Abstract
Objectives
The aim of the study was to test a novel method for assessing oxidative stress, the RedoxSYS™ diagnostic system, a holistic, fast, minimally invasive, and requiring small sample volume method, that measures two parameters, the static (sORP) and the capacity (cORP) oxidation-reduction potential.
Methods
The redox status of 14 athletes participating in a mountain marathon race was assessed. Redox status in blood obtained 1 day before the race and immediately after the race was assessed using the RedoxSYS diagnostic system as well as conventional oxidative stress markers such as glutathione levels (GSH), catalase activity (CAT), thiobarbituric acid reactive substances (TBARS), protein carbonyls (CARB), and total antioxidant activity.
Results
The results showed that after the race, the sORP was increased significantly by 7% indicating oxidative stress induction, while cORP was decreased by 14.6% but not significantly. Moreover, the conventional oxidative stress markers GSH and CAT were decreased significantly by 13.1 and 23.4%, respectively, while TBARS and CARB were increased significantly by 26.1 and 15.6%, respectively, after the race indicating oxidative stress induction.
Discussion
The present study demonstrated for the first time that the RedoxSYS diagnostic system can be used for evaluating the exercise-induced oxidative stress in athletes.
Introduction
Reactive oxygen species (ROS) are products of normal metabolism.Citation1 ROS include free radicals such as superoxide radical , hydroxyl radical
, peroxyl radical
as well as non-radical species such as hydrogen peroxide (H2O2).Citation1 Endogenous sources of free radicals include the mitochondrial respiratory chain, inflammation, peroxisomes, and the cytochrome P450.Citation2 Some ROS are necessary for physiological processes including phagocytosis, intracellular signaling, cell proliferation, metabolism, apoptosis, and muscle contraction.Citation1,Citation3,Citation4 However, excessive production of ROS can lead to oxidative stress, a pathophysiological condition, resulting in oxidative damage of macromolecules (e.g. lipids, proteins, and DNA), immune dysfunction, muscle damage, and fatigue.Citation1,Citation5–Citation8 Thus, oxidative stress may be a causal factor for several pathophysiological conditions and diseases.Citation1
A number of oxidative stress biomarkers have been developed for assessing oxidative stress levels in humans. These biomarkers help to assess the redox status of a person and consequently predict the possible manifestation of a pathological condition or the progress of an already present disease.Citation9 However, oxidative stress measurement is still incomplete, time consuming and impractical in a clinical setting, and so there is a great need for developing new methods for assessing the redox status in humans.Citation10 The aim of the present study was to test a novel method for assessing oxidative stress, the Luoxis’ proprietary RedoxSYS diagnostic system. RedoxSYS diagnostic system measures oxidation-reduction potential (ORP) which is an integrated measure of the balance between total oxidants (e.g. oxidized thiols, superoxide radical, hydroxyl radical, hydrogen peroxide, nitric oxide, peroxynitrite, and transition metal ions), and total reductants (e.g. free thiols, ascorbate, a-tocopherol, b-carotene, and uric acid) as well as unknown participants. Thus, ORP is an overall measure of the oxidative stress to which a biological system is subjected.Citation11 The RedoxSYS diagnostic system enables robust and rapid assessment of oxidative stress in a single drop of plasma via measurement within four minutes of two distinct elements to determine ORP, the static ORP (sORP), and the capacity ORP (cORP). sORP, the classically termed ORP, is the standard potential between a working electrode and a reference electrode with no driving current (or extremely small current) which is proportional to the balance of reductants and oxidants. Low sORP values mean that the biological sample is in the normal range of oxidative stress, while higher than normal sORP values means that the biological sample is in a higher state of oxidative stress. cORP is the measure of antioxidant reserve available in the body's system; high cORP values mean that the biological sample has antioxidant reserves in the normal range; lower than normal cORP values means that the biological sample has below normal antioxidant reserves. Specifically, the RedoxSYS diagnostic system measures the ORP with a three electrode system – a working electrode, a counter electrode and a reference electrode. First, a negligible amount of current is applied between the working and counter electrodes, and the ORP is measured between the working and reference electrodes. Once the ORP reading reaches equilibrium, the sORP is established and measured in millivolts (mV). Then, a linearly increasing current is applied to the sample, between the counter and working electrodes. The time from the beginning of the current sweep to the maximum rate of change in ORP is referred to as transition time and the integrated current to this time is the cORP, measured in microcoulombs (µC). Moreover, this method is also adapted specifically to plasma whereas traditional electrodes are geared for simpler solutions.
In particular, in the present study, the RedoxSYS diagnostic system was used to assess the redox status of athletes participating in a mountain marathon race. The mountain marathon race was the Faethon Olympus Marathon, an annual mountain run race of 44 km taking place in the mountain of Olympus in Greece. This race consists of continuous and prolonged exercise that involves 3600 m elevation gain and 3600 m elevation loss, a fact that increases the degree of difficulty of the race and the muscle damage of the athletes. In addition, more than 25 km of the race take place at an altitude of 2000 m or more.
It is well established that excessive exercise induces production of ROS resulting in oxidative stress that may cause muscle damage, fatigue, oxidative damage of macromolecules and immune dysfunction.Citation6,Citation7,Citation12,Citation13 The main sources of ROS generated during strenuous exercise is the leakage of electrons from mitochondrial electron transport chain, activated neutrophils, macrophages recruited to repair damaged tissues, alterations of prostanoid and catecholamine metabolism, and changes in the activity of the enzymes xanthine oxidase and nicotinamide adenine dinucleotide phosphate oxidase.Citation14,Citation15 Several studies have exhibited that ultra-endurance exercise such as marathon race increases oxidative stress as shown by increase in lipid peroxidation, oxidative DNA damage, sensitivity of low density lipoprotein to oxidation, production of ROS by phagocytes and protein oxidation, and by decrease in antioxidant enzymes (e.g. superoxide dismutase) and antioxidant molecule levels (e.g. glutathione (GSH)). These detrimental effects induced by marathon race are in the most cases higher compared to exercise of shorter duration.Citation16–Citation21 Thus a marathon race could be considered a good model of exercise-induced oxidative stress. Since strenuous exercise such as marathon race induces oxidative stress that may lead to pathological conditions, it would be useful if we could monitor the levels of oxidative stress after exercise, so as to make the appropriate interventions (e.g. antioxidant supplementation) for reducing its adverse effects. Moreover, the benefit of dietary intake of antioxidants during exercise is controversial. The reason is that exercise-induced oxidative stress play important role in the improvement of metabolic adaptations, but also it may cause inflammation and finally damage cellular components.Citation6,Citation7,Citation12,Citation13,Citation22–Citation24 Thus, the future development and evaluation of markers for determining the optimal oxidative stress level after exercise are necessary.Citation24
Apart from using the RedoxSYS diagnostic system, the redox status of the athletes was assessed by ‘conventional’ oxidative stress markers that are used widely such as GSH, catalase activity, thiobarbituric acid reactive substances (TBARS), protein carbonyls (CARB), and total antioxidant capacity (TAC). Moreover, since intensive exercise-induced oxidative stress causes inflammation, the inflammation biomarker C-reactive protein (CRP) was also measured.Citation25 ORP values were compared and correlated with those of the other oxidative stress markers in order to examine the validity and the sensitivity of the RedoxSYS diagnostic system for assessing oxidative stress, particularly as induced by strenuous exercise.
Material and methods
Subjects
Fourteen mountain marathon runners, 11 men, and 3 women (age, 39.3 ± 3 year; height, 174 ± 2 cm; weight, 70.5 ± 2.3 kg; body mass index, 23.2 ± 0.6 kg/m2; mean ± SEM) participated in this study. A written informed consent to participate in the study was provided by all participants. The procedures were in accordance with the Helsinki declaration of 1975 as revised in 2000 and approval was received by the human subjects committee of the University of Thessaly.
The subjects trained at least three times per week for at least 3 hours and had a training history of at least 3 years. They were nonsmokers and were not receiving anti-inflammatory medication or nutritional supplements. Moreover, all runners participating in the Faethon Olympus Marathon race were healthy, endurance-trained athletes, fully familiarized with mountain running, since the prerequisite of participation includes prior participation in one other mountain marathon race of over 40 km.
The subjects visited the Hospital of Elassona located close to the starting point of the race for screening of anthropometric parameters and they completed a health and activity questionnaire. Body mass was measured to the nearest 0.5 kg (Beam Balance 710, Seca, UK) with the subjects lightly dressed and barefoot. Standing height was measured to the nearest 0.5 cm (Stadiometer 208, Seca). Body mass index was calculated as the ratio of bodyweight (kg)/height (m).
During the race, the athletes consumed fluids (i.e. water, beverages, and sports drinks) and carbohydrate-rich food (i.e. bread, fruits, cookies, and pasta) available at 10 checkpoints throughout the race ad libitum. The mean running time of the athletes was 9 hours and 59 ± 27 minutes.
Blood collection and handling
Blood was drawn at two different time points, at pre-race and immediately post-race. Blood samples (10 ml) were drawn from a forearm vein with subjects in a seated position. Blood was collected in ethylenediamine tetraacetic acid tubes for measuring TAC, TBARS, CARB, GSH, and CRP. Blood was also collected in heparin tubes for measuring ORP. Blood samples were centrifuged immediately at 1370 g for 10 minutes at 4°C and the plasma was collected and used for the above measurements. The packed erythrocytes were lysed with distilled water (1:1 v/v), inverted vigorously, centrifuged at 4020 g for 15 minutes at 4°C and the erythrocyte lysate was collected for measurement of catalase activity. A portion of erythrocyte lysate (500 µl) was treated with 5% trichloroacetic acid (TCA) (1:1 v/v), vortexed vigorously, and centrifuged at 28 000 g for 5 minutes at 4°C. The supernatants were removed, treated again with 5% TCA (1.3:1 v/v), and centrifuged again at 28 000 g for 5 minutes at 4°C. The clear supernatants were transferred to eppendorf tubes and were used for the determination of GSH. Plasma and erythrocyte lysate were stored at 80°C prior to biochemical analyses.
Assessment of sORP and cORP using the RedoxSYS diagnostic system
sORP and cORP values were determined using the RedoxSYS diagnostic system (Luoxis Diagnostics, Inc., Englewood, CO, USA). In particular, 20 µl of plasma was applied to disposable sensors designed by Luoxis, which were inserted into the RedoxSYS diagnostic system that measured and reported within 4 minutes the sORP and cORP values. sORP captures the integrated balance of oxidants and reductants in a specimen and is reported in millivolts (mV). cORP is the amount of antioxidant reserves and is expressed in microcoulombs (µC).
Assessment of TAC, TBARS, GSH, catalase activity, and CARB
For TBARS determination, a slightly modified assay of Keles et al. was used.Citation26 TBARS is one of the most frequently used methods for lipid peroxidation, although it has been criticized for a potential lack of specificity.Citation27 According to this method, 100 µl of plasma was mixed with 500 µl of 35% TCA and 500 µl of trishydroxymethylaminomethane hydrochloride (Tris–HCl) (200 mM, pH 7.4) and incubated for 10 minutes at room temperature. One milliliter of 2 M sodium sulfate (Na2SO4) and 55 mM thiobarbituric acid (TBA) solution was added and the samples were incubated at 95°C for 45 minutes. The samples were cooled on ice for 5 minutes and were vortexed after adding 1 ml of 70% TCA. The samples were centrifuged at 15 000g for 3 minutes and the absorbance of the supernatant was read at 530 nm. A baseline absorbance was taken into account by running a blank along with all samples during the measurement. Calculation of TBARS concentration was based on the molar extinction coefficient of malondialdehyde. The intra- and inter-assay coefficients of variation (CV) for TBARS were 3.9 and 5.9%, respectively.
Protein carbonyls were determined based on the method of Patsoukis et al.Citation28 The derivatization of the carbonyl group with 2,4-dinitrophenylhydrazine (DNPH), which leads to the formation of a stable 2,4-dinitrophenyl hydrazone product, is one of the most widely utilized measurements of protein oxidation.Citation29 Although there are some concerns regarding the specificity of protein carbonyl assay using spectrophotometry, it has been shown to be very sensitive especially for the determination of carbonyl content in purified proteins.Citation29 For this reason, the proteins have been precipitated with TCA. Particularly, in this assay, 50 µl of 20% TCA was added to 50 µl of plasma and this mixture was incubated in an ice bath for 15 minutes and centrifuged at 15 000g for 5 minutes at 4°C. The supernatant was discarded and 500 µl of 10 mM DNPH [in 2.5 N hydrochloride (HCl)] for the sample, or 500 µl of 2.5 N HCl for the blank, was added to the pellet. The samples were incubated in the dark at room temperature for 1 hours, with intermittent vortexing every 15 minutes and were centrifuged at 15 000g for 5 minutes at 4°C. The supernatant was discarded and 1 ml of 10% TCA was added, vortexed, and centrifuged at 15 000g for 5 minutes at 4°C. The supernatant was discarded and 1 ml of ethanol–ethyl acetate (1:1 v/v) was added, vortexed, and centrifuged at 15 000g for 5 minutes at 4°C. This washing step was repeated twice. The supernatant was discarded and 1 ml of 5 M urea (pH 2.3) was added, vortexed, and incubated at 37°C for 15 minutes. The samples were centrifuged at 15 000g for 3 minutes at 4°C and the absorbance was read at 375 nm. Calculation of protein carbonyl concentration was based on the molar extinction coefficient of DNPH. The intra- and inter-assay CV for CARB were 4.3 and 7.0%, respectively. Total plasma protein was assayed using a Bradford reagent from Sigma–Aldrich.
GSH was measured according to Reddy et al.Citation30 Twenty microliters of erythrocyte lysate treated with 5% TCA were mixed with 660 µl of 67 mM sodium potassium phosphate (pH 8) and 330 µl of 1 mM 5,5′-dithiobis-2 nitrobenzoate. Proteins in the erythrocyte lysate were precipitated two times with 5% TCA in order to eliminate protein-linked -SH groups from the measurements. The samples were incubated in the dark at room temperature for 45 minutes and the absorbance was read at 412 nm. GSH concentration was calculated relative to a calibration curve made using commercial standards. The intra- and inter-assay CV for GSH were 3.1 and 4.5%, respectively.
Catalase activity was determined using the method of Aebi.Citation31 Briefly, 4 µl of erythrocyte lysate (diluted 1:10) were added to 2991 µl of 67 mM sodium potassium phosphate (pH 7.4) and the samples were incubated at 37°C for 10 minutes. Five microliters of 30% hydrogen peroxide (H2O2) were added to the samples and the change in absorbance was immediately read at 240 nm for 130 seconds. Calculation of catalase activity was based on the molar extinction coefficient of H2O2. The intra- and inter-assay CV for catalase were 6.2 and 10.0%, respectively.
The determination of TAC was based on the method of Janaszewska and Bartosz.Citation32 Briefly, 20 µl of plasma were added to 480 µl of 10 mM sodium potassium phosphate (pH 7.4) and 500 µl of 0.1 mM 2,2-diphenyl-1-picrylhydrazyl (DPPH) free radical and the samples were incubated in the dark for 30 minutes at room temperature. The samples were centrifuged for 3 minutes at 20 000g and the absorbance was read at 520 nm. The intra- and inter-assay CV for TAC were 2.9 and 5.4%, respectively. TAC is presented as mmol of DPPH reduced to 2,2-diphenyl-1-picrylhydrazine by the antioxidants of plasma.
Plasma CRP measurement
The immunoturbidimetric assay for CRP was carried out using Olympus System CRP reagent, with an Olympus AU2700 apparatus (Rungis, France). The lower detection limit for CRP was 1.57 mg/l. The assay was linear within a 5–300 mg/l concentration range.
Statistical analysis
Oxidative stress, hematological and anthropometric data were analyzed by two-way (treatment time) analysis of variance with repeated measures on time. Pairwise comparisons were performed through simple main-effect analysis. Correlations between sORP or cORP values and the conventional oxidative stress markers’ values were examined by Spearman's correlation analysis. The level of statistical significance was set at p<0.05. For all statistical analyses SPSS, version 13.0 (SPSS Inc., Chicago, IL, USA) was used. Data are presented as mean ± SEM.
Results
Oxidative stress markers
From the two parameters measured by the RedoxSYS diagnostic system, sORP indicating the current redox balance increased significantly (p < 0.05) after the race by 7% compared to pre-race (A). On the contrary, cORP showing the reserves of antioxidants was decreased after the race by 14.5% but not statistically significant (B).
Figure 1. Parameters of the redox status in plasma of mountain marathon athletes at pre- and post-race as measured by the RedoxSYS diagnostic system. (A) sORP; (B) cORP. Significantly different compared to pre-race (p < 0.05).
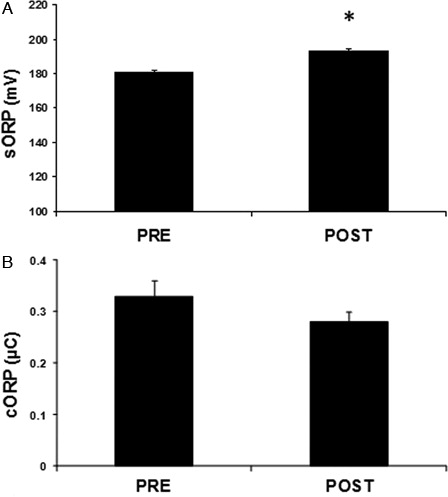
Regarding the ‘conventional’ oxidative stress markers, the plasma levels of CARB indicating protein oxidation increased significantly (p < 0.05) by 15.6% after the race compared to pre-race (A). TAC in plasma increased significantly (p < 0.01) by 6.9% after the race (B). TBARS levels in plasma showing lipid peroxidation increased significantly (p < 0.05) after the race by 26.1% (C). On the other hand, in red blood cells, the non-enzymatic antioxidant GSH levels decreased significantly (p < 0.05) by 13.1% at post-race compared to pre-race (A). Similarly to GSH, the activity of the antioxidant enzyme catalase in red blood cells significantly decreased by 23.4% after the race (B).
Figure 2. Parameters of the redox status in plasma of mountain marathon athletes at pre- and post-race. (A) CARB; (B) TAC; (C) TBARS. Significantly different compared to pre-race (p < 0.05).
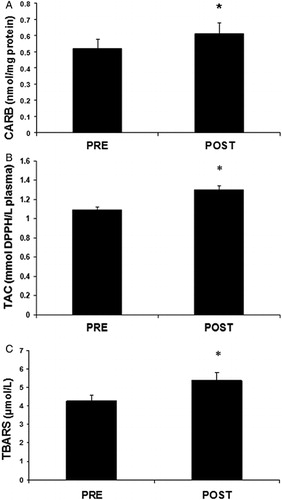
Figure 3. Parameters of the redox status in red blood cells of mountain marathon athletes at pre- and post-race. (A) GSH; (B) CAT. Significantly different compared to pre-race (p < 0.05).
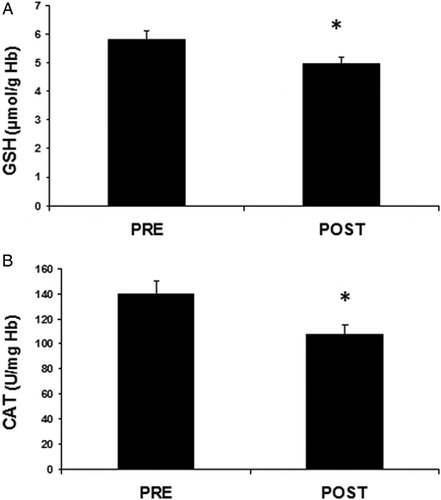
As regards the correlation analysis, it showed that there was strong correlation only between a few of the tested oxidative stress markers (). In particular, sORP values had a significant strong negative correlation (r =−0.855, p < 0.01) only with cORP values ( and ).
Table 1. Correlation coefficient (r) between sORP, cORP, TAC, TBARS, CARB, CAT, GSH, and CRP values of the athletes at post-race
Inflammation marker
The levels of the inflammation biomarker CRP appeared significantly (p < 0.01) higher after the race by 22-fold compared to pre-race levels (). The values of the inflammation marker CRP had only a significant negative correlation with GSH values (r =−0.622, p < 0.05) ().
Discussion
Oxidative stress, the imbalance between the overproduction of ROS and the inability of antioxidant defense mechanisms to inactivate them, is considered a potential cause for a number of pathophysiological conditions and diseases.Citation1 Therefore, evaluation of the redox status of a person in order to predict a disease or monitor the progress of an already occurring disease may be clinically valuable. Direct measurement of ROS is difficult to perform and impossible in the clinical setting, since these direct detection methods (e.g. electron spin resonance) are not yet applicable for clinical examination because of the instability and short half-life of many reactive species and the need for expensive equipment. The most applicable method for assessing the redox status in living organisms is the use of stable markers in the circulation that may indicate systemic oxidative stress.Citation9 For example, since oxidative stress may cause damage to DNA, proteins, and lipids, a number of biomarkers for evaluating oxidatively modified proteins (e.g. protein carbonyl levels), DNA (e.g. measurement of 8-Oxo-2′-deoxyguanosine), and lipids (e.g. TBARS assay) have been developed and used for predicting or monitoring diseases in which ROS are involved.Citation1,Citation5,Citation10 However, the current biomarkers are often insufficient for assessing oxidative stress due to the complex effects of ROS on physiological and pathophysiological processes. Moreover, several oxidative stress biomarkers present problems with specificity, sensitivity, reproducibility, degree of intra- and inter-individual variability, and establishing a causal relation with diseases.Citation9,Citation33 In addition, several oxidative stress biomarkers are of limited use because they require invasive sampling methods, large sample volume or samples having low storage stability.Citation9 Therefore, there is a great need for developing new methods for assessing redox status in humans.
In the present study, ORP as measured by the RedoxSYS diagnostic system was used for the first time in order to assess oxidative stress induced by strenuous exercise, specifically after a mountain marathon race. The results showed that in the participating athletes the sORP values (i.e. the current redox balance) at post race in plasma were significantly higher compared to pre-race values indicating induction of oxidative stress. In contrast to sORP values, the cORP values (i.e. the antioxidant reserves) at post-race in plasma were lower, as expected, compared to pre-race values, although this decrease was not statistically significant. The absence of significance in the case of cORP may be explained by the fact that sometimes, especially in exercise, under oxidative stress conditions the organism increases antioxidant molecules as a compensatory mechanism against free radicals.Citation34 For the validation of the method, it was important that, as expected, there was a significant high negative correlation (r = −0.855, p < 0.01) between sORP and cORP, indicating that in the athletes with the higher oxidative stress there were lower antioxidant reserves.
Apart from ORP values, the oxidative stress in the athletes was assessed using ‘conventional’ oxidative stress biomarkers. There was a consistency between the results from sORP measurements and from the other oxidative stress biomarkers. Namely, the results from the ‘conventional’ biomarkers also supported the induction of oxidative stress at post-race. Specifically, TBARS, a lipid peroxidation marker, in plasma increased significantly after the race. Lipid peroxides are usually formed from the oxidative conversion of polyunsaturated fatty acids and in several studies they increased in runners at post-race.Citation35 Moreover, CARB, a marker of protein oxidation, in plasma increased significantly at post-race. The increase in protein carbonylation following training or single exercise has been reported in many studies.Citation36 Furthermore, in erythrocyte lysate, the levels of GSH, the most important non-enzymatic intracellular antioxidant molecule, decreased significantly at post-race. Most studies investigating the effects of acute aerobic exercise on redox status showed that GSH levels decreased at least in part because of its inactivation of free radicals for regenerating ascorbic acid and alpha tocopherol.Citation37 The most detailed study that has measured GSH levels after aerobic exercise is that of Bloomer et al., in which blood samples were collected at four different time-points post exercise.Citation38 The results showed a decrease in GSH levels only immediately after exercise but not at 1, 6, and 24 hours. In addition, catalase activity decreased at post-race in red blood cells. Catalase, an enzyme catalyzing the decomposition of hydrogen peroxide to water and oxygen, is one of the most important antioxidant enzymes protecting the cell from ROS.Citation1 Other studies have also reported decreases in catalase activity after endurance exercise due to ROS production.Citation39 As the erythrocyte does not synthesize proteins, the changes in catalase activity in erythrocytes could be due to interactions with other enzymes or with reactive oxygen species or molecules generated during exercise, that cause changes in the structure (e.g. covalent protein modification) or the redox status of the protein.Citation40 For example, catalase has been shown to be inhibited by high concentrations of its substrate, hydrogen peroxide.Citation31 The present results were similar to the results from other studies demonstrating that marathon running induces oxidative stress.Citation41,Citation42 In general, it is well established that exhaustive exercise induces ROS production (e.g. due to increase oxygen consumption in mitochondria) predominantly by contracting skeletal and heart muscle.Citation43
Moreover, the significant increase by 22-fold at post-race in the levels of the inflammatory marker CRP supports the oxidative stress induction in the participating athletes. CRP is an inflammatory protein made by liver in response to increases in IL-6 and other inflammatory mediators.Citation44 Many studies have also shown that CRP level increased after exhaustive exercise.Citation45,Citation46 This increase in CRP may be in part attributed to the increased formation of ROS occurring during exercise, since ROS are capable of activating transcription factors known to regulate IL-6 synthesis.Citation25
From the tested oxidative stress biomarkers, only the increase in TAC at post-race indicated an enhancement of the antioxidant mechanisms. This may seem in contrast to oxidative stress induction suggested by the other biomarkers. However, other studies have also reported increases in TAC after exercise, which is explained as a compensatory mechanism to counteract the exercise-induced oxidative stress.Citation34 However, we believe that these increases in TAC after exercise may imply the insufficiency of this method for evaluating in vivo oxidative stress. Namely, in most cases, the evaluation of TAC is based on the reduction of a free radical (e.g. DPPH) by the antioxidant molecules in plasma. Thus, this method is imperfect, since it evaluates only the reductants found in plasma. However, the redox status is based not only on the amount of the reductants but also of the oxidants in the plasma. For this reason, sORP measurement may be a better method than TAC as a holistic approach for assessing in vivo oxidative stress, since sORP is based on the evaluation of both the reductants and the oxidants in plasma.
It is also worth mentioning that the correlation analysis showed that the changes in most of the tested biomarkers do not correlate with each other. As mentioned, there was only a significant negative high correlation between sORP and cORP (r=−0.855, p < 0.01), and a medium negative correlation between GSH and CRP levels (r = −0.622, p < 0.05). The latter correlation may be explained by the fact that GSH reduction leads to increase in ROS that in turn cause inflammation.Citation47 As regards the lack of correlation between the sORP and the other biomarkers of oxidative stress, there was a crucial point. This point was that there was consistency between sORP and the other biomarkers of oxidative stress. Namely, like sORP, the results from the other biomarkers supported the induction of oxidative stress at post race. Specifically, like the significant increase in sORP at post-race has suggested induction of oxidative stress, the significant increases in TBARS, CARB as well as the significant decrease in GSH and catalase activity at post-race have indicated oxidative stress. Moreover, there was a lack of correlation not only between sORP and the other ‘conventional’ markers, but also between the ‘conventional’ oxidative stress biomarkers themselves. Thus, the lack of correlation meant that the different biomarkers have not been changed at the same extent at post-race in each individual, although the overall change of each biomarker at post-race suggested the same induction of oxidative stress. The inter-individual differences in the changes of the different redox biomarkers are emphasized by the fact that there were individuals in whom a biomarker has not been changed at all. For example, although protein carbonyl levels increased at post-race in most participants, they have not been changed in some of them. Furthermore, Margaritelis et al. have examined recently the individual variation of redox biomarkers in exercise-induced oxidative stress and have found a wide heterogeneity of the changes between the different individuals.Citation48 Importantly, they have reported that exercise induced reductive stress or no stress in a considerable number of participants in the study instead of the expected oxidative stress.Citation48 This inter-individual heterogeneity of redox biomarkers can be explained by the inter-individual differences in the antioxidant defense mechanisms. Moreover, oxidative stress is a very complex condition affected by many different factors (e.g. genetic, physiological, biochemical, and dietary), and so it is reasonable to be appeared differently in different individuals. In addition, in many studies (related not only to exercise), it has been shown that some conditions induce oxidative stress by changing some redox biomarkers, while they had no effect on others.Citation49,Citation50 For this reason, in order to make a better conclusion for the oxidative stress levels, several different biomarkers should be examined. There is a higher possibility to be correlation only between biomarkers that somehow are connected. For example, there was a significant correlation between sORP and cORP because their estimation was based on the same methodology.
In conclusion, the present study is the first demonstrating that the ORP as measured by the RedoxSYS diagnostic system can be used for evaluating the exercise-induced oxidative stress in athletes. It is important for ORP measurement to be validated as a reliable assessment of redox status, since it is a holistic approach, minimally invasive, fast, and requires small sample volume. However, the proposed method is more useful for a rapid and general assessment of the redox status. However, if it is needed a more specific assessment of the redox status, then more oxidative stress biomarkers should be measured (e.g. activity of antioxidant enzymes, levels of specific antioxidant molecules, and damage to specific biomolecules). Of course, more studies that will use different types and protocols of exercise as well as larger samples are needed for confirming that ORP measurement can be used reliably for monitoring both the exercise-induced oxidative stress as well as the antioxidant status (with or without use of antioxidant supplements) in athletes. Moreover, the validity of the new proposed measurements may be checked by in vitro analyses, comparing untreated blood with the same after a challenge with oxidants at different concentrations.
Disclaimer statements
Contributors All authors contributed equally.
Funding None.
Conflicts of interest This work was funded by a grant from Luoxis diagnostics company.
Ethics approval The procedures were in accordance with the Helsinki declaration of 1975 as revised in 2000 and approval was received by the human subjects committee of the University of Thessaly.
References
- Halliwell B. Free radicals and other reactive species in disease. In: Wiley John & Sons (eds.) Encyclopedia of life sciences. London: Nature Publishing Group; 2001. p. 1–7.
- Valko M, Leibfritz D, Moncol J, Cronin MTD, Mazur M, Telser J. Free radicals and antioxidants in normal physiological functions and human disease. Int J Biochem Cell B 2007;39:44–84.
- Dupre-Crochet S, Erard M, Nube O. ROS production in phagocytes: why, when, and where? J Leukoc Biol 2013;94(4):657–70.
- Linnane AW, Zhang C, Yarovaya N. Human aging and global function of coenzyme Q10. Ann NY Acad Sci 2002;959:396–411.
- Mylonas C, Kouretas D. Lipid peroxidation and tissue damage. In Vivo 1999;13(3):295–309.
- Schneider BS, Tiidus PM. Neutrophil infiltration in exercise-injured skeletal muscle: how do we resolve the controversy. Sports Med 2007;37:837–56.
- Nikolaidis MG, Paschalis V, Giakas G, Fatouros IG, Koutedakis Y, Kouretas D, et al. Decreased blood oxidative stress after repeated muscle-damaging exercise. Med Sci Sports Exerc 2007;39(7):1080–9.
- Meeus M, Nijs J, Hermans L, Goubert D, Calders P. The role of mitochondrial dysfunctions due to oxidative and nitrosative stress in the chronic pain or chronic fatigue syndromes and fibro myalgia patients: peripheral and central mechanisms as therapeutic targets. Expert Opin Ther Targets 2013;17:1081–9.
- Dalle-Donne I, Rossi R, Colombo R, Giustarini D, Milzani A. Biomarkers of oxidative damage in human disease. Clin Chem 2006;52(4):601–23.
- Ogino K, Wang DH. Biomarkers of oxidative/nitrosative stress: an approach to disease prevention. Acta Med Okayama 2007;61(4):181–9.
- Harris C, Hansen JM. Oxidative stress, thiols, and redox profiles. Methods Mol Biol 2012;889:325–46.
- Betters JL, Criswell DS, Shanely RA, Van Gammeren D, Falk D, Deruisseau KC, et al. Trolox attenuates mechanical ventilation-induced diaphragmatic dysfunction and proteolysis. Am J Respir Crit Care Med 2004;170:1179–84.
- Veskoukis AS, Nikolaidis MG, Kyparos A, Kokkinos D, Nepka C, Barbanis S, et al. Effects of xanthine oxidase inhibition on oxidative stress and swimming performance in rats. Appl Physiol Nutr Metab 2008;33:1140–54.
- Davies KJ, Quintanilha AT, Brooks GA, Packer L. Free radicals and tissue damage produced by exercise. Biochem Biophys Res Commun 1982;107:1198–205.
- Skenderi KP, Tsironi M, Lazaropoulou C, Anastasiou CA, Matalas AL, Kanavaki I, et al. Changes in free radical generation and antioxidant capacity during ultramarathon foot race. Eur J Clin Invest 2008;38(3):159–65.
- Kłapcińska B, Waśkiewicz Z, Chrapusta SJ, Sadowska-Krępa E, Czuba M, Langfort J. Metabolic responses to a 48-h ultra-marathon run in middle-aged male amateur runners. Eur J Appl Physiol 2013;113(11):2781–93.
- Briviba K, Watzl B, Nickel K, Kulling S, Bös K, Haertel S, et al. A half-marathon and a marathon run induce oxidative DNA damage, reduce antioxidant capacity to protect DNA against damage and modify immune function in hobby runners. Redox Rep 2005;10(6):325–31.
- Tsai K, Hsu TG, Hsu KM, Cheng H, Liu TY, Hsu CF, et al. Oxidative DNA damage in human peripheral leukocytes induced by massive aerobic exercise. Free Radic Biol Med 2001;31(11):1465–72.
- Liu ML, Bergholm R, Mäkimattila S, Lahdenperä S, Valkonen M, Hilden H, et al. A marathon run increases the susceptibility of LDL to oxidation in vitro and modifies plasma antioxidants. Am J Physiol 1999;276(6 Part 1):E1083–91.
- Inayama T, Kumagai Y, Sakane M, Saito M, Matsuda M. Plasma protein-bound sulfhydryl group oxidation in humans following a full marathon race. Life Sci 1996;59(7):573–8.
- Hessel E, Haberland A, Müller M, Lerche D, Schimke I. Oxygen radical generation of neutrophils: a reason for oxidative stress during marathon running? Clin Chim Acta 2000;298(1–2):145–56.
- Gomez-Cabrera MC, Domenech E, Romagnoli M, Arduini A, Borras C, Pallardo FV, et al. Oral administration of vitamin C decreases muscle mitochondrial biogenesis and hampers training-induced adaptations in endurance performance. Am J Clin Nutr 2008;87:142–9.
- Powers SK, Talbert EE, Adhihetty PJ. Reactive oxygen and nitrogen species as intracellular signals in skeletal muscle. J Physiol 2011;589:2129–38.
- Aoi W, Naito Y, Yoshikawa T. Role of oxidative stress in impaired insulin signaling associated with exercise-induced muscle damage. Free Radic Biol Med 2013;65:1265–72.
- Fischer CP. Interleukin-6 in acute exercise and training: what is the biological relevance? Exerc Immunol Rev 2006;12:6–33.
- Keles MS, Taysi S, Sen N, Aksoy H, Akçay F. Effect of corticosteroid therapy on serum and CSF malondialdehyde and antioxidant proteins in multiple sclerosis. Can J Neurol Sci 2001;28(2):141–3.
- Lykkesfeldt J. Malondialdehyde as biomarker of oxidative damage to lipids caused by smoking. Clin Chim Acta 2007;380(1–2):50–8.
- Patsoukis N, Zervoudakis G, Panagopoulos NT, Georgiou CD, Angelatou F, Matsokis NA. Thiol redox state (TRS) and oxidative stress in the mouse hippocampus after pentylenetetrazol-induced epileptic seizure. Neurosci Lett 2004;357:83–6.
- Luo S, Wehr NB. Protein carbonylation: avoiding pitfalls in the 2,4-dinitrophenylhydrazine assay. Redox Rep 2009;14(4):159–66.
- Reddy YN, Murthy SV, Krishna DR, Prabhakar MC. Role of free radicals and antioxidants in tuberculosis patients. Indian J Tuberc 2004;51:213–8.
- Aebi H. Catalase in vitro. Methods Enzymol 1984;105:121–6.
- Janaszewska A, Bartosz G. Assay of total antioxidant capacity: comparison of four methods as applied to human blood plasma. Scand J Clin Lab Invest 2002;62(3):231–6.
- Ho E, Karimi Galougahi K, Liu CC, Bhindi R, Figtree GA. Biological markers of oxidative stress: applications to cardiovascular research and practice. Redox Biol 2013;1(1):483–91.
- Fatouros IG, Jamurtas AZ, Villiotou V, Pouliopoulou S, Fotinakis P, Taxildaris K, et al. Oxidative stress responses in older men during endurance training and detraining. Med Sci Sports Exerc 2004;36(12):2065–72.
- Nikolaidis MG, Jamurtas AZ, Paschalis V, Fatouros IG, Koutedakis Y, Kouretas D. The effect of muscle-damaging exercise on blood and skeletal muscle oxidative stress: magnitude and time-course considerations. Sports Med 2008;38(7):579–606.
- Jammes Y, Steinberg JG, Bregeon F. The oxidative stress in response to routine incremental cycling exercise in healthy sedentary subjects. Respir Physiol Neurobiol 2004;144:81–90.
- Finaud J, Lac G, Filaire E. Oxidative stress: relationship with exercise and training. Sports Med 2006;36:327–58.
- Bloomer RJ, Goldfarb AH, Wideman L, McKenzie MJ, Consitt LA. Effects of acute aerobic and anaerobic exercise on blood markers of oxidative stress. J Strength Cond Res 2005;19(2):276–85.
- Sureda A, Ferrer MD, Tauler P, Maestre I, Aguiló A, Córdova A. Intense physical activity enhances neutrophil antioxidant enzyme gene expression. Immunocytochemistry evidence for catalase secretion. Free Radic Res 2007;41(8):874–83.
- Tauler P, Aguiló A, Guix P, Jiménez F, Villa G, Tur JA, et al. Pre-exercise antioxidant enzyme activities determine the antioxidant enzyme erythrocyte response to exercise. J Sports Sci 2005;23(1):5–13.
- Hattori N, Hayashi T, Nakachi K, Ichikawa H, Goto C, Tokudome Y, et al. Changes of ROS during a two-day ultra-marathon race. Int J Sports Med 2009;30(6):426–9.
- Briviba K, Watzl B, Nickel K, Kulling S, Bös K, Haertel S, et al. A half-marathon and a marathon run induce oxidative DNA damage, reduce antioxidant capacity to protect DNA against damage and modify immune function in hobby runners. Redox Rep 2005;10(6):325–31.
- Powers SK, Jackson MJ. Exercise-induced oxidative stress: cellular mechanisms and impact on muscle force production. Physiol Rev 2008;88(4):1243–76.
- Edward TH. A new perspective on the biology of C-reactive protein. Circ Res 2005;97:609–11.
- Kerasioti E, Stagos D, Jamurtas A, Kiskini A, Koutedakis Y, Goutzourelas N. Anti-inflammatory effects of a special carbohydrate-whey protein cake after exhaustive cycling in humans. Food Chem Toxicol 2013;61:42–6.
- Afroundeh R, Siahkouhian M, Khalili A. The effect of post-exercise carbohydrate ingestion on inflammatory responses to short time, high-force eccentric exercise. J Sports Med Phys Fitness 2010;50(2):182–8.
- Nadeem A, Siddiqui N, Alharbi NO, Alharbi MM, Imam F. Acute glutathione depletion leads to enhancement of airway reactivity and inflammation via p38MAPK-iNOS pathway in allergic mice. Int Immunopharmacol 2014;22(1):222–9.
- Margaritelis NV, Kyparos A, Paschalis V, Theodorou AA, Panayiotou G, Zafeiridis A, et al. Reductive stress after exercise: the issue of redox individuality. Redox Biol 2014;19(2):520–8.
- Kisa U, Caglayan O, Kacmaz M. The effects of topotecan on lipid peroxidation and antioxidant enzyme levels in rabbit liver tissue. Redox Rep 2005;10(2):79–82.
- Ziolkowski W, Flis DJ, Halon M, Vadhana DM, Olek RA, Carloni M, et al. Prolonged swimming promotes cellular oxidative stress and p66Shc phosphorylation, but does not induce oxidative stress in mitochondria in the rat heart. Free Radic Res 2014;21:1–10.