Abstract
Objectives
Moderate exercise is known to have health benefits, while both sedentarism and strenuous exercise have pro-oxidant effects. In this study, we assessed the effect of moderate exercise on the antioxidant homeostasis of rats’ hippocampi.
Methods
Female Wistar rats were submitted to a 30-minute swimming protocol on 5 days a week, for 4 weeks. Control rats were immersed in water and carefully dried. Production of hippocampal reactive species, activity of antioxidant enzymes, and glutathione levels in these animals were determined up to 30 days after completion of the 4-week protocol.
Results
Production of reactive species and hippocampal glutathione levels were increased 1 day after completion of the 4-week protocol, and returned to control levels after 7 days. Antioxidant enzyme activities were increased both 1 day (catalase) and 7 days (superoxide dismutase and glutathione peroxidase) after completion of the protocol. Thirty days after completion of the protocol, none of the antioxidant parameters evaluated differed from those of controls.
Discussion
Our results reinforce the benefits of aerobic exercise, which include positive modulation of antioxidant homeostasis in the hippocampi. The effects of exercise are not permanent; rather, an exercise regimen must be continued in order to maintain the neurometabolic adaptations.
Introduction
Moderate-intensity physical exercise has clear health benefits, improving quality of life, reducing the risk of death, and preventing the development of chronic oxidative-stress-associated conditions such as obesity, type-II diabetes, cardiovascular dysfunction, neurodegenerative diseases, depression, and cancer.Citation1,Citation2 The dose of exercise may define its metabolic effects; moderate exercise appears to induce beneficial metabolic adaptations, while sedentarism and strenuous exercise appear to increase the risk of infection and exacerbate oxidative damage.Citation3,Citation4 This is not clear-cut, however, with recent reports showing potential benefits from high-intensity exercise.Citation5–Citation7 We still do not know the optimum dose of aerobic exercise, although many studies have attempted to define it. Today, moderate exercise for 150 minutes/week, performed on most days of the week, is considered adequate to maintain health.Citation8,Citation9
In an earlier rat study, the benefits of exercise seemed to be restricted to animals that maintained activity, as brain adaptations in response to exercise were lost 8 weeks after the end of the exercise protocol.Citation10 Some of the benefits experienced by the exercised animals appeared to be related to the brain's redox status. In this context, the increased activities of antioxidant and damage-repair enzymes are probably mediated by the initial production of reactive species.Citation4,Citation10–Citation12 These findings suggest an antioxidant effect mediated by regular moderate aerobic exercise, resembling the characteristics of hormesis, responding to the ‘stressor’ with a metabolic adaptation.Citation4,Citation12,Citation13
Oxidative stress was first defined by Sies et al.Citation14 as an unbalance between the oxidant and antioxidant pools, resulting in an oxidative environment capable of damaging cellular components such as lipids, proteins, and nucleic acids.Citation14,Citation15 More recently, JonesCitation16 redefined the term ‘oxidative stress’ as a disturbance of redox signaling and control, in which the ratio of reduced glutathione (GSH) to oxidized glutathione is crucial in determining the redox status. The brain is particularly sensitive to oxidative stress, considering its high content of self-oxidizable neurotransmitters, glutamate, and iron, in addition to membranes enriched in polyunsaturated fatty acids, and the high demand for ATP, which increases the activity of the respiratory chain.Citation15 In this sense, aerobic exercise increases free-radical generation mainly via the mitochondrial respiratory chain, xanthine oxidase activity, and phagocyte activation.Citation8,Citation17,Citation18 The adaptive response elicited by exercise is dependent on the production of reactive species, which is followed by increased numbers of mitochondria, improved antioxidant status, and the altered expression of a large number of sensitive genes.Citation17
In this study, we investigated the effect of swimming exercise on oxidative and antioxidant parameters in the hippocampi of female Wistar rats. The rats were trained for 4 weeks and euthanized up to 30 days after the last training session in order to verify the persistence of neurometabolic adaptations.
Experimental procedures
Animals and reagents
A total of 70 female Wistar rats (90 days old) were obtained from the Central Animal House of Departamento de Bioquímica, Instituto de Ciências Básicas da Saúde, Universidade Federal do Rio Grande do Sul, Porto Alegre, RS, Brazil. Rats were divided into the following groups: control euthanized 1 day after swimming (14 animals), exercised euthanized 1 day after swimming (14 animals), control euthanized 7 days after swimming (14 animals), exercised euthanized 7 days after swimming (14 animals), control euthanized 30 days after swimming (7 animals), exercised euthanized 30 days after swimming (7 animals). The animals were maintained on a 12/12-hour light/dark cycle in an air-conditioned colony room at a constant temperature (22 ± 1°C). They had free access to water and a 20% (w/w) protein commercial chow.
The experiments were approved by the local animal ethics commission (Comissão de Ética no Uso de Animais/Universidade Federal do Rio Grande do Sul – CEUA/UFRGS) under the number 19481, and national animal rights regulations (Law 11.794/2008), the National Institutes of Health Guide for the Care and Use of Laboratory Animals (NIH publication No. 80-23, revised 1996), and Directive 2010/63/EU were followed. We further attest that all efforts were made to minimize the number of animals used and their suffering.
All chemicals were obtained from Sigma Chemical Co., St Louis, MO, USA.
Swimming exercise protocol
The experimental design is presented in . The rats were divided into control and exercised groups. In the exercised group, rats were submitted to swimming in a pool filled with 32 ± 1°C water on 5 days/week for 4 weeks. Each swimming session lasted for 30 minutes, and always took place between 9 and 12 a.m. Each rat was isolated for the swim, which was conducted using an apparatus designed specifically for rat swimming. Within this apparatus, each room measures 30 × 30 × 90 cm (width × length × depth), preventing the animals from touching the bottom of the tank. The animals were left free to swim, without any extra weight, and were gently stimulated to swimming. This protocol has moderate intensity.Citation19 Control rats were immersed in water, carefully dried, and returned to the housing boxes.
Biochemical assays
Sample preparation
The rats were euthanized 1, 7, or 30 days after accomplishment of the 4-week exercise protocol. Following euthanasia, the brain was isolated and the hippocampus was dissected in a Petri dish on ice. The tissue was homogenized in 10 volumes (1 : 10, w/v) of phosphate-buffered saline pH 7.4, containing 1 mM phenylmethylsulfonyl fluoride and 1 mM ethylene glycol tetra-acetic acid. The homogenates used were from individual animals; samples were never pooled. Homogenates were centrifuged at 1000g for 10 minutes at 4°C, and nuclei and cell debris were discarded. The pellet was discarded and the supernatant was taken for biochemical assays.
Production of reactive species
Production of reactive species was assessed using the dichlorofluorescein (DCF) oxidation method.Citation20 Briefly, 60 μl of biologic sample was incubated at 37°C, in the dark, for 30 minutes, with the addition of 240 μl 2′,7′-dichlorofluorescein diacetate (H2DCFDA) in a 96-well plate. H2DCFDA is cleaved by cellular esterases to form H2DCF. This is oxidized by reactive oxygen/nitrogen species present in the sample, producing a fluorescent compound, DCF. DCF oxidation was measured fluorimetrically using a wavelength of 488 nm excitation and 525 nm emission. A standard curve, using standard DCF (0.25–10 mM), was performed in parallel with the samples. Results were calculated as nmol/mg protein.
Activity of antioxidant enzymes
Superoxide dismutase (SOD) (EC 1.15.1.1) activity was evaluated by quantifying the inhibition superoxide-dependent autoxidation of epinephrine, verifying the absorbance of the samples at 480 nm.Citation21 Considering the protocol used in sample preparation, we measured total SOD activity, expressed as the amount of enzyme that inhibits the oxidation of epinephrine by 50%, which is equal to 1 unit. The data were calculated as units/mg protein.
Catalase (CAT) (EC 1.11.1.6) activity was assayed according to Aebi,Citation22 by measuring the absorbance decrease at 240 nm in a reaction medium containing 20 mM H2O2, 0.1% Triton X-100, and 10 mM potassium phosphate buffer (pH 7.0). One CAT unit is defined as 1 μmol H2O2 consumed per minute, with the specific activity calculated as units/mg protein.
Glutathione peroxidase (GPx) (EC 1.11.1.9) activity was measured according to the method described by Wendel,Citation23 using tert-butyl hydroperoxide as the substrate. Nicotinamide adenine dinucleotide phosphate (NADPH) disappearance was monitored spectrophotometrically at 340 nm in a medium containing 2 mM GSH, 0.15 U/ml glutathione reductase (EC 1.8.1.7), 0.4 mM azide, 0.5 mM tert-butyl hydroperoxide, and 0.1 mM NADPH. One GPx unit is defined as 1 μmol NADPH consumed per minute, with the specific activity calculated as units/mg protein.
Glutathione levels
GSH concentration was measured according to Browne and Armstrong,Citation24 where GSH reacts with the fluorophore o-phthalaldehyde. The proteins in supernatant were initially precipitated with meta-phosphoric acid (1 : 1, v:v), and centrifuged at 5.000g for 10 minutes at 25°C. A total of 50 μl supernatant was incubated at room temperature for 15 minutes with 15 μl of 7.5 mM ο-phthaldialdehyde and 235 μl of 120 mM sodium phosphate buffer (pH 8.0) containing 5 mM ethylene diamine tetraacetic acid. A blank sample was run in parallel. Fluorescence was measured using excitation and emission wavelengths of 350 and 420 nm, respectively. A calibration curve was prepared with standard GSH (0.001–1 mM) and the concentrations were calculated as nmol/mg protein.
Protein concentration assay
Protein concentration was measured following the method of Lowry et al.,Citation25 using bovine serum albumin as the standard.
Statistical analysis
Data were analyzed using Student's t-test, with GraphPad Prism 6.0 software. Data were considered statistically significant at P < 0.05.
Results
Swimming exercise increased hippocampal production of reactive species 1 day after protocol completion
shows DCF oxidation in the hippocampi of rats up to 7 days after completion of the 4-week, moderate-intensity exercise protocol. Reactive species were increased 1 day after the last swimming session (t(14) = 4.323, P < 0.001). Seven days after completion of the protocol, however, DCF oxidation had returned to control levels (t(10) = 1.442, P > 0.05).
Swimming exercise enhanced hippocampal antioxidant status up to 7 days after training
Adaptation of the antioxidant system may be the result of an increase in reactive species synthesis resulting from exercise-induced, mild oxidative stress. In this context, we evaluated the activities of SOD, CAT, and GPx in the hippocampi of female rats trained for 4 weeks in a swimming pool.
As shown in , all of the antioxidant enzymes studied were positively modulated 1 or 7 days after completion of the 4-week exercise protocol. CAT was the first enzyme altered, demonstrating increased activity 1 day after completion of the protocol (t(12) = 2.776, P < 0.05) and a return to normal levels at 7 days (t(10) = 0.3833, P > 0.05). Surprisingly, SOD and GPx were not altered 1 day after completion of the training (SOD: t(16) = 0.1194, P > 0.05; GPx: t(12) = 2.141, P > 0.05). However, both showed significantly increased activity at 7 days (SOD: t(10) = 2.434, P < 0.05; GPx t(12) = 4.514, P < 0.001). Both SOD and GPx had returned to control levels at 30 days after the end of exercise protocol (SOD: t(9) = 0.1996, P > 0.05; GPx: t(8) = 1.979, P > 0.05; ).
Figure 3. Effect of exercise on (A) superoxide dismutase, (B) catalase, and (C) glutathione peroxidase activity in hippocampi of rats, evaluated 1 and 7 days after the end of a 4-week swimming protocol. Results are expressed as mean + SEM for n = 4–9. *P < 0.05; ***P < 0.001 versus corresponding control (Student's t-test). CAT, catalase; GPx, glutathione peroxidase; SOD, superoxide dismutase.
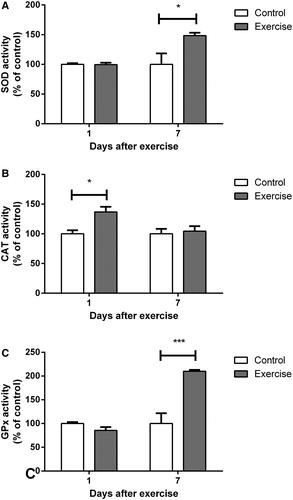
Table 1. Effect of swimming detraining on antioxidant parameters in hippocampi of rats, 30 days after the last exercise session
We also evaluated the levels of GSH, the most important endogenous non-enzymatic antioxidant defense. As shown in , hippocampal GSH content showed a significant increase 1 day after completion of the training protocol (t(16) = 2.666, P < 0.05), returning to normal levels at 7 days (t(12) = 0.1912, P > 0.05). Thirty days after the end of the exercise program, GSH levels were similar to control levels (t(9) = 1.308, P > 0.05; ).
Discussion
Moderate aerobic exercise (30 minutes’ swimming per session, five times per week, for 4 weeks) was associated with a relevant improvement in hippocampal antioxidant status up to 7 days after the end of the exercise protocol, probably as a result of increased levels of reactive species.
Our results showed increased DCF oxidation 1 day after the last swimming session. In addition, hippocampal antioxidant defenses were increased 1 day after exercise, as shown by increases in GSH levels and CAT activity. SOD and GPx activity was significantly increased 7 days after completion of the exercise program, while DCF oxidation, GSH levels, and CAT activity had returned to control levels at this time. We also assessed lipid peroxidation in the hippocampi of exercised rats (data not shown); however, this was not modified by the swimming protocol, suggesting that cell injury did not occur. Of note, this study used only female rats in order to verify whether the benefits of exercise that have been widely reported in males are also found in females.
Although it is difficult to compare exercise studies in the literature, due to a variety of protocols, intensities, and runtimes, our findings are extensively supported by published works, which have reported that brain redox status is positively modulated by exercise, without affecting cell damage parameters. The maximum velocity of CAT and GPx has previously been reported to be increased in the brains of exercised rats.Citation26 Similar results were found by Devi and Kiran,Citation27 who evaluated CAT, SOD, and GPx in the hippocampi of young, middle-aged, and old rats.Citation13 In addition, Marosi et al.Citation28 reported the up-regulation of SOD and GPx in the hippocampi of female rats exercised on a treadmill for 15 weeks, probably mediated by signaling pathways coordinated by 5′-AMP-activated protein kinase (AMPK) and peroxisome proliferator-activated receptor gamma coactivator 1-alpha (PGC-1α), although reduced levels of reactive oxygen species were found. In agreement with our results, others have reported that exercise not only affects antioxidant enzymes, but is also associated with an increase in GSH levels.Citation29 It has been proposed that an augmented resistance to oxidative stress, via up-regulation of the antioxidant network, is a consequence of reactive oxygen/nitrogen species produced by aerobic exercise.Citation3
With respect to the oxidative effects of aerobic exercise, Turgut et al.Citation30 reported that a single session of swimming did not induce lipid peroxidation in the brains of exercised rats, even though the liver and heart were affected. Even a more extended swimming protocol, performed for 1 hour a day, 5 days a week, for 9 weeks, did not alter cerebral lipid and DNA oxidation, assayed by thiobarbituric acid reactive species and 8-OH-2′-deoxyguanosine measures, although carbonyl levels decreased.Citation11 Goto et al.,Citation12 using an intense exercise protocol of 2 hours swimming each day for 8 weeks, reported similar results, correlating the decline in oxidative stress with better cognitive function in rats. Exercise was associated with improved learning and memory in a passive avoidance task, allied with increased hippocampal brain-derived neurotrophic factor and decreased free-radical levels in the cerebellum.Citation12 The effects on memory were not preserved after 8 weeks of detraining, which was associated with reduced neurotrophins in the hippocampus and reduced cerebellar free-radical concentrations.Citation10 These results demonstrate the dual effect of exercise, which follows the hormesis model; while sedentarism or a single bout of exercise does not alter the oxidative status, moderate-intensity exercise promotes an increased antioxidant response.
It is well accepted that reactive species up-regulate antioxidant enzymes, largely by modulating transcription. In this respect, exercise activates cAMP response element-binding protein, mitogen-activated protein kinases, and synapsin,Citation31,Citation32 and increases phosphorylation of AKT in the brain.Citation33 Muscle metabolic adaptations are more thoroughly understood.Citation13,Citation17,Citation34,Citation35 Myocytes react to exercise-induced increases in reactive species, increasing the expression of SOD, CAT, and GPx in a mechanism that is dependent on nuclear factor κB,Citation17,Citation36,Citation37 nuclear erythroid 2 p45-related factor 2,Citation34 and PGC-1α.Citation35,Citation38–Citation41 Recently, Marosi et al.Citation28 offered evidence to support this assumption, showing that moderate-intensity aerobic exercise increases AMPK phosphorylation and PGC-1α activity, probably in response to SOD and GPx up-regulation in the hippocampus.
In conclusion, moderate-intensity swimming exercise promotes hippocampal metabolic adaptation, improving antioxidant status by up-regulating antioxidant enzyme activity and GSH levels up to 7 days after exercise, probably in response to exercise-induced increased levels of reactive species. Our data also provide significant evidence that exercise-induced antioxidant adaptations are rapidly lost, with continued training required to maintain the neurometabolic adaptations.
Disclaimer statements
Contributors The study was designed by Cristiane Matté; data were collected by Karen Y. Kudo, Vinícius Stone, Thiago B. Marcelino, and Pauline M. August; and were analyzed and interpreted by all authors. The article was drafted by Cristiane Matté, and was revised and approved for publishing by all authors.
Funding This work was supported by grants from Pró-Reitoria de Pesquisa/Universidade Federal do Rio Grande do Sul (PROPESQ/UFRGS).
Conflicts of interest None.
Ethics approval All the experiments were approved by a local Animal Ethics Commission (Comissão de Ética no Uso de Animais/Universidade Federal do Rio Grande do Sul – CEUA/UFRGS) under the number 19481, and followed national animal rights regulation (Law 11.794/2008), the National Institute of Health Guide for the Care and Use of Laboratory Animals (NIH publication No. 80-23, revised 1996), and the Directive 2010/63/EU. We further attest that all efforts were made to minimize the number of animals used and their suffering.
Acknowledgements
This work was supported by grants from Pró-Reitoria de Pesquisa/Universidade Federal do Rio Grande do Sul (PROPESQ/UFRGS).
References
- Powell KE, Paluch AE, Blair SN. Physical activity for health: what kind? How much? How intense? On top of what? Annu Rev Public Health 2011;32:349–65.
- Wolff E, Gaudlitz K, von Lindenberger BL, Plag J, Heinz A, Strohle A. Exercise and physical activity in mental disorders. Eur Arch Psychiatry Clin Neurosci 2011;261( Suppl. 2):S186–91.
- Radak Z, Taylor AW, Ohno H, Goto S. Adaptation to exercise-induced oxidative stress: from muscle to brain. Exerc Immunol Rev 2001;7:90–107.
- Radak Z, Chung HY, Koltai E, Taylor AW, Goto S. Exercise, oxidative stress and hormesis. Ageing Res Rev 2008;7(1):34–42.
- Lezi E, Burns JM, Swerdlow RH. Effect of high-intensity exercise on aged mouse brain mitochondria, neurogenesis, and inflammation. Neurobiology of Aging 2014;35(11):2574–83.
- Rojas Vega S, Knicker A, Hollmann W, Bloch W, Struder HK. Effect of resistance exercise on serum levels of growth factors in humans. Horm Metab Res 2010;42(13):982–6.
- de Almeida AA, Gomes da Silva S, Fernandes J, Peixinho-Pena LF, Scorza FA, Cavalheiro EA, et al. Differential effects of exercise intensities in hippocampal BDNF, inflammatory cytokines and cell proliferation in rats during the postnatal brain development. Neurosci Lett 2013;553:1–6.
- Vina J, Sanchis-Gomar F, Martinez-Bello V, Gomez-Cabrera MC. Exercise acts as a drug; the pharmacological benefits of exercise. Br J Pharmacol 2012;167(1):1–12.
- O'Donovan G, Blazevich AJ, Boreham C, Cooper AR, Crank H, Ekelund U, et al. The ABC of physical activity for health: a consensus statement from the British Association of Sport and Exercise Sciences. J Sports Sci 2010;28(6):573–91.
- Radak Z, Toldy A, Szabo Z, Siamilis S, Nyakas C, Silye G, et al. The effects of training and detraining on memory, neurotrophins and oxidative stress markers in rat brain. Neurochem Int 2006;49(4):387–92.
- Radak Z, Kaneko T, Tahara S, Nakamoto H, Pucsok J, Sasvari M, et al. Regular exercise improves cognitive function and decreases oxidative damage in rat brain. Neurochem Int 2001;38(1):17–23.
- Goto S, Naito H, Kaneko T, Chung HY, Radak Z. Hormetic effects of regular exercise in aging: correlation with oxidative stress. Appl Physiol Nutr Metab 2007;32(5):948–53.
- Radak Z, Chung HY, Goto S. Systemic adaptation to oxidative challenge induced by regular exercise. Free Radic Biol Med 2008;44(2):153–9.
- Sies H, Cadenas E, Symons MCR, Scott G. Oxidative stress – damage to intact-cells and organs. Philos T Roy Soc B 1985;311(1152):617–31.
- Halliwell B, Gutteridge JMC. Free radicals in biology and medicine. New York: Oxford University; 2007.
- Jones DP. Redefining oxidative stress. Antioxid Redox Signal 2006;8(9–10):1865–79.
- Sachdev S, Davies KJ. Production, detection, and adaptive responses to free radicals in exercise. Free Radic Biol Med 2008;44(2):215–23.
- Gomez-Cabrera MC, Sanchis-Gomar F, Garcia-Valles R, Pareja-Galeano H, Gambini J, Borras C, et al. Mitochondria as sources and targets of damage in cellular aging. Clin Chem Lab Med 2012;50(8):1287–95.
- Marcelino TB, Longoni A, Kudo KY, Stone V, Reck A, de Assis A, et al. Evidences that maternal swimming exercise improves antioxidant defenses and induces mitochondrial biogenesis in brain of young Wistar rats. Neuroscience 2013, 246:28–39.
- LeBel CP, Ischiropoulos H, Bondy SC. Evaluation of the probe 2′,7′-dichlorofluorescin as an indicator of reactive oxygen species formation and oxidative stress. Chem Res Toxicol 1992;5(2):227–31.
- Misra HP, Fridovich I. The role of superoxide anion in the autoxidation of epinephrine and a simple assay for superoxide dismutase. J Biol Chem 1972;247(10):3170–5.
- Aebi H. Catalase in vitro. Methods Enzymol 1984;105:121–6.
- Wendel A. Glutathione peroxidase. Methods Enzymol 1981;77:325–33.
- Browne RW, Armstrong D. Reduced glutathione and glutathione disulfide. Methods Mol Biol 1998;108:347–52.
- Lowry OH, Rosebrough NJ, Farr AL, Randall RJ. Protein measurement with the Folin phenol reagent. J Biol Chem 1951;193(1):265–75.
- Somani SM, Husain K. Exercise training alters kinetics of antioxidant enzymes in rat tissues. Biochem Mol Biol Int 1996;38(3):587–95.
- Devi SA, Kiran TR. Regional responses in antioxidant system to exercise training and dietary vitamin E in aging rat brain. Neurobiol Aging 2004;25(4):501–8.
- Marosi K, Bori Z, Hart N, Sarga L, Koltai E, Radak Z, et al. Long-term exercise treatment reduces oxidative stress in the hippocampus of aging rats. Neuroscience 2012;226:21–8.
- Santin K, da Rocha RF, Cechetti F, Quincozes-Santos A, de Souza DF, Nardin P, et al. Moderate exercise training and chronic caloric restriction modulate redox status in rat hippocampus. Brain Res 2011;1421:1–10.
- Turgut G, Demir S, Genc O, Karabulut I, Akalin N. The effect of swimming exercise on lipid peroxidation in the rat brain, liver and heart. Acta Physiol Pharmacol Bulg 2003;27(2–3):43–5.
- Vaynman S, Ying Z, Gomez-Pinilla F. Hippocampal BDNF mediates the efficacy of exercise on synaptic plasticity and cognition. Eur J Neurosci 2004;20(10):2580–90.
- Molteni R, Wu A, Vaynman S, Ying Z, Barnard RJ, Gomez-Pinilla F. Exercise reverses the harmful effects of consumption of a high-fat diet on synaptic and behavioral plasticity associated to the action of brain-derived neurotrophic factor. Neuroscience 2004;123(2):429–40.
- Seo TB, Cho HS, Shin MS, Kim CJ, Ji ES, Baek SS. Treadmill exercise improves behavioral outcomes and spatial learning memory through up-regulation of reelin signaling pathway in autistic rats. J Exerc Rehab 2013;9(2):220–9.
- Muthusamy VR, Kannan S, Sadhaasivam K, Gounder SS, Davidson CJ, Boeheme C, et al. Acute exercise stress activates Nrf2/ARE signaling and promotes antioxidant mechanisms in the myocardium. Free Radic Biol Med 2012;52(2):366–76.
- Gomes EC, Silva AN, de Oliveira MR. Oxidants, antioxidants, and the beneficial roles of exercise-induced production of reactive species. Oxid Med Cell Longev 2012;2012:756132.
- Gomez-Cabrera MC, Domenech E, Vina J. Moderate exercise is an antioxidant: upregulation of antioxidant genes by training. Free Radic Biol Med 2008;44(2):126–31.
- Ji LL, Gomez-Cabrera MC, Vina J. Role of nuclear factor kappaB and mitogen-activated protein kinase signaling in exercise-induced antioxidant enzyme adaptation. Appl Physiol Nutr Metab 2007;32(5):930–5.
- Jackson MJ. Redox regulation of adaptive responses in skeletal muscle to contractile activity. Free Radic Biol Med 2009;47(9):1267–75.
- Derbre F, Gomez-Cabrera MC, Nascimento AL, Sanchis-Gomar F, Martinez-Bello VE, Tresguerres JA, et al. Age associated low mitochondrial biogenesis may be explained by lack of response of PGC-1alpha to exercise training. Age (Dordr) 2012;34(3):669–79.
- Zhang Q, Wu Y, Zhang P, Sha H, Jia J, Hu Y, et al. Exercise induces mitochondrial biogenesis after brain ischemia in rats. Neuroscience 2012;205:10–7.
- Gomez-Cabrera MC, Domenech E, Romagnoli M, Arduini A, Borras C, Pallardo FV, et al. Oral administration of vitamin C decreases muscle mitochondrial biogenesis and hampers training-induced adaptations in endurance performance. Am J Clin Nutr 2008;87(1):142–9.