Abstract
Objective: To investigate the effect of Potentilla fulgens extract on lipid peroxidation and antioxidant status in male mice as a function of age.
Methods: Eighteen-month-old Swiss albino male mice were administered the dichloromethane-methanol extract of P. fulgens (250 mg/kg b.w.) on alternate days via intraperitoneal route for a period of 14 days. Lipid peroxidation and activities of catalase (CAT) and glutathione peroxidase (GPx1) in liver and kidney were measured and serum oxygen radical absorbance capacity (ORAC) assay was estimated. Phytochemical analysis of P. fulgens extract using high performance thin layer chromatography (HPTLC) was carried out with gallic acid, quercetin, catechin, and epicatechin as markers.
Results: Significant increase in level of thiobarbituric acid-reactive substances (TBARS), decreased GPx1, and CAT activities as well as reduction in ORAC were observed in 18-month-old mice as compared to that of 2-month-old mice. Treatment with P. fulgens extract significantly lowered TBARS level, ameliorated CAT, and GPx1 activities in liver and kidney and improved serum ORAC in aging mice. HPTLC studies revealed well resolved bands of P. fulgens extract containing epicatechin and catechin.
Discussion: This study showed that P. fulgens is a potent antioxidative agent, which can emerge as a promising candidate in alleviating the age-associated oxidative stress and related diseases.
Introduction
Aging is a complex biological phenomenon which involves progressive decline in different physiological functions of various tissues of living organisms.Citation1 Increased reactive oxygen species (ROS) generation during the aging process has been found to contribute to the accumulation of oxidative damage to cellular constituents.Citation2 The ‘oxidative stress theory’ states that a progressive and irreversible accumulation of oxidative damage caused by ROS impacts on critical aspects of the aging process and contributes to impaired physiological function, increased incidence of disease, and a reduction in life span.Citation3
Oxidative stress occurs when the rate of ROS generation exceeds the natural capacity of the cell for their removal, causing damages to cell membranes and DNA, inducing oxidation that causes membrane lipid peroxidation, decreased membrane fluidity, and DNA mutations. The homeostasis between ROS production and antioxidant defense system loses its control during aging process and this impaired oxidative stress tolerance kindles several signaling events like apoptosis.Citation4 According to Kregel and ZhangCitation3 the modulation of expression of various stress-response genes and the intracellular damage to macromolecules arise as the primary effect of oxidative stress, eliciting subsequent responses at cellular levels (e.g., inflammation, proliferation, apoptosis, and necrosis) that can stimulate additional ROS generation from endogenous sources. These ROS-induced changes at cellular levels can also lead to an integrated array of systemic responses that can impact, with the passage of time, on aging processes, as well as organ dysfunction, frailty, and age-related diseases.
Antioxidant intervention to ameliorate oxidative damage in aging and to delay the onset of age-related diseases has been approached by dietary supplementation of natural antioxidants and the use of phytochemicals.Citation5 Role of nutrition and specific dietary constituents in the prevention of diseases have gained increased attention where reportedly their bioactive phytochemical constituents, including flavonoids and phenolic compounds can alter the activity of several cell signaling pathways, which can lead to modulation of inflammatory processes, regulation of cytoprotective mechanisms, and regulation of cell growth.Citation6,Citation7
Potentilla fulgens L. of the Rosaceae family commonly found at higher altitudes (1500–2000 m MSL) of Khasi Hills, Meghalaya, India has been used as folk remedy. Traditionally, pieces of roots are chewed along with betel, composed of raw areca nut (Areca catechu), locally called Kwai, and betel leaf (Piper betel). It has been shown that roots of P. fulgens possess hypoglycemic and anti-hyperglycemic properties in mice,Citation8 affect the polyol pathway,Citation9 and have potential antioxidant activity in vitro systems.Citation10 In addition, P. fulgens was evaluated for its potent gastroprotective and antisecretory effects.Citation11 Jaitak et al.Citation12 reported the presence of antioxidant compounds epicatechin and a new biflavanoid (Potifulgene) in P. fulgens. Since, to the best of our knowledge, no scientific data have been reported on the antioxidant effect of P. fulgens on age-related oxidative stress, this study was undertaken to investigate the effect of dichloromethane-methanol extract of P. fulgens on lipid peroxidation, activities of the antioxidant enzymes (catalase (CAT), glutathione peroxidase (GPx1)) in liver and kidney of old mice and serum oxygen radical absorbance capacity (ORAC) levels, and to compare its effects with that of a standard antioxidant, vitamin C. In addition, phytochemical analysis of P. fulgens extract was performed using high performance thin layer chromatography (HPTLC).
Materials and methods
Chemicals
Glutathione reduced, 1,1,3,3-tetramethoxy propane (TMOP), 5,5-dithiobis-2-nitrobenzoic acid, 6-hydroxy-2,5,7,8-tetramethylchroman-2-carboxylic acid (trolox), beta-phycoerythrin, thiobarbituric acid, nitrocellulose membrane (0.045 µm pore size), 2,2´-azobis(2-aminopropane) dihydrochloride (AAPH), N,N,N′,N′-tetramethylenediamine (TEMED), 1,1-diphenyl-2-picrylhydrazyl (DPPH), beta-mercaptoethanol, catechin hydrate, gallic acid, epicatechin, and quercetin dihydrate were procured from Sigma-Aldrich (St Louis, USA). Primary antibodies for CAT, GPx1, and beta-actin were purchased from Santa Cruz Biotechnology Inc., USA. Rabbit-anti-mouse-IgG HRP conjugate and 3,3′,5,5′-tetramethylbenzidine/hydrogen peroxide (TMB/H2O2) were obtained from Bangalore Genei, India. Other chemicals and solvents used were of analytical grade obtained from SRL, Hi-media, and Rankem, India.
Plant material and extraction process
Potentilla fulgens was collected from Shillong peak area, East Khasi Hills District, Meghalaya, India and the plant specimen (voucher no. 464) was submitted and identified by herbarium curator Dr P.B. Gurung, Department of Botany, NEHU, Shillong.
The dried root powder of P. fulgens was extracted with dichloromethane-methanol solution (1:1, v/v) for 48 hours by maceration method by stirring at room temperature.Citation13 The extraction unit was protected from light to prevent polymerization of the secondary metabolites. The mixture was filtered and the filtrate was dried under vacuum in a rotary evaporator (Yamato RE800) yielding the crude dichloromethane-methanol extract. The dried mass obtained was used for the investigation.
Experimental animals
Healthy, male Swiss albino mice (BALB/c strain) of two age groups: (a) 2-months in age, weighing 20–25 g and (b) 18-months in age, weighing 40–45 g, procured from Pasteur Institute, Shillong, Meghalaya, were used for the study after approval from the Institutional Ethical Committee (IEC (Animal model)/NEHU, 09-10-13). Mice were housed in a room kept under controlled conditions with temperature maintained at 22°C on a 12-hour light/dark cycle (06:00–16:00 hours) and were fed with balanced mice feed obtained from Amrut Laboratory, Pune, India.
Experimental design
In this study, old (18-month) mice were divided into three subgroups (comprising six animals each): normal untreated, extract-treated groups which were administered the extract at the dose of 250 mg/kg of body weight on alternate days via i.p. route for a period of 14 days and vitamin C treated group in which old mice were treated with the standard antioxidant vitamin C at an equivalent dose of 250 mg/kg of body weight. The 2-month-old mice were used for comparison with the 18-month age group.
Preparation of serum and tissue homogenate
After the 14-day treatment period blood was obtained from retro-orbital sinus puncture drawn into a sample tube, kept in room temperature for 2 hours and was allowed to clot. Following clot formation, tubes were centrifuged at 870 × g at 4°C for 10 minutes to separate the serum. Serum was collected and stored at −80°C until use. Mice were sacrificed by cervical dislocation at a fixed time of the day (11:00 hour) and the liver and kidney tissues were quickly excised free of fat and connective tissues, washed in normal saline (0.9%), and blotted dry. For measurement of lipid peroxidation, a 10% (w/v) homogenate of individual liver and kidney tissue was prepared in ice-cold 1.15% KCl solution. The homogenates were centrifuged at 1000 × g for 10 minutes at 4°C. The pellet was discarded and supernatant was used to determine the thiobarbituric acid-reactive substances (TBARS) levels. For estimation of CAT and GPx1 activities, tissues were homogenized (10% w/v) in 50 mM potassium phosphate buffer, pH 7.4, and centrifuged at 18 000 × g for 15 minutes at 4°C. The pellet was discarded and the cytosolic supernatant was used as enzyme preparations. Protein content of the enzyme preparation was determined according to the dye-binding method of Bradford using bovine serum albumin as the standard.Citation14
Lipid peroxidation assay
Lipid peroxidation was estimated by measurement of TBARS according to the method of Ohkawa et al.Citation15 The pink chromogen produced by the reaction of thiobarbituric acid with malondialdehyde (MDA), a secondary product of lipid peroxidation was measured at 532 nm. TMOP was used as the standard, and the level of lipid peroxides was expressed as µmoles of MDA.
Measurement of oxygen radical absorbance capacity
ORAC was determined by measuring the inhibition of free radical action on the fluorescent compound, beta-phycoerythrin by the sample.Citation16 This inhibition can be correlated with the sample's antioxidant capacity. AAPH was used to generate peroxyl radicals. Excitation and emission wavelengths of beta-phycoerythrin were set at wavelengths 562 nm for excitation and 575 nm for emission. Trolox was used as the control standard. Antioxidant capacity of the sample was expressed as mM Trolox equivalents.
Assay of antioxidant enzyme activities
Catalase
CAT activity was measured according to the method of AebiCitation17 by determining the rate of degradation of hydrogen peroxide at 240 nm. The extinction co-efficient of 71/mM/cm was used for calculation. One unit is defined as 1 mmol of H2O2 consumed/minute and the specific activity is reported as units/mg protein.
Glutathione peroxidase
GPx1 was assayed by measuring the amount of reduced glutathione (GSH) at 412 nm according to the method of Rotruck et al.Citation18 The activity of GPx1 is expressed as µmoles of GSH utilized/minute/mg protein (units/mg protein).
Western blot analysis
Protein levels of CAT and GPx1 were detected by Western blot using 12% sodium dodecyl sulfate polyacrylamide gel electrophoresis (SDS-PAGE), where an aliquot of sample proteins (20 µl) containing 30 µg protein for CAT detection and 50 µg protein for GPx1 detection were used. Following electrophoresis, the proteins in the gel were transferred to a nitrocellulose membrane using Bio-Rad Mini trans-blot electrophoretic unit at 100 V (constant) for 60 minutes. The electroblotted membrane was transferred in blocking solution containing 5% non-fat dry milk in Tris buffer saline (TBS) for 45 minutes at room temperature. After washing in Tween Tris buffer saline (TTBS), the membrane was incubated overnight with primary antibodies for CAT and GPx1 or anti-beta-actin antibody solution (1:1000). Beta-actin was used as a loading control. The membrane was washed twice in TTBS to remove unbound antibodies and incubated with the secondary antibody, rabbit-anti-mouse-IgG conjugated to horseradish peroxidase (1:500) for 3 hours followed by the addition of the substrate (TMB/H2O2) for color development. The reaction was stopped by washing the membrane in double distilled H2O and photographed using an HP Scan jet 7400C. The blots were quantified densitometrically using Kodak Digital Science 1D Image Analysis Software, Version 3.0. The total protein content of liver and kidney cytosolic supernatant was estimated by the method of Bradford.Citation14
High performance thin layer chromatography procedure
Dichloromethane-methanol extract of P. fulgens was reconstituted in absolute methanol (1 mg/ml, 7 µl) and applied on a pre-coated HPTLC plate (silica gel 60 F254, Merck) using a Camag Linomat 5 system and application was programed through WinCATS planar chromatography manager (Version 1.4.8). Standard solutions (0.1 mg/ml, 2 µl) of gallic acid, quercetin dehydrate, epicatechin, and catechin hydrate prepared in absolute methanol were run as qualitative markers. The mobile phase for developing the chromatogram was composed of n-hexane/toluene/ethyl acetate/formic acid mixture (2:8:8:2; v/v). The developed plate was air-dried and photo-documented with a Camag TLC visualizer under ultraviolet light at 254 and 366 nm before and after derivatization with Marquis reagent. Another HPTLC separation was carried out by treatment with 0.25 mM DPPH solution which was used for derivatization to evaluate the free radical scavenging activity of the components separated.
Statistical analysis
Values were expressed as mean ± SD in each group. Data obtained from different sets were analyzed by one-way analysis of variance (ANOVA) followed by Bonferroni's multiple comparison test (using GraphPad Prism 6 Software). A P value <0.05 was considered as significant.
Results
Effect of P. fulgens extract on lipid peroxidation
TBARS level, an index of lipid peroxidation, showed a significant increase in liver and kidney of old mice (18-month) compared to the young (2-month) control mice (Fig. ). The elevation in TBARS level of old mice was 155% (P < 0.001) in liver and 153% (P < 0.001) in kidney. Administration of P. fulgens extract (250 mg/kg of body weight) to 18-month-old mice significantly lowered TBARS level by 60% (P < 0.001) in liver and 50% (P < 0.01) in kidney, from that of the 18-month-old control mice. Treatment with vitamin C decreased lipid peroxidation level by 70% (P < 0.001) in liver and 72% (P < 0.001) in kidney.
Effect of P. fulgens extract on serum ORAC levels
Graphs for blank and serum showed exponential decay curves as the fluorescence of beta-PE was progressively impaired by free radical attack from AAPH (Fig. A). Administration of P. fulgens extract considerably slowed the rate of fluorescence decay in the 18-month-old treated mice when compared to the control group. Correspondingly, the ORAC value, expressed as the net area under the curve for each sample was shown to decrease in the serum of 18-month-old group when compared to the 2-month-old control group (Fig. B). The reduction was observed to be 47% (P < 0.05) from that of the young control. Eighteen-month-old mice treated with P. fulgens extract exhibited a significant increase by 116% (P < 0.01) in the ORAC value in comparison to the age-matched control, its effect was found to be similar to that shown by vitamin C.
Figure 2 (A) Effect of P. fulgens extract on the fluorescence decay of beta-PE in serum of 18-month-old mice. (B) Effect of P. fulgens extract on the oxygen radical absorbance capacity of serum of control 2- and 18-month-old and treated 18-month-old mice. Values are mean ± SD, n = 6, ###P < 0.001 compared to 2-month control group (YC); **P < 0.01, ***P < 0.001 compared to 18-month control group (OC); vit C: vitamin C.
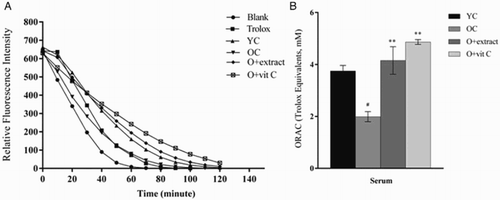
Effect of P. fulgens extract on antioxidant enzymes
Catalase
Eighteen-month-old control mice showed a significant decline in the activity of CAT in liver and kidney when compared to the young (2-month) control mice (Fig. A). CAT activity in liver was reduced by 33% (P < 0.001) and in kidney by 45% (P < 0.001) as compared to the old control group. Western blot analysis of the CAT protein (Fig. B) in both tissues confirmed lowered activity in older mice when compared to younger ones (P < 0.01). Following treatment with P. fulgens extract to 18-month-old mice, CAT showed a marked increase in its activity by 241% (P < 0.001) in liver and 319% (P < 0.001) in kidney from that of the age-matched control group. Effect of treatment with vitamin C was observed in both tissues with an increase in CAT activity by 195% (P < 0.01) in liver and 144% (P < 0.01) in kidney. Protein levels were significantly increased in liver (P < 0.01) and kidney (P < 0.05) when treated with P. fulgens extract which corroborated with the significant increase in enzyme activity in these tissues of treated old mice. However, vitamin C raised protein amount of CAT to a higher degree than when compared to its activity in the kidney tissue.
Figure 3 (A) Effect of P. fulgens extract on CAT activity in liver and kidney of 18-month-old mice. (B) Western blot analysis of CAT protein from (a) liver and (b) kidney of 2-month control group (YC), 18-month control group (OC) and 18-month-old mice treated with P. fulgens extract and vitamin C. (C) The relative intensity of the western blot determined by densitometric analysis (KDS-I software) after normalization to actin. Values are mean ± SD, n = 6, ###P < 0.001 compared to 2-month control group (YC); **P < 0.01, ***P < 0.001 compared to 18-month control group (OC); vit C: vitamin C.
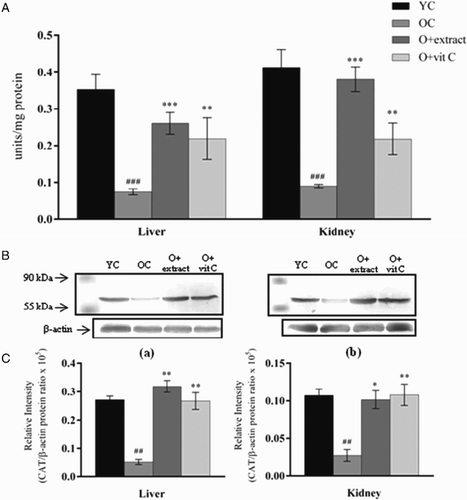
Glutathione peroxidase
A significant decrease in GPx1 activity in liver and kidney tissues of 18-month-old mice was observed in comparison to the young (2-month) control mice (Fig. A). The activity was lowered by 59% (P < 0.001) in liver and 61% (P < 0.001) in kidney. Immunoblot analysis of GPx1 protein from liver and kidney (Fig. B) showed a marked decrement in the protein expression level of 18-month-old mice as compared to the young control (P < 0.01) corroborating with the observed decrease in the enzyme activity. Eighteen-month-old mice supplemented with P. fulgens extract showed significant elevation in GPx1 activity in liver by 144% (P < 0.001) and in kidney by 82% (P < 0.01) while vitamin C significantly increased GPx1 activity in liver and kidney by 92% (P < 0.001) and 53% (P < 0.05), respectively. Elevation of GPx1 protein level in liver (P < 0.01) and kidney (P < 0.05) after treatment with P. fulgens extract supported the increase in enzyme activity observed in extract-treated old mice although extent of increase was observed to be higher for enzyme activity in case of liver, and protein level in the case of kidney.
Figure 4 (A) Effect of P. fulgens extract on GPx activity in liver and kidney of 18-month-old mice. (B) Western blot analysis of GPx protein from (a) liver and (b) kidney of 2-month control group (YC), 18-month control group (OC) and 18-month-old mice treated with P. fulgens extract and vitamin C. (C) The relative intensity of the western blot determined by densitometric analysis (KDS-I software) after normalization to actin. Values are mean ± SD, n = 6, ###P < 0.001, ##P < 0.01 compared to 2-month control group (YC); **P < 0.01, ***P < 0.001 compared to 18-month control group (OC); vit C: vitamin C.
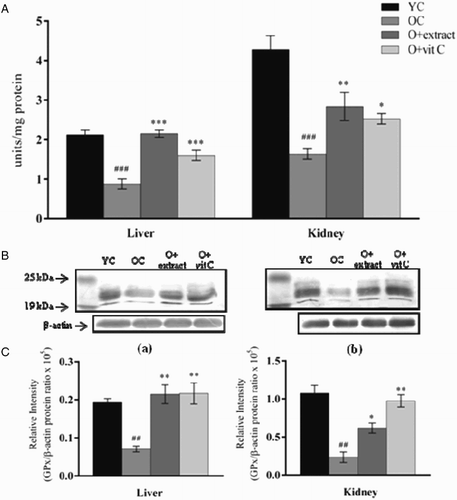
HPTLC analysis
HPTLC studies revealed well-resolved bands of P. fulgens extract containing epicatechin and catechin (Fig. B) which corresponded to the reference standards tested. These bands were not clearly visible before staining with Marquis reagent (Fig. A). Presence of chemical entities indicated by dark colored bands following staining with Marquis reagent was observed (first run). Further, post chromatographic derivatization using 0.25 mM DPPH solution in a separate run under identical conditions as the first run indicated scavenging activities of these chemical entity(s) by the appearance of white yellow band on a light pink purple background (Fig. C). These phyto-constituents not yet identified together with the identified and known antioxidants-epicatechin and catechin may be synergistically responsible for the bioactivity of dichloromethane-methanol extract of P. fulgens.
Figure 5 Developed HPTLC plates (A) without staining at lambda-366 nm, (B) stained with Marquis reagent at 366 nm, and (C) stained with 0.25 mM DPPH solution in white light. Lane 1 – gallic acid, Rf – 0.23 and quercetin, Rf – 0.38; Lane 2 – P. fulgens extract; Lane 3 – epicatechin, Rf – 0.16; Lane 4 – catechin, Rf – 0.18.
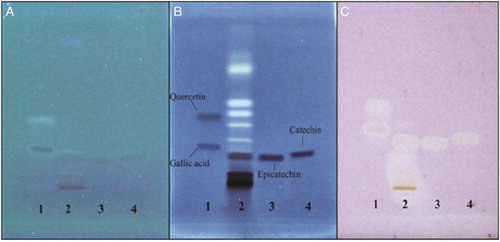
Discussion
For many years, it has been proposed that the lifelong production and accumulation of free radicals as byproducts of oxidative metabolism is the basis of aging process.Citation19 The view that lipid peroxidation may be the key process contributing to free radical-induced damage is reinforced by the fact that oxidized lipid residues are major components of lipofuscin, the fluorescent pigment that accumulates with age in most tissues.Citation20 Protein carbonylation, a consequence of oxidative damage to protein, has been shown to consistently increase with advanced age and is implicated in age-related degenerative diseases. Protein oxidation through reactive protein carbonyl assessment provides a good indication of overall oxidation and it also reflects covalent adduct formation from lipid peroxidation products whereby several oxidative pathways and products from lipid peroxidation and glycooxidation can generate carbonyls from proteins.Citation21 Our study showed that the levels of TBARS in the liver and kidney of 18-month-old mice increased significantly as compared to the 2-month-old mice, which is indicative of an increased oxidative stress and enhanced ROS formation in senescent mice.Citation22,Citation23 It has been hypothesized that age-related peroxidation of membrane lipids brings about fluidity changes in the membranes, which in turn disrupt vital functions, such as signal transduction or the maintenance of selective permeability to ions.Citation24 Increase of TBARS might be due to depletion of alpha-tocopherol and ascorbic acid content in aged mice where it has been reported that an age-dependent decline in ascorbic acid and alpha-tocopherol occurs in both plasma and tissues of rodentsCitation25 since both react with active oxygen radicals such as LOO·, LO· and HO· in biological systems and protects unsaturated lipids from peroxidation. Treatment with P. fulgens extract at 250 mg/kg of body weight reversed TBARS buildup in the liver and kidney tissues of aging mice where TBARS level was significantly brought down even below the normal levels of young mice, conferring the chelating and free radical quenching nature of the extractCitation10 which is comparable to an equivalent dose of vitamin C.
Vitamin C, also known as ascorbic acid, is a naturally occurring water-soluble antioxidant. It acts as a scavenger of ROS and RNOS or at least alleviate the deleterious effects caused by them, and in that respect it can prevent the damaging effects of oxidative stress. It also acts as a cofactor maintaining the activity of a number of enzymes by keeping the metal ions in the reduced state and has a role in stress resistance.Citation26 It works synergistically with vitamin E to quench free radicals and also regenerates the reduced form of vitamin E.Citation27 Vitamin E or tocopherols are a class of lipid-soluble antioxidants which form essential components of the human diet because they perform numerous crucial functions, including quenching and scavenging of GSH and ascorbate, which accumulate in response to oxidative stress. Alpha-tocopherols interact with the polyunsaturated acyl groups of lipids, stabilize membranes, and scavenge and quench various ROS and lipid-soluble byproducts of oxidative stress, thus acting as a powerful terminator of lipid peroxidation.Citation28 Beta-carotene (provitamin A), a fat soluble member of carotenoids, is assumed to stimulate the immune system and cancer suppressor genes as well as deregulate oncogenes and block tumor angiogenesis.Citation29 Lycopene is considered the carotenoid with the greatest capacity to eliminate the singlet oxygen. Studies have demonstrated that lycopene protects lipid molecules, low-density lipoproteins, proteins, and DNA against free radical attack, playing an essential role in the protection against diseases.Citation30
Total level of antioxidants in serum of old mice before and after treatment of extract was determined using the ORAC method. The ORAC assay has provided significant information regarding the antioxidant capacity of various biological samples, from pure compounds such as melatonin, dopamine, and flavonoids to complex matrices such as tea, fruits, vegetables, herbs, and animal tissues.Citation31 Results showed that ORAC in serum of 18-month-old mice was lowered which is indicative of a low antioxidant level and/or high ROS level in comparison to the 2-month-old group. Aging is accompanied by a decline in many non-enzymatic endogenous antioxidants in the plasma and tissues of rodents which could have contributed to low ORAC in old mice.Citation25 Administration of P. fulgens elevated the ORAC levels in 18-month-old mice in comparison to the age-matched control. In addition, treatment with standard antioxidant vitamin C revealed highest ORAC levels in the old mice. Vitamin C is effective as a vitamin supplement with ORAC value of 1890 units per gram.Citation32 It has been stated that young and middle-aged people may be able to reduce risk of diseases of aging, including senility, simply by adding high-ORAC foods to their diets.Citation33
CAT, one of the H2O2 metabolizing enzymes, is considered to be an important regulator of oxidative stress.Citation34 In conformity with other studies,Citation23,Citation35 our finding shows that CAT activity in the liver and kidney tissues was reduced in old mice (Fig. ). Schriner et al.Citation36 have shown that transgenic mice overexpressing human CAT in mitochondria had an increase in lifespan and this extension of lifespan was accompanied by reduction of H2O2 production. GPx plays a predominant role in the detoxification of peroxides from cells and tissues.Citation37 GPx1 uses glutathione (GSH) as a substrate to reduce hydrogen peroxide to water while a majority of other GPx isozymes catalyze the GSH-dependent reduction of lipid hydroperoxides to alcohol, thus indicating that normal GSH levels are required to maintain GPx activity. GSH, in itself is a powerful endogenous antioxidant with multiple functions wherein it maintains activities of glycolytic and antioxidant enzymes, and keeps other antioxidant compounds in the reduced state. It is a major thiol source that maintains essential redox couple in the cells.Citation21 Low GSH levels may negatively impact on the activity of GSH-dependent enzymes such as GPx, thereby, contributing to oxidative stress which plays a key role in aging and pathogenesis of neurodegenerative diseases. In our study, its activity was found to effectively decrease in the liver and kidney tissues of old mice. A study by Shih and YenCitation38 demonstrates that the relative expressions of GPx and CAT exhibit a profound decline in aged rats. Kishido et al.Citation39 have described the decreased activity of GPx as an important contributor of brain dysfunction in aging mice. Dichloromethane-methanol extract of P. fulgens ameliorated the activities of antioxidant enzymes CAT and GPx1 in liver and kidney of 18-month-old mice. While protein levels increased mostly in parallel to the activities of CAT and GPx1 in liver tissue after extract and vitamin C administration, however, higher elevation in CAT and GPx1 protein levels and comparatively lower increment of CAT and GPx1 activity in kidney after vitamin C treatment was observed. This suggests that vitamin C could be exerting its action on these enzymes in a tissue-specific manner regulating at the level of transcription, translation, or activity level, as demonstrated in another study by Sadi et al.Citation40 Further, the extract exerted a higher effect on GPx1 activity than protein level in kidney tissue which may suggest a role of post-translational modification given that the presence of other chemical entities in the extract may influence the enzyme also through other mechanisms. Potentilla fulgens extract afforded protection as evidenced by decreased TBARS and the near normal activities of these enzymatic antioxidants observed, which may be due to decreased free radical generation and increased antioxidant defenses. Veglioglu et al.Citation41 established that antioxidant activity of many compounds of botanical origin is proportional to the total phenolic content (TPC), thereby suggesting a causative relationship between TPC and antioxidant activity. HPTLC studies showed the presence of epicatechin and catechin in the dichloromethane-methanol extract of P. fulgens and these compounds could be part of the chemical entity(s) responsible for the bioactivity of dichloromethane-methanol extract of P. fulgens. Previous studies have shown the presence of these compounds gallic acid, epicatechin, and catechin in the butanol extract of P. fulgens using NMR.Citation12,Citation42 Gallic acid which was not clearly visible in Lane 2 (Fig. B) could be due to less concentration of this compound present in the dichloromethane-methanol extract of P. fulgens. In this paper we are reporting the presence of these compounds using HPTLC. Polyphenols including flavonoids interact with different membrane components and as a consequence of direct interaction with membrane lipids, these bioactive compounds can alter membrane physical properties and membrane-associated functions which can affect the occurrence and extent of deleterious events like lipid oxidation and membrane disruption.Citation43 The molecular mechanisms for the antioxidative properties of the plant extract may not merely rely on a direct radical scavenging activity but also its polyphenols may function at several cellular levels, including modulation of enzymatic activitiesCitation44,Citation45 and regulation of signaling pathways with implications for cell survival and death,Citation46 thus possibly preventing apoptosis and protect cells from the damaging effect of oxygen radicals.
Conclusion
This study indicates that Potentilla fulgens could be a candidate to suppress age-induced damage by protecting against oxidative stress and by increasing antioxidant defenses. This study also calls attention to the need of further biochemical investigations of the plant constituents to assess the efficacy of the plant and to determine the underlying mechanism of its action in the prevention and treatment of age-related diseases.
Disclaimer statements
Contributors None.
Funding UGC funded University with Potential for Excellence (UPE) program for NEHU, Department of Biotechnology (DBT), Department of Science and Technology (DST), New Delhi, India.
Conflicts of interest None.
Ethics approval Institutional ethical approval obtained from the Institutional Ethical Committee (IEC (Animal model)/NEHU, 09-10-13).
Acknowledgments
We thank Mr H. Jasha, Department of Chemistry, NEHU for the plant extract preparation; UGC funded UPE program for NEHU, DBT, DST through FIST, and the Department of Biochemistry, North-Eastern Hill University, Shillong, India for providing facilities.
References
- Harman D. Aging: overview. Ann NY Acad Sci 2001;928:1–21. doi: 10.1111/j.1749-6632.2001.tb05631.x
- Weinert BT, Timiras PS. Physiology of aging invited review: theories of aging. J Appl Physiol 2003;95:1706–16. doi: 10.1152/japplphysiol.00288.2003
- Kregel KC, Zhang HJ. An integrated view of oxidative stress in aging: basic mechanisms, functional effects, and pathological considerations. Am J Physiol Regul Integr Comp Physiol 2007;292:R18–R36. doi: 10.1152/ajpregu.00327.2006
- Li J, Holbrook NJ. Common mechanism for decline in oxidative stress tolerance and proliferation with aging. Free Radic Biol Mol 2003;35:292–9. doi: 10.1016/S0891-5849(03)00308-3
- Halliwell B. Establishing the significance and optimal intake of dietary antioxidants: the biomarker concept. Nutr Rev 1999;57:104–13. doi: 10.1111/j.1753-4887.1999.tb06933.x
- Harborne JB. Nature, distribution and function of plant flavonoids. In: Cody V, Middleton E, Jr, Harborne JB, (eds.) Plant flavonoids in biology and medicine: biochemical, pharmacological and structure activity relationship. New York: Alan R Liss Inc; 1986. p. 15–24.
- Devasagayam TPA, Tilak JC, Boloor KK. Review: free radicals and antioxidants in human health. J Assoc Physicians India 2004;52:794–804.
- Syiem D, Syngai G, Khup PZ, Khongwir BS, Kharbuli B, Kayang H. Hypoglycemic effects of Potentilla fulgens in normal and alloxan-induced diabetic mice. J Ethnopharmacol 2002;83:55–61. doi: 10.1016/S0378-8741(02)00190-3
- Syiem D, Majaw S. Effect of Potentilla fulgens L. methanolic extract on Sorbitol dehydrogenase in normal and alloxan-induced diabetic mice. Pharmacologyonline 2010;2:671–80.
- Syiem D, Sharma R, Saio V. In vitro study of the antioxidant potential of some traditionally used medicinal plants of North-East India and assessment of their total phenolic content. Pharmacologyonline 2009;3:952–65.
- Laloo D, Prasad SK, Krishnamurthy S, Hemalath S. Gastroprotective activity of ethanolic root extract of Potentilla fulgens Wall. ex Hook. J Ethnopharmacol 2013;146:505–14. doi: 10.1016/j.jep.2013.01.015
- Jaitak V, Sharma K, Kalia K, Kumar N, Singh HP, Kaul VK, et al.Antioxidant activity of Potentilla fulgens: an alpine plant of western Himalayas. J Food Compos Anal 2010;23:142–7. doi: 10.1016/j.jfca.2009.02.013
- Sevimli-Gur C, Akgun IH, Deliloglu-Gurhan I, Korkmaz KS, Bedir E. Cytotoxic naphthoquinones from Alkanna cappadocica. J Nat Prod 2010;73:860–4. doi: 10.1021/np900778j
- Ohkawa H, Ohishi N, Yagi K. Assay of lipid peroxides in animal tissues by thiobarbituric acid reaction. Anal Biochem 1979;95:351–8. doi: 10.1016/0003-2697(79)90738-3
- Bradford MM. A rapid and sensitive method for quantitation of microgram quantities of protein utilizing the principle of protein-dye-binding. Anal Biochem 1976;72:248–54. doi: 10.1016/0003-2697(76)90527-3
- Cao G, Prior RL. Comparison of different analytical methods for assessing total antioxidant capacity of human serum. Clin Chem 1998;44:1309–15.
- Aebi H. Catalase in vitro. In Methods in enzymology, Vol. 105. New York: Academy Press; 1984. p. 121–6.
- Rotruck JT, Pope AL, Ganther HE. Selenium: biochemical role as a component of glutathione peroxidase. Science 1973;179:588–90. doi: 10.1126/science.179.4073.588
- Harman D. The biologic clock: the mitochondria? J Am Geriatr Soc 1972;20:145–7. doi: 10.1111/j.1532-5415.1972.tb00787.x
- Brunk UT, Jones CB, Sohal RS. A novel hypothesis of lipofuscinogenesis and cellular aging based on interactions between oxidative stress and autophagocytosis. Mutat Res 1992;275:395–403. doi: 10.1016/0921-8734(92)90042-N
- Pollack M, Leeuwenburgh C. Molecular mechanisms of oxidative stress in aging: free radicals, aging, antioxidants and disease. In: Sen CK, Packer L, Hanninen O, (eds.) Handbook of oxidants and antioxidants in exercise. Amsterdam: Elsevier Science B.V.; 1999. p. 881–923.
- Kaur J, Sharma D, Singh R. Acetyl-L-carnitine enhances NaþKþ ATPase glutathiones-transferase and multiple unit activity and reduces lipid peroxidation and lipofuscin concentration in aged rat brain regions. Neurosci Lett 2001;301:1–4. doi: 10.1016/S0304-3940(01)01576-2
- Farahmand SK, Samini F, Samini M, Samarghandian S. Safranal ameliorates antioxidant enzymes and suppresses lipid peroxidation and nitric oxide formation in aged male rat liver. Biogerontology 2013;14:63–71. doi: 10.1007/s10522-012-9409-0
- Yu BP, Suescun EA, Yang SY. Effect of age-related lipid peroxidation on membrane fluidity and phospholipase A2: modulation by dietary restriction. Mech Ageing Dev 1992;65:17–33. doi: 10.1016/0047-6374(92)90123-U
- Michels AJ, Hagen TM. Vitamin C status declines with age. In: Asard H, May JM, Smirnoff N, (eds.) Vitamin C-functions and biochemistry in animals and plants. London: Taylor & Francis; 2004. p. 203–27.
- Kojo S. Vitamin C: basic metabolism and its function as an index of oxidative stress. Curr Med Chem 2004;11:1041–64. doi: 10.2174/0929867043455567
- Li Y, Schellhorn HE. New developments and novel therapeutic perspectives for vitamin C. J Nutr 2007;137(10):2171–84.
- Kiffin R, Bandyopadhyay U, Cuervo AM. Oxidative stress and autophagy. Antioxid Redox Signal 2006;8:152–62. doi: 10.1089/ars.2006.8.152
- Manetta A, Schubbert T, Chapman J, Schell MJ, Peng YM, Liao SY, et al.Beta-carotene treatment of cervical intraepithelial neoplasia: a phase II study. Cancer Epidemiol Biomarkers Prev 1996;5(11):929–32.
- Teodoro AJ, Oliveira FL, Martins NB, Maia GA, Martucci RB, Borojevic R. Effect of lycopene on cell viability and cell cycle progression in human cancer cell lines. Cancer Cell Int 2012;12:36–44. doi: 10.1186/1475-2867-12-36
- Cao G, Prior RL. Comparison of different analytical methods for assessing total antioxidant capacity of human serum. Clin Chem 1998;44:1309–15.
- USDA (United States Department of Agriculture). [Accessed 20 August 2013]. Available from: www.ars.usda.gov/IS/pr/1999/990208.htm.
- Horn FP. High-ORAC Foods May Slow Aging, 1999. [Accessed 17 July 2013]. Available from: http://www.ars.usda.gov/is/AR/archive/feb99/aging0299.htm.
- D'souza A, Kurien BT, Rodgers R, Shenoi J, Kurono S, Matsumoto H, et al. Detection of catalase as a major protein target of the lipid peroxidation product 4-HNE and the lack of its genetic association as a risk factor in SLE. BMC Med Genet 2008;9:62. doi: 10.1186/1471-2350-9-62
- Tian L, Cai Q, Wei H. Alterations of antioxidant enzymes and oxidative damage to macromolecules in different organs of rats during aging. Free Radic Biol Med 1998;24:1477–84. doi: 10.1016/S0891-5849(98)00025-2
- Schriner SE, Linford NJ, Martin GM, Treuting P, Ogburn CE, Emond M, et al. Extension of murine life span by overexpression of catalase targeted to mitochondria. Science 2005;308:1909–11. doi: 10.1126/science.1106653
- Raes M, Michiels C, Remacle J. Comparative study of the enzymatic defense systems against oxygen-derived free radicals: the key role of glutathione peroxidase. Free Radic Biol Med 1987;3:3–7. doi: 10.1016/0891-5849(87)90032-3
- Shih PH, Yen GC. Differential expressions of antioxidant status in aging rats: the role of transcriptional factor Nrf2 and MAPK signaling pathway. Biogerontology 2007;8:71–80. doi: 10.1007/s10522-006-9033-y
- Kishido T, Unno K, Yoshida H, Choba D, Fukutomi R, Asahina S, et al. Decline in glutathione peroxidase activity is a reason for brain senescence: consumption of green tea catechin prevents the decline in its activity and protein oxidative damage in ageing mouse brain. Biogerontology 2007;8(4):423–30. doi: 10.1007/s10522-007-9085-7
- Sadi G, Güray T. Gene expressions of Mn-SOD and GPx-1 in streptozotocin-induced diabetes: effect of antioxidants. Mol Cell Biochem 2009;327(1–2):127–34. doi: 10.1007/s11010-009-0050-4
- Veglioglu YS, Mazza G, Gao L, Oomah BD. Antioxidant activity and total phenolics in selected fruits, vegetables and grain products. J Agric Food Chem 1998;46:4113–7. doi: 10.1021/jf9801973
- Choudhary A, Mittal AK, Radhika M, Tripathy D, Chatterjee A, Banerjee UC, et al. Two new stereoisomeric antioxidant triterpenes from Potentilla fulgens. Fitoterapia 2013;91:290–7. doi: 10.1016/j.fitote.2013.09.008
- Verstraeten SV, Fraga CG, Oteiza PI. Flavonoid–membrane interactions: consequences for biological actions. In: Fraga CG, (ed.) Plant phenolics and human health: biochemistry, nutrition and pharmacology. New Jersey: John Wiley & Sons, Inc; 2010. p. 107–35.
- Halliwell B, Gutteridge JMC. Free radicals in biology and medicine. London: Oxford University Press; 1999.
- Williams RJ, Spencer JPE, Rice-Evans C. Flavonoids: antioxidants or signalling molecules? Free Radic Biol Med 2004;36:838–49. doi: 10.1016/j.freeradbiomed.2004.01.001
- Aoki TM, Kataoka HH, Shimamura MH. NF-κB is a key mediator of cerebral aneurysm formation. Circulation 2007;116:2830–40. doi: 10.1161/CIRCULATIONAHA.107.728303