNS) production in cardiomyocytes such as peroxynitrite and NO with a resultant accumulation of nitrite in cells.Citation57,Citation58 These results were similar to previous studies in rats and mice.Citation55,Citation58,Citation59 Conversely to other studies, our data showed that chronic administration of ADR increased cardiac GSH levels. Salvemini and co-authors have reported that the induction of GSH synthesis in cells exposed to oxidative stress could be an adaptive process or a defense mechanism of the cells to combat oxidative stress.Citation60 However, a previous study in rabbits has suggested that GSH elevation is consistent with activation of the glutathione–GPx redox pathway secondary to the generation of ADR-induced free radicals and their subsequent detoxification.Citation61 This change has also been observed by Boor using a rat model, where GSH levels increased with low cumulative doses of ADR, while the highest dose of ADR (50 mg/kg) caused a decline in GSH levels.Citation62 High levels of GSH may also be resulted from insufficient oxidation of GSH due to reduced cardiac GPx activity.Citation63
We found that there is a significant increase in the activities of cardiac SOD and CAT which was similar to what have been reported previously by Adachi et al.Citation64 This was accompanied by a decrease in GPx activity. The elevation of CAT and SOD activities could be an indicator for enhanced free radical generation in cardiac tissues, especially H2O2 produced during ADR redox-cycle.Citation65 Furthermore, Yin et al. have demonstrated that ADR increased the mRNA levels of CAT, SOD, and GPx to compensate for their consumption in the detoxification of ADR-induced free radicals; however, only CAT activity was increased.Citation66 GPx activities among different groups were quite similar. This does not rule out the presence of ROS; it suggests that the cardiac detoxification system is not overwhelmed.Citation61,Citation67 This may be changed when the oxidative stress is increased by ADR injection at higher doses and longer treatment duration. Furthermore, GST activity was decreased in ADR group. Its role in the detoxification and excretion of xenobiotics could be the reason for its consumption.Citation67
Recent studies have similarly revealed the occurrence of myocardial necrosis, myofibrillar loss, and cytoplasmic vacuolization in ADR-exposed mice.Citation59,Citation68 These damages may eventually lead to progressive myocytolysis and decrease of antioxidative enzymes.Citation69 Our results showed that there is elevation of serum cardiac enzymes LDH, CPK and AST accompanied by disturbances in the activities of the antioxidant enzymes (CAT, SOD, GPx, and GST).
To solve this problem, biological antioxidant compounds may contribute to the protection of heart tissues against the deleterious effects induced by ADR.Citation70 Medicinal plants could be a source of these antioxidants. S. kali is a desert plant species that has been proven to have antibacterial activityCitation25 and antioxidant properties. It has been analyzed to reveal its content of polyphenols, flavonoids, and phenolic acids that possess antioxidant activity and can reduce lipid levels in the sera and tissues of animals with induced hyperlipidemia.Citation28,Citation71 According to our results, this could be the reason for improving the antioxidant status of heart tissues in the S. kali + ADR group through the restoration of normal levels of cardiac antioxidative enzymes and GSH, decrease in NO levels and reduction of hyperlipidemia.
Being a halophyte, S. kali has the ability to withstand salt-triggered oxidative stress by biochemical mechanisms that facilitate retention of water, protect chloroplast functioning and maintain ion homeostasis through its powerful antioxidant system that includes enzymatic and non-enzymatic components.Citation29,Citation72 Polyphenol synthesis, a mechanismthat is stimulated in response to stresses such as salinity, plays a role in adaptation to these conditions.Citation73
We have shown that S. kali aqueous extract possesses antioxidant activity by the measurement of SOD-like and reducing power activities. This was suggested to be due to the presence of various known antioxidant secondary metabolites such as phenolic acids, flavonoids, and tannins. This was in agreement with previous studies.Citation28,Citation29 In this study, S. kali aqueous extract administration alone had no effects on sera and cardiac tissues of administered mice, except for an elevation of cardiac GST activity and a reduction in cardiac MDA level and serum LDH activity. These changes might be considered as an improvement of the weak cardiac antioxidant defenses and heart function.
Owing to these radical-scavenging and iron-chelating properties, flavonoids which are produced in significant amounts by S. kali have been suggested to be potential protectors against chronic cardiotoxicity caused by ADR.Citation15 Therefore, these flavonoids could not only reduce the cardiotoxic effects, but also enhance ADR-induced antitumor activity and increase its concentration in tumors through the inhibition of ADR efflux, as has been demonstrated for some polyphenols of green tea.Citation15,Citation74
We found that the combination of S. kali extract with ADR injection normalized sera marker enzymes activities (LDH, CPK, ALT, and AST) indicating the reduction of membrane disturbances and restoration of its stabilization,Citation10 which was confirmed by reduction in scores of myocardial lesions induced by ADR as was reported in an earlier study.Citation59
Conclusions
This study confirmed that ADR-induced cardiotoxicity could be related to oxidative stress and subsequent LPO in heart tissues. It has been suggested that S. kali aqueous extract had a potential antioxidant activity which ameliorated ADR-induced cardiotoxicity in male albino mice. The protective mechanisms may be caused by the inhibition of LPO and enhancement of antioxidant status in the heart. Further investigations are necessary to use different extraction methods and different fractions of S. kali to investigate their therapeutic properties and possible mechanisms of cardioprotective action, as well as their possible effect(s) on the antitumor activity of ADR.
Disclaimer statements
Contributor/Guarantor Statement
None.
Funding
None.
Conflicts of interest
We all authors disclose here that there are no conflicts of interest that could inappropriately influence the outcome of the present study.
Ethics approval
Ethical approval was provided by Directorate of Veterinary Medicine in Cairo, Egypt.
Acknowledgements
The authors wish to thank Prof Mamdouh S. Serag (Botany Department, Damietta University) for his valuable assistance in plant taxonomic identification.
References
- Minotti G, Menna P, Salvatorelli E, Cairo G, Gianni L. Anthracyclines: molecular advances and pharmacologic developments in antitumor activity and cardiotoxicity. Pharmacol Rev 2004;56(2):185–229.
- Kim DS, Kim HR, Woo ER, Hong ST, Chae HJ, Chae SW. Inhibitory effects of rosmarinic acid on adriamycin-induced apoptosis in H9c2 cardiac muscle cells by inhibiting reactive oxygen species and the activations of c-Jun N-terminal kinase and extracellular signal-regulated kinase. Biochem Pharmacol 2005;70(7):1066–78.
- Menna P, Salvatorelli E, Minotti G. Cardiotoxicity of antitumor drugs. Chem Res Toxicol 2008;21(5):978–89.
- Yilmaz S, Atessahin A, Sahna E, Karahan I, Ozer S. Protective effect of lycopene on adriamycin-induced cardiotoxicity and nephrotoxicity. Toxicology 2006;218:164–71.
- Doroshow JH. Doxorubicin-induced cardiac toxicity. N Engl J Med 1991;324(12):843–5.
- Lefrak EA, Pitha J, Rosenheim S, Gottlieb JA. A clinicopathologic analysis of adriamycin cardiotoxicity. Cancer 1973;32(2):302–14.
- Buyukokuroglu ME, Taysi S, Buyukavci M, Bakan E. Prevention of acute adriamycin cardiotoxicity by dantrolene in rats. Hum Exp Toxicol 2004;23(5):251–6.
- Allen A. The cardiotoxicity of chemotherapeutic drugs. Semin Oncol 1992;19(5):529–42.
- Balachandar AV, Malarkodi KP, Varalakshmi P. Protective role of DLalpha-lipoic acid against adriamycin-induced cardiac lipid peroxidation. Hum Exp Toxicol 2003;22(5):249–54.
- Rajaprabhu D, Rajesh R, Jeyakumar R, Buddhan S, Ganesan B, Anandan R. Protective effect of Picrorhiza kurroa on antioxidant defense status in adriamycin-induced cardiomyopathy in rats. J Med Plant Res 2007;1(4):80–5.
- Peng X, Chen B, Lim CC, Sawyer DB. The cardiotoxicology of anthracycline chemotherapeutics: translating molecular mechanism into preventative medicine. Mol Interv 2005;5(3):163–71.
- Venditti P, Balestrieri M, De Leo T, Di Meo S. Free radical involvement in doxorubicin-induced electrophysiological alterations in rat papillary muscle fibres. Cardiovas Res 1998;38(3):695–702.
- Simunek T, Sterba M, Popelova O, Adamcova M, Hrdina R, Gersl V. Anthracycline-induced cardiotoxicity: overview of studies examining the roles of oxidative stress and free cellular iron. Pharmacol Rep: PR 2009;61(1):154–71.
- Luo X, Evrovsky Y, Cole D, Trines J, Benson LN, Lehotay DC. Doxorubicin-induced acute changes in cytotoxic aldehydes, antioxidant status and cardiac function in the rat. Biochim Biophys Acta 1997;1360(1):45–52.
- Quiles JL, Huertas JR, Battino M, Mataix J, Ramirez-Tortosa MC. Antioxidant nutrients and adriamycin toxicity. Toxicology 2002;180(1):79–95.
- Halliwell B, Gutteridge J. Free radicals in biology and medicine. 4th ed. Oxford: Clarendon Press; 2007.
- Ramamoorthy PK, Bono A. Antioxidant activity, total phenolic and flavonoid content of Morinda citrifolia fruit extracts from various extraction processes. J Eng Sci Technol 2007;2(1):70–80.
- Fu SY. Salsola ruthenica in treatment of essential hypertension. Zhonghua nei ke za zhi (Chin J Internal Med) 1959;7:977–81.
- Loizzo MR, Tundis R, Statti GA, Passalacqua NG, Peruzzi L, Menichini F. In vitro angiotensin converting enzyme inhibiting activity of Salsola oppositifolia Desf., Salsola soda L. and Salsola tragus L. Nat Product Res 2007;21(9):846–51.
- Khan KM, Maharvi GM, Abbaskhan A, Hayat S, Khan MTH, Makhmoor T, et al. Three tyrosinase inhibitors and antioxidant compounds from Salsola foetida. Helvetica Chim Acta 2003;86(2):457–64.
- Tundis R, Menichini F, Conforti F, Loizzo MR, Bonesi M, Statti G. A potential role of alkaloid extracts from Salsola species (Chenopodiaceae) in the treatment of Alzheimer's disease. J Enzyme Inhib Med Chem 2009;24(3):818–24.
- Lee HJ, Pan C-H, Kim E-S, Kim CY. Online high performance liquid chromatography (HPLC)-ABTS+ based assay and HPLC-electrospray ionization mass spectrometry analysis of antioxidant phenolic compounds in Salsola komarovii. J Korean Soc Appl Biol Chem 2012;55(2):317–21.
- Ahmad Z, Mehmood S, Fatima I, Malik A, Ifzal R, Afza N, et al. Structural determination of salsolins A and B, new antioxidant polyoxygenated triterpenes from Salsola baryosma, by 1D and 2D NMR spectroscopy. Magn Reson Chem: MRC 2008;46(1):94–8.
- Holm LG, Plucknett DL, Pancho JV, Herberger JP. The world's worst weeds. University Press; 1977.
- Mughal T, Naeem I, Aziz MT, Ahsan A. Antibacterial and Synergistic Studies of Salsola Kali. J App Pharm 2010;1(2):18–26.
- De la Rosa G, Martinez-Martinez A, Pelayo H, Peralta-Videa JR, Sanchez-Salcido B, Gardea-Torresdey JL. Production of low-molecular weight thiols as a response to cadmium uptake by tumbleweed (Salsola kali). Plant Physiol Biochem: PPB/Societe francaise de physiologie vegetale 2005;43(5):491–8.
- Abegaz BM, Woldu Y. Isoflavonoids from the roots of Salsola somalensis. Phytochemistry 1991;30(4):1281–4.
- Sokolowska-Krzaczek A, Skalicka-Wozniak K, Czubkowska K. Variation of phenolic acids from herb and roots of Salsola kali L. Acta Societatis Botanicorum Poloniae 2009;78(3):197–201.
- Ksouri R, Megdiche W, Falleh H, Trabelsi N, Boulaaba M, Smaoui A, et al. Influence of biological, environmental and technical factors on phenolic content and antioxidant activities of Tunisian halophytes. C R Biol 2008;331(11):865–73.
- Hegnauer R. Chemotaxonomie der Pflanzen. III. Dicoyledoneae 1964;1:343.
- Salt TA, Adler JH. Diversity of sterol composition in the family Chenopodiaceae. Lipids 1985;20(9):594–601.
- Richard D, Kefi K, Barbe U, Bausero P, Visioli F. Polyunsaturated fatty acids as antioxidants. Pharmacol Res: Official J Ital Pharmacol Soc 2008;57(6):451–5.
- Kabore A, Tamboura H, Traore A, Traore A, Meda R, Kiendrebeogo M, et al. Phytochemical analysis and acute toxicity of two medicinal plants (Anogeissus leiocarpus and Daniellia oliveri) used in traditional veterinary medicine in Burkina Faso. Arch Appl Sci Res 2010;2(6):47–52.
- Trease G, Evans W. Pharmacognosy. 13th ed. London: English Language Book Society, Bailliere Tindall; 1989.
- Chang C-C, Yang M-H, Wen H-M, Chern J-C. Estimation of total flavonoid content in propolis by two complementary colorimetric methods. J Food Drug Anal 2002;10(3):178–82.
- McDonald S, Prenzler PD, Antolovich M, Robards K. Phenolic content and antioxidant activity of olive extracts. Food Chem 2001;73(1):73–84.
- Oyaizu M. Studies on products of browning reaction: antioxidative activity of products of browning reaction. Jpn J Nutr 1986;44:307–15.
- Nishikimi M, Appaji Rao N, Yagi K. The occurrence of superoxide anion in the reaction of reduced phenazine methosulfate and molecular oxygen. Biochemical Biophys Res Commun 1972;46(2):849–54.
- Litchfield Ja, Wilcoxon F. A simplified method of evaluating dose-effect experiments. J Pharmacol Exp Ther 1949;96(2):99–113.
- Iliskovic N, Singal PK. Lipid lowering: an important factor in preventing adriamycin-induced heart failure. Am Jo Pathol 1997;150(2):727–34.
- Weisshaar D, Gossrau E, Faderl B. Normal ranges of alpha-HBDH, LDH, AP, and LAP as measured with substrate-optimated test charges. Die Medizinische Welt 1975;26(9):387.
- Hørder M, Elser R, Gerhardt W, Mathieu M, Sampson E. IFCC methods for the measurement of catalytic concentration of enzymes. Part 7. IFCC method for creatine kinase (ATP: creatine N-phosphotransferase, EC 2.7. 3.2). Clin Chim Acta 1990;190:S4–17.
- Reitman S, Frankel S. A colorimetric method for the determination of serum glutamic oxalacetic and glutamic pyruvic transaminases. Am J Clin Pathol 1957;28(1):56–63.
- Kei S. Serum lipid peroxide in cerebrovascular disorders determined by a new colorimetric method. Clinica Chimica Acta 1978;90(1):37–43.
- Aebi H. Catalase in vitro. Methods Enzymol 1984;105:121–6.
- Paglia DE, Valentine WN. Studies on the quantitative and qualitative characterization of erythrocyte glutathione peroxidase. J Lab Clin Med 1967;70(1):158–69.
- Habig WH, Pabst MJ, Jakoby WB. Glutathione S-transferases the first enzymatic step in mercapturic acid formation. J Biol Chem 1974;249(22):7130–9.
- Ellman GL. Tissue sulfhydryl groups. Arch Biochem Biophys 1959;82(1):70–7.
- Montgomery H, Dymock J. The determination of nitrite in water. Analyst 1961;86(1023):414–6.
- Lowry OH, Rosenbraugh NI, Farr AL, Randell RJ. Protein Measurement with the Folin Phenol Reagent. J biol Chem 1951;1:193, 265–74.
- Billingham M, Mason J, Bristow M, Daniels J. Anthracycline cardiomyopathy monitored by morphologic changes. Cancer Treat Rep 1978;62(6):865–72.
- L'Ecuyer T, Allebban Z, Thomas R, Vander Heide R. Glutathione S-transferase overexpression protects against anthracycline-induced H9C2 cell death. Am J Physiol Heart Circ Physiol 2004;286(6):2057–64.
- Doroshow JH, Locker GY, Myers CE. Enzymatic defenses of the mouse heart against reactive oxygen metabolites: alterations produced by doxorubicin. J Clin Invest 1980;65(1):128–35.
- Olson HM, Capen CC. Chronic cardiotoxicity of doxorubicin (adriamycin) in the rat: morphologic and biochemical investigations. Toxicol Appl Pharmacol 1978;44(3):605–16.
- El-Shitany NA, El-Haggar S, El-desoky K. Silymarin prevents adriamycin-induced cardiotoxicity and nephrotoxicity in rats. Food Chem Toxicol 2008;46(7):2422–8.
- Abd-Allah AR, Al-Majed AA, Mostafa AM, Al-Shabanah OA, Din AG, Nagi MN. Protective effect of arabic gum against cardiotoxicity induced by doxorubicin in mice: a possible mechanism of protection. J Biochem Mol Toxicol 2002;16(5):254–9.
- Weinstein DM, Mihm MJ, Bauer JA. Cardiac peroxynitrite formation and left ventricular dysfunction following doxorubicin treatment in mice. J Pharmacol Exp Therapeut 2000;294(1):396–401.
- Aldieri E, Bergandi L, Riganti C, Costamagna C, Bosia A, Ghigo D. Doxorubicin induces an increase of nitric oxide synthesis in rat cardiac cells that is inhibited by iron supplementation. Toxicol Appl Pharmacol 2002;185(2):85–90.
- Mohan IK, Kumar KV, Naidu MU, Khan M, Sundaram C. Protective effect of CardiPro against doxorubicin-induced cardiotoxicity in mice. Phytomedicine: International J Phytotherapy Phytopharmacol 2006;13(4):222–9.
- Salvemini F, Franzé A, Iervolino A, Filosa S, Salzano S, Ursini MV. Enhanced glutathione levels and oxidoresistance mediated by increased glucose-6-phosphate dehydrogenase expression. J Biol Chem 1999;274(5):2750–7.
- Jackson JA, Reeves JP, Muntz KH, Kruk D, Prough RA, Willerson JT, et al. Evaluation of free radical effects and catecholamine alterations in adriamycin cardiotoxicity. Am J Pathol 1984;117(1):140–53.
- Boor PJ. Cardiac glutathione: diurnal rhythm and variation in drug-induced cardiomyopathy. Res Commun Chem Pathol Pharmacol 1979;24(1):27–36.
- Mukherjee S, Banerjee SK, Maulik M, Dinda AK, Talwar KK, Maulik SK. Protection against acute adriamycin-induced cardiotoxicity by garlic: role of endogenous antioxidants and inhibition of TNF-alpha expression. BMC Pharmacol 2003;3:16.
- Adachi T, Nagae T, Ito Y, Hirano K, Sugiura M. Relation between cardiotoxic effect of adriamycin and superoxide anion radical. J Pharmacobio-dynam 1983;6(2):114–23.
- Gille L, Nohl H. Analyses of the molecular mechanism of adriamycin-induced cardiotoxicity. Free Radic Biol Med 1997;23(5):775–82.
- Yin X, Wu H, Chen Y, Kang YJ. Induction of antioxidants by adriamycin in mouse heart. Biochem Pharmacol 1998;56(1):87–93.
- Reddy PN, Reddy PS, Rao MR. Studies on the effect of doxorubicin on MDA, NO 2, NO 3, Se-GSH peroxidase and SOD levels in albino rat tissues. Afr J Biotechnol 2007;6(20):2303–2309
- Mitra MS, Donthamsetty S, White B, Latendresse JR, Mehendale HM. Mechanism of protection of moderately diet restricted rats against doxorubicin-induced acute cardiotoxicity. Toxicol Appl Pharmacol 2007;225(1):90–101.
- Van Vleet JF, Greenwood LA, Rebar AH. Effect of selenium-vitamin E on hematologic alterations of adriamycin toxicosis in young pigs. Am J Veterin Res 1981;42(7):1153–9.
- Prahalathan C, Selvakumar E, Varalakshmi P. Lipoic acid ameliorates adriamycin-induced testicular mitochondriopathy. Reprod Toxicol 2005;20(1):111–6.
- Bourgou S, Ksouri R, Bellila A, Skandrani I, Falleh H, Marzouk B. Phenolic composition and biological activities of Tunisian Nigella sativa L. shoots and roots. Comptes Rendus Biologies 2008;331(1):48–55.
- Jaleel CA, Gopi R, Manivannan P, Panneerselvam R. Antioxidative potentials as a protective mechanism in Catharanthus roseus (L.) G. Don. plants under salinity stress. Turk J Bot 2007;31:245–51.
- Navarro JM, Flores P, Garrido C, Martinez V. Changes in the contents of antioxidant compounds in pepper fruits at different ripening stages, as affected by salinity. Food Chem 2006;96(1):66–73.
- Sadzuka Y, Sugiyama T, Shimoi K, Kinae N, Hirota S. Protective effect of flavonoids on doxorubicin-induced cardiotoxicity. Toxicol Lett 1997;92(1):1–7.