Abstract
Objective
The treatment of schizophrenia is multifactorial, with antipsychotic medications comprising a major part of treatment. Paliperidone is a newly commercialized antipsychotic whose formulation includes the principal active metabolite risperidone, 9-hydroxyrisperidone. Ever since the relationship between schizophrenia and oxidative stress was first demonstrated, many studies have been conducted in order to probe the potential protective effects of antipsychotic drugs on the oxidant–antioxidant system and lipid peroxidation. The basic aim of this study is to determine the effects of the newly marketed drug paliperidone on the activities of the enzymes adenosine deaminase (ADA), xanthine oxidase (XO), superoxide dismutase (SOD), catalase (CAT), and glutathione peroxidase (GSH-Px) as well as on malondialdehyde (MDA) and nitric oxide (NO) levels in rat brain tissues.
Methods
Twenty male Sprague-Dawley rats were used for the study, which were divided into two equal groups. The first was the control group (n = 10) and the second was the paliperidone group (n = 10). Saline was administered once daily for 14 days in the control group. In the paliperidone group, paliperidone was administered once daily with a dose of 1 mg/kg for 14 days. All rats were sacrificed at the end of the fourteenth day. Brain samples were collected and then analyzed.
Results
Our results demonstrated that paliperidone significantly decreased the activities of ADA (P = 0.015), XO (P = 0.0001), and CAT (P = 0.004) while insignificantly increasing the activity of SOD (P = 0.49), MDA (P = 0.71), and NO (P = 0.26) levels in rat brain tissues. In addition, paliperidone insignificantly decreased the activity of GSH-Px (P = 0.30) compared to the control group in rat brain tissues.
Discussion
In conclusion, the data obtained in this study suggest that paliperidone can positively alter antioxidant status and, accordingly, can offer positive outcomes in the treatment of schizophrenia by reducing activity in the enzymes ADA and XO, which are associated with purine metabolism. We believe that such a comprehensive approach used with other antipsychotic drugs warrants further study.
Introduction
Schizophrenia is a severe, chronic, and debilitating psychiatric disease that affects close to 1% of the world's population; however, its etiology remains incompletely understood.Citation1 An increasing number of studies suggest that, genetic factors aside, reactive oxygen species (ROS) may play a crucial role in the pathogenesis of schizophrenia.Citation2 Antipsychotic drugs are commonly administered in the treatment of schizophrenia. With older generation (typical) antipsychotic drugs, tardive dyskinesia and extrapyramidal side effects are frequently observed side effects of treatment. It has been suggested that oxidative stress may be responsible for the etiology of tardive dyskinesia, which may develop after antipsychotic treatment.Citation3
Oxidative stress is a common term to describe an imbalance between oxidants and antioxidants in favor of oxidants.Citation4 ROS can be produced under physiological conditions. If the excess amount of ROS cannot be detoxified by antioxidant defense mechanisms, cellular damage will occur (lipid peroxidation, DNA damage, etc.). Malondialdehyde (MDA) is one of the major signs of lipid peroxidation. Superoxide dismutase (SOD), catalase (CAT), and glutathione peroxidase (GSH-Px) play key roles in antioxidant defense mechanisms by scavenging and decomposing oxidant products into water (H2O) ().
Abnormalities in antioxidant defense systems and major decreases in antioxidant levels were determined in schizophrenic patients.Citation2,Citation5 There are many studies with differing results concerning the oxidative stress markers in schizophrenia patients.Citation6 Some articles stated that the SOD activity of red blood cells (RBC) in schizophrenic patients was found to be increased,Citation7 whereas others found it to be either unaffectedCitation8 or reduced,Citation9 while CAT and GSH-Px activities were found to be elevatedCitation8 or unaffected.Citation10 Since the relationship between oxidative stress and schizophrenia was established, several studies have been performed on the possible protective effects of antipsychotic drugs on oxidant–antioxidant systems and lipid peroxidation. The effects of antipsychotic drugs on oxidant, antioxidant, and lipid peroxidation have become a popular subject. It has also been suggested that some older generation antipsychotics may have shown pro-oxidant effects by increasing the cellular damage, especially in the brain.Citation11,Citation12 On the other hand, some antipsychotic drugs were reported to have neuroprotective effects against oxidative stress at the cellular level.Citation13,Citation14
Figure 1. Schematic representation of purine metabolism, free-oxygen generation, enzymatic antioxidant defense system, and lipid peroxidation.
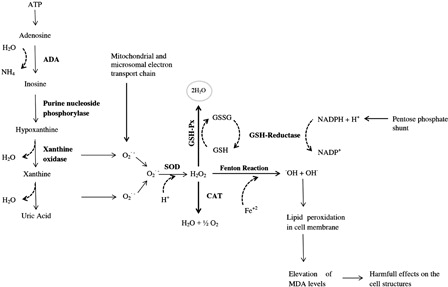
Adenosine deaminase (ADA) plays a key role in purine nucleotide catabolism and catalyzes adenosine into inosine. Moreover, ADA is the enzyme that catalyzes deoxiadenosine into deoxiinosine.Citation15 It has been previously demonstrated that adenosine, as a neuromodulator, is related to the pathophysiology of schizophrenia.Citation16,Citation17 Impaired mechanisms in adenosinergic activity may lead to significant alterations of glutamergic and dopaminergic activities in schizophrenia. Furthermore, it was reported that adenosine may be responsible for some systemic changes in schizophrenic patients.Citation18
Xanthine oxidase (XO) is one of the enzymes that catalyze hypoxanthine into xanthine and xanthine into uric acid. XO is also one of the most important enzymatic sources of superoxide anion radical .Citation2 Inhibition of XO has been shown to have positive effects on schizophrenia patients.Citation19
Nitric oxide (NO) is commonly known to act as a neurotransmitter in the central and peripheral nervous systems. NO is also known as a member of the ROS family.Citation2 NO can interact and couple with to produce peroxynitrite, a compound that is harmful to cellular structures. Elevated NO levels were reported in schizophrenic patients.Citation20
Paliperidone, the main active metabolite of risperidone, was formulated by using 9-hydroxyrisperidone and was developed as a new atypical antipsychotic agent.Citation21 Although paliperidone is an active metabolite of risperidone, the World Health Organization classifies paliperidone as a separate medication in terms of its therapeutic and chemical features. Paliperidone has some differences and advantages compared to risperidone. First of all, paliperidone provides constant release due to its osmotic formulation, which reduces the fluctuation of plasma levels in the drug. Moreover, continuous release simplifies the stable occupancy of D2 receptors. Second, paliperidone is scarcely metabolized by CYP450 isoenzymes, which is an advantage regarding drug interactions. Third, compared to risperidone, paliperidone rapidly dissociates from D2 receptors. This causes lower extrapyramidal side effects.Citation22
In summary, the main purpose of the current study is to investigate the effects of the newly marketed paliperidone on purine metabolism and oxidant–antioxidant parameters in rat brains.
Material and methods
Chemicals
All chemicals were obtained from Sigma Chemical, Inc. (St. Louis, MO, USA), and all organic solvents were obtained from Merck Chemical, Inc. (Darmstadt, Germany). All reagents were of analytical grade and, except for the phosphate buffers, were prepared each day and stored in a refrigerator at 4°C. The reagents were equilibrated at room temperature for 0.5 hours before use (i.e., whenever the analysis was initiated or reagent containers were refilled). Phosphate buffers were stable at 4°C for 1 month.
Experimental animals
This study was conducted at the Experimental Animal Research Laboratory and the Department of Medical Biology, School of Medicine at Suleyman Demirel University in Isparta, Turkey. All experimental protocols of the current study were approved by the Ethical Committee of the Experimental Animal Laboratory of Suleyman Demirel University (02-06-09-13-01). Male Sprague-Dawley rats (200–260 ± 19 g) were used in the current experiment. Animals were maintained and used in accordance with the Animal Welfare Act and with the guide for the care and use of laboratory animals, which was prepared by the Ethical Committee of Suleyman Demirel University. All animals were kept singly in stainless steel cages in a pathogen-free environment in our laboratory at 23 ± 2°C with light from 08:00 to 20:00 (12 hours light/dark) and had ad libitum access to a commercial dry diet and liquid water.
The rats were randomly divided into two main groups, which were, respectively, identified as control and paliperidone, as indicated below.
Group 1 (control) (n = 10): physiological serum (0.09% NaCl), including 0.3% l-tartaric acid (pH 4), was intraperitoneally administered to rats once daily for 14 days.
Group 2 (paliperidone) (n = 10): paliperidone tablets were dissolved into physiological serum, including 0.3% l-tartaric acid (pH 4). Twenty-four hours after preparation, the solution was administered intraperitoneally to the rats as 1 mg/kg/day, once daily during a period of 14 days.Citation23,Citation24
Anesthesia and tissue samples
Animals fasted and rested for 24 hours after the final injection of paliperidone on the fifteenth day of the experiment. The rats were sacrificed under ketamine (90 mg/kg) and xylazine HCl (10 mg/kg) anesthesia. After decapitation, the brains were removed and maintained at −20°C until biochemical analyses were performed.
Homogenization and preparing samples for analysis
Brain tissue samples were homogenized in 50 mM Tris–HCl, pH 7.4. After homogenization, samples were centrifuged at 16 000 rpm for 3 minutes. Homogenates were immediately transferred to Eppendorf tubes and centrifuged again at 5000 rpm for 30 minutes at 4°C (Hettich Centrifuge, Tuttlingen, Germany). CAT, XO, and ADA experiments were performed on the samples. Supernatants were diluted and vortexed with chloroform/ethanol to 1/1 (v/v) and then centrifuged at 3200 rpm for 40 minutes at 4°C. SOD, GSH-Px activities, and protein levels were determined from the supernatant.
Determination of SOD activity
Total (Cu-Zn and Mn) SOD (EC 1.15.1.1) activity was determined according to Sun et al.'s method.Citation25 The basic principle of the current method is based on the inhibition of nitro blue tetrazolium (NBT) reduction by the xanthine–xanthine oxidase system as a superoxide generator. Activity was assessed in the ethanol phase of the supernatant after 1.0 ml ethanol/chloroform mixture (5/3, v/v) was added to the same sample volume and centrifuged at 4000 rpm. One unit of SOD was defined as the amount of enzymes required to cause 50% inhibition of the NBT reduction rate. Absorbance was read at 560 nm and SOD activity was also expressed as units per milligram of protein.
Determination of catalase activity
CAT (EC 1.11.1.6) activity was determined according to Aebi's method.Citation26 The basic principle of the current method was based on the determination of the rate constant (k) or the H2O2 decomposition rate at 240 nm. The results were expressed as k (s−1)/g of protein in the brain tissue.Citation26
Determination of glutathione peroxidase activity
GSH-Px (EC 1.6.4.2) activity was measured using Paglia and Valentine's method.Citation27 The enzyme reaction in the tube, which contains nicotinamide adenine dinucleotide phosphate (NADPH), reduced glutathione, sodium azide, and glutathione reductase. It was initiated by the addition of hydrogen peroxide, and the change in absorbance at 340 nm was monitored using a spectrophotometer. Activity was recorded as units per gram of protein in the brain tissue. All samples were assayed in duplicate.Citation27
Determination of XO activities
Tissue XO (EC 1.2.3.2) activity was measured spectrophotometrically by the formation of uric acid from xanthine through the increase in absorbance at 293 nm.Citation28 A calibration curve was constructed by using 10–50 mU/ml concentrations of standard XO solutions (Sigma X-1875). One unit of activity was defined as 1 μmol urate formed per minute at 37°C in a phosphate buffer (pH 7.5, 50 mM). The results were expressed in units per gram protein.Citation28
Determination of adenosine deaminase activities
ADA (EC 3.5.4.4) activity in the brain tissues was estimated spectrophotometrically using the Giusti method,Citation29 which is based on the direct measurement of the ammonia produced when ADA acts in excess of adenosine. The results were expressed as units per gram protein.
Determination of lipid peroxidation
The level of MDA, which acts as an indicator of the free radical generation that increases at the end of lipid peroxidation, was estimated using Draper and Hadley's double heating method.Citation30
The principle of the method is based on the spectrophotometric measurement of color generated by the reaction of thiobarbituric acid with MDA. The concentration of MDA was calculated by the absorbance coefficient of the MDA–thiobarbituric acid complex (absorbance coefficient ɛ = 1.56 × 105 cm−1 M−1) and is expressed as nanomoles per gram of protein.
Determination of NO levels
Measuring NO is very difficult in biological specimens because it is easily oxidized to nitrite (NO−) and subsequently to nitrate (NO−3), which serve as index parameters of NO production. The method for measuring the RBCs' nitrite and nitrate levels was based on the Griess reaction.Citation31
Samples were initially deproteinized with a Somogyi reagent. Total nitrite (NO−2 + NO−3) was measured by spectrophotometry (Shimadzu, UV-Pharmaspec 1700, Tokyo, Japan) at 545 nm after conversion of NO−2 to NO−3 by copperized cadmium granules. The results were expressed as micromole per gram of protein. The Hb in RBC was measured by Drabkin's reagent, which contains potassium ferricyanide and potassium cyanide.
Protein estimation
The protein content of the brain tissue was measured according to Lowry et al.'s method,Citation32 using bovine serum where albumin is used as a standard.
Statistical analysis
Data were presented as mean ± standard deviation. P values of less than 0.001 were noted as significant through the use of a computer program (SPSS 9.0; SPSS, Inc., Chicago, IL, USA) for statistical analysis. As for the data of biochemical measurements, one-way analysis of variance and post hoc multiple comparison tests Least Significant Difference (LSD) were performed on the data of biochemical variables to examine the differences among groups.
Results
Levels of SOD, GSH-Px, MDA, and NO
The values of the SOD, GSH-Px, MDA, and NO levels are shown in . These variables did not show any statistically significant changes between the control and paliperidone groups (P > 0.05).
Table 1. SOD, GSH-Px, MDA, and NO levels in rat brain tissues. The results are shown as mean ± SD. We did not determine any statistically significant changes in SOD, GSH-Px, MDA, and NO levels in rat brain tissues
Levels of ADA activities in rat brain tissues
The levels of ADA activities in rat brain tissues are shown in . We determined a statistically significant decrease in ADA activities in the paliperidone group compared to the control group (P = 0.015).
Levels of XO activities in rat brain tissues
The levels of XO activities in rat brain tissues are shown in . We determined a statistically significant decrease in XO activities in the paliperidone group compared to the control group (P = 0.0001).
Levels of CAT activities in rat brain tissues
The levels of CAT activities in rat brain tissues are shown in . We determined a statistically significant decrease in CAT activities in the paliperidone group compared to the control group (P = 0.004).
Discussion
The most important findings of the current study are the decreased levels of three important enzymes. We determined a statistically significant decrease in ADA, XO, and CAT levels in rat brains. According to our findings, this is the first study to clarify the effects of paliperidone on oxidative stress parameters in rat brains. We also determined some alterations in SOD, MDA, GSH-Px, and NO levels in brain tissues, but these were statistically insignificant. According to these results, paliperidone acts as a protective agent by interacting with DNA mechanisms such as purin and pyrimidine bases.
Our main goal was to determine the effects of paliperidone on brain enzyme activities as well as its effects on some antioxidant parameters. Brain tissue is vulnerable to oxidant agents, such as ROS, due to the consumption of high levels of oxygen and because it is composed of unsaturated fatty acids.Citation33 Increased catecholamine metabolism causes free radical toxicity in schizophrenia. This pathway will result in increased levels of lipid peroxidation.Citation34 According to this knowledge, we aimed to evaluate the possible effects of the newly marketed antipsychotic drug paliperidone on adenosinergic activity in rat brains. Golembiowska et al.Citation35 determined that adenosine deficiency may increase dopamine levels in rat striatum with a reduced inhibiting effect of adenosine on dopamine release. It has been previously reported that adenosine is a regulator of the bioenergetics network that has been observed to modulate both glutamatergic and dopaminergic neurotransmission.Citation36 Moreover, Lara et al.Citation37 suggested that dysfunction in purine metabolism would result in decreased levels of adenosinergic activity, which will cause an imbalance between glutamatergic and dopaminergic neurotransmission. This mechanism is accepted as the milestone of the pathophysiology of schizophrenia.Citation16,Citation17 We determined a statistically significant reduction in ADA levels (P = 0.015). Thus, decreased levels of ADA may cause significant increases in adenosine levels. Subsequently, the increased adenosine levels may result in decreased dopamine levels, and the decreased dopamine levels may recover positive psychotic symptoms of schizophrenia, such as hallucinations, delusions, and disorganized thinking. Similarly, dipyridamole, an inhibitor of adenosine transport, has beneficial effects in schizophrenia patients. Furthermore, by increasing the levels of adenosine, dipyridamole has effects similar to allopurinol.Citation38 Allopurinol, an XO inhibitor, has already been studied as an additional treatment method in schizophrenia patients.Citation19,Citation37,Citation39 It functions as an inhibitor in the major degradation pathway of purines and is strongly believed to increase levels of purines, including adenosine.Citation18 In our experimental model, we determined that XO levels were significantly reduced (P = 0.0001) in the paliperidone group. Our results also support and overlap with these findings. These findings demonstrated that reduced levels of XO will increase adenosine levels, which will in turn result in beneficial effects in the treatment of schizophrenia. Additionally, since XO is a major source of O2–, reduced levels of XO will also lead to a reduction in ROS production.
We also determined that CAT levels were significantly lower in the paliperidone group (P = 0.004). However, we did not determine any statistical significance in GSH-Px, SOD, and MDA levels. Although we performed our study in rat brain tissues, the alterations in tissue enzyme activities may also reflect alterations in plasma enzyme activities.Citation2 Our results also overlap with another study, which was performed by Tsai et al. on oxidative stress markers in schizophrenia patient's serum samples. They also determined significantly decreased levels of CAT activity; however, other oxidative stress markers, including MDA, SOD, and GSH-Px levels, did not show any significant changes in patients with schizophrenia who had received the paliperidone for 4 weeks.Citation40 In some experimental animal studies, scientists determined discrepant results concerning levels of antioxidant enzymes and lipid peroxidation when using different typical or atypical antipsychotic drugs in rat brains.Citation41–Citation43 Our research is original because it is the first to determine the effects of paliperidone on rat brains. Paliperidone is the active metabolite of risperidone. CAT activity was determined to be significantly decreased in risperidone-administered rats.Citation44 The results that we obtained, determining the effects of paliperidone on CAT activity, were also supported by the findings determined by Pillai et al.Citation44 MDA, GSH-Px, and SOD play key roles in oxidative stress mechanisms (). We did not determine any statistically significant changes in MDA, GSH-Px, and SOD levels in paliperidone-administered rat brains. These findings clearly demonstrate that paliperidone did not cause any oxidative stress-induced neuronal cell damage. Kropp et al.Citation45 evaluated plasma MDA levels and determined increased MDA levels in patients who were administered typical antipsychotic drugs compared to atypical antipsychotic drugs. A study that determined the effects of risperidone on C6 astroglial cells demonstrated that risperidone did not trigger oxidative stress but reinforced antioxidant capacity by refraining from altering the MDA levels.Citation46 Similarly, we also determined that paliperidone did not show any statistically significant effects on MDA levels. Additionally, we determined unchanged levels of NO in our experimental model. Taneli et al.Citation47 did not determine any statistical changes in NO metabolite levels, although they administered antipsychotic drugs to schizophrenic patients for 8 weeks. Tarazi et al.Citation41 pointed out that atypical antipsychotic drugs like risperidone, olanzapine, and quetiapine have minimal efficiency on neuronal NO mechanisms in rat brains.
As a result of this study, we determined that paliperidone may have positive effects on schizophrenia treatment by interacting with the purine mechanism and reducing XO and ADA levels. Our findings suggest that paliperidone may offer favorable neuroprotective effects. According to our findings, paliperidone administration did not cause any elevation in oxidative stress markers. Thus, the mechanism of free radical generation differs according to the type of antipsychotic drugs used. Future studies will be necessary to investigate the relationship between antioxidant capacity and the efficiency of antipsychotic drugs.
Acknowledgements
This study was supported by The Scientific Research Projects Unit of Suleyman Demirel University with 1889-TU-09 project number.
Disclaimer statements
Contributors The current study was designed by K.D., R.O., and H.R.Y.. A.Y., K.D., and K.K. were responsible for analysis. K.D. and A.C.U. were responsible for writing the report. H.R.Y. was responsible for statistical analyses of the data. A.D. and A.A. made critical revision on the manuscript.
Funding None.
Conflicts of interest None.
Ethics approval Our paper has received ethical approval from Ethical Committee of Experimental Animal Laboratory of Suleyman Demirel University (02-06-09-13-01).
References
- Frangou S, Murray RM. Imaging as a tool in exploring the neurodevelopment and genetics of schizophrenia. Br Med Bull 1996;52:587–96.
- Akyol O, Herken H, Uz E, Fadillioglu E, Unal S, Sogut S, et al. The indices of endogenous oxidative and antioxidative processes in plasma from schizophrenic patients. The possible role of oxidant/antioxidant imbalance. Prog Neuropsychopharmacol Biol Psychiatry 2002;26:995–1005.
- Wu JQ, Chen DC, Tan YL, Tan SP, Wang ZR, Xiu MH, et al. Cognition impairment in schizophrenia patients with tardive dyskinesia: association with plasma superoxide dismutase activity. Schizophr Res 2013;152:210–6.
- Naziroglu M. Molecular role of catalase on oxidative stress-induced Ca(2+) signaling and TRP cation channel activation in nervous system. J Recept Signal Transduct Res 2012;32:134–41.
- Dakhale G, Khanzode S, Khanzode S, Saoji A, Khobragade L, Turankar A. Oxidative damage and schizophrenia: the potential benefit by atypical antipsychotics. Neuropsychobiology 2004;49:205–9.
- Ciobica A, Padurariu M, Dobrin I, Stefanescu C, Dobrin R. Oxidative stress in schizophrenia – focusing on the main markers. Psychiatr Danub 2011;23:237–45.
- Vaiva G, Thomas P, Leroux JM, Cottencin O, Dutoit D, Erb F, et al. Erythrocyte superoxide dismutase (eSOD) determination in positive moments of psychosis. Therapie 1994;49:343–8.
- Herken H, Uz E, Ozyurt H, Sogut S, Virit O, Akyol O. Evidence that the activities of erythrocyte free radical scavenging enzymes and the products of lipid peroxidation are increased in different forms of schizophrenia. Mol Psychiatry 2001;6:66–73.
- Mukerjee S, Mahadik SP, Scheffer R, Correnti EE, Kelkar H. Impaired antioxidant defense at the onset of psychosis. Schizophr Res 1996;19:19–26.
- Yao JK, Reddy R, McElhinny LG, van Kammen DP. Effects of haloperidol on antioxidant defense system enzymes in schizophrenia. J Psychiatr Res 1998;32:385–91.
- Reddy RD, Yao JK. Free radical pathology in schizophrenia: a review. Prostaglandins Leukot Essent Fatty Acids 1996;55:33–43.
- Mahadik SP, Mukherjee S. Free radical pathology and antioxidant defense in schizophrenia: a review. Schizophr Res 1996;19:1–17.
- Wang H, Xu H, Dyck LE, Li XM. Olanzapine and quetiapine protect PC12 cells from beta-amyloid peptide(25–35)-induced oxidative stress and the ensuing apoptosis. J Neurosci Res 2005;81:572–80.
- Wei Z, Bai O, Richardson JS, Mousseau DD, Li XM. Olanzapine protects PC12 cells from oxidative stress induced by hydrogen peroxide. J Neurosci Res 2003;73:364–8.
- Lloyd HG, Fredholm BB. Involvement of adenosine deaminase and adenosine kinase in regulating extracellular adenosine concentration in rat hippocampal slices. Neurochem Int 1995;26:387–95.
- Lara DR, Souza DO. Schizophrenia: a purinergic hypothesis. Med Hypotheses 2000;54:157–66.
- Lara DR, Dall'Igna OP, Ghisolfi ES, Brunstein MG. Involvement of adenosine in the neurobiology of schizophrenia and its therapeutic implications. Prog Neuropsychopharmacol Biol Psychiatry 2006;30:617–29.
- Brunstein MG, Silveira EM Jr, Chaves LS, Machado H, Schenkel O, Belmonte-de-Abreu P, et al. Increased serum adenosine deaminase activity in schizophrenic receiving antipsychotic treatment. Neurosci Lett 2007;414:61–4.
- Buie LW, Oertel MD, Cala SO. Allopurinol as adjuvant therapy in poorly responsive or treatment refractory schizophrenia. Ann Pharmacother 2006;40:2200–4.
- Herken H, Uz E, Ozyurt H, Akyol O. Red blood cell nitric oxide levels in patients with schizophrenia. Schizophr Res 2001;52:289–90.
- Spina E, Cavallaro R. The pharmacology and safety of paliperidone extended-release in the treatment of schizophrenia. Expert Opin Drug Saf 2007;6:651–62.
- Álamo C, López-Muñoz F. The pharmacological role and clinical applications of antipsychotics’ active metabolites: paliperidone versus risperidone. Clin Exp Pharmacol 2013;3:117. doi:10.4172/2161–1459.1000117.
- Corena-McLeod Mdel P, Oliveros A, Charlesworth C, Madden B, Liang YQ, Boules M, et al. Paliperidone as a mood stabilizer: a pre-frontal cortex synaptoneurosomal proteomics comparison with lithium and valproic acid after chronic treatment reveals similarities in protein expression. Brain Res 2008;1233:8–19.
- Dremencov E, El Mansari M, Blier P. Distinct electrophysiological effects of paliperidone and risperidone on the firing activity of rat serotonin and norepinephrine neurons. Psychopharmacology (Berl) 2007;194:63–72.
- Sun Y, Oberley LW, Li Y. A simple method for clinical assay of superoxide dismutase. Clin Chem 1988;34:497–500.
- Aebi H. Catalase in vitro. Methods Enzymol 1984;105:121–6.
- Paglia DE, Valentine WN. Studies on the quantitative and qualitative characterization of erythrocyte glutathione peroxidase. J Lab Clin Med 1967;70:158–69.
- Prajda N, Weber G. Malignant transformation-linked imbalance: decreased xanthine oxidase activity in hepatomas. FEBS Lett 1975;59:245–9.
- Giusti G, Castagnari L, Gakis C, Galanti B. Evaluation of the efficacy of laboratory diagnosis of typhoid infection. (Latex test, conditioned hemagglutination, adenosine deaminase activity in the serum). G Mal Infett Parassit 1972;24:296–9.
- Draper HH, Hadley M. Malondialdehyde determination as index of lipid peroxidation. Methods Enzymol 1990;186:421–31.
- Cortas NK, Wakid NW. Determination of inorganic nitrate in serum and urine by a kinetic cadmium-reduction method. Clin Chem 1990;36:1440–3.
- Lowry OH, Rosebrough NJ, Farr AL, Randall RJ. Protein measurement with the Folin phenol reagent. J Biol Chem 1951;193:265–75.
- Ng F, Berk M, Dean O, Bush AI. Oxidative stress in psychiatric disorders: evidence base and therapeutic implications. Int J Neuropsychopharmacol 2008;11:851–76.
- Boskovic M, Grabnar I, Terzic T, Kores Plesnicar B, Vovk T. Oxidative stress in schizophrenia patients treated with long-acting haloperidol decanoate. Psychiatry Res 2013;210:761–8.
- Golembiowska K, Zylewska A. Agonists of A1 and A2A adenosine receptors attenuate methamphetamine-induced overflow of dopamine in rat striatum. Brain Res 1998;806:202–9.
- Boison D, Singer P, Shen HY, Feldon J, Yee BK. Adenosine hypothesis of schizophrenia – opportunities for pharmacotherapy. Neuropharmacology 2012;62:1527–43.
- Lara DR, Brunstein MG, Ghisolfi ES, Lobato MI, Belmonte-de-Abreu P, Souza DO. Allopurinol augmentation for poorly responsive schizophrenia. Int Clin Psychopharmacol 2001;16:235–7.
- Akhondzadeh S, Shasavand E, Jamilian H, Shabestari O, Kamalipour A. Dipyridamole in the treatment of schizophrenia: adenosine-dopamine receptor interactions. J Clin Pharm Ther 2000;25:131–7.
- Akhondzadeh S, Safarcherati A, Amini H. Beneficial antipsychotic effects of allopurinol as add-on therapy for schizophrenia: a double blind, randomized and placebo controlled trial. Prog Neuropsychopharmacol Biol Psychiatry 2005;29:253–9.
- Tsai MC, Liou CW, Lin TK, Lin IM, Huang TL. Changes in oxidative stress markers in patients with schizophrenia: the effect of antipsychotic drugs. Psychiatry Res 2013;209:284–90.
- Tarazi FI, Zhang K, Baldessarini RJ. Long-term effects of newer antipsychotic drugs on neuronal nitric oxide synthase in rat brain. Nitric Oxide 2002;7:297–300.
- Reinke A, Martins MR, Lima MS, Moreira JC, Dal-Pizzol F, Quevedo J. Haloperidol and clozapine, but not olanzapine, induces oxidative stress in rat brain. Neurosci Lett 2004;372:157–60.
- Parikh V, Khan MM, Mahadik SP. Differential effects of antipsychotics on expression of antioxidant enzymes and membrane lipid peroxidation in rat brain. J Psychiatr Res 2003;37:43–51.
- Pillai A, Parikh V, Terry AV Jr, Mahadik SP. Long-term antipsychotic treatments and crossover studies in rats: differential effects of typical and atypical agents on the expression of antioxidant enzymes and membrane lipid peroxidation in rat brain. J Psychiatr Res 2007;41:372–86.
- Kropp S, Kern V, Lange K, Degner D, Hajak G, Kornhuber J, et al. Oxidative stress during treatment with first- and second-generation antipsychotics. J Neuropsychiatry Clin Neurosci 2005;17:227–31.
- Quincozes-Santos A, Bobermin LD, Kleinkauf-Rocha J, Souza DO, Riesgo R, Goncalves CA, et al. Atypical neuroleptic risperidone modulates glial functions in C6 astroglial cells. Prog Neuropsychopharmacol Biol Psychiatry 2009;33:11–5.
- Taneli F, Pirildar S, Akdeniz F, Uyanik BS, Ari Z. Serum nitric oxide metabolite levels and the effect of antipsychotic therapy in schizophrenia. Arch Med Res 2004;35:401–5.