Abstract
Objectives
Exposure to high altitudes is associated with oxidative cellular damage due to the increased level of reactive oxygen and nitrogen species and altered activity of antioxidant systems. Subjects were submitted to prolonged hypoxia, to evaluate changes in mitochondrial enzyme activities of monocytes and their attenuation by supplementation with antioxidants.
Methods
Twelve subjects were randomly assigned to receive antioxidant supplements or placebo prior to and during an expedition to Pik Lenin (7145 m). Monocytes were isolated from blood samples to determine the activity of mitochondrial enzymes cytochrome c oxidase and citrate synthase at 490 m (baseline) and at the altitudes of 3550 m, 4590 m, and 5530 m.
Results
An increase in citrate synthase activity at all altitudes levels was observed. Hypoxia induced an increase in the activity of cytochrome c oxidase only at 4590 m. Neither citrate synthase activity nor cytochrome c oxidase activity differed between the subjects receiving antioxidant supplements and those receiving placebo.
Conclusions
Hypoxia leads to an increase in citrate synthase activity of monocyte mitochondria as a marker of mitochondrial mass, which is not modified by antioxidant supplementation. The increase in mitochondrial mass may represent a compensatory mechanism to preserve oxidative phosphorylation of monocytes at high altitudes.
Objectives
Various physiological changes involving different organ systems occur during exposure and acclimatization to hypobaric hypoxia in the context of a high-altitude sojourn.Citation1 The adjustments required to maintain homeostasis in hypoxic conditions lead to the activation of sympathoadrenal pathwaysCitation2,Citation3 which can influence the physiologic and metabolic adaptations of the immune systemCitation4 and may modify innate defense mechanisms against infection.Citation5,Citation6 Exposure to hypoxia in the context of a stay at high altitudes is also associated with an increase in oxidative cellular damage due to the increased level of reactive oxygen and nitrogen species (RONS) production and altered activity of antioxidant systemsCitation7–Citation9
Cellular hypoxia is also a fundamental mechanism of injury in the critically ill, and it has been suggested that the physiological and pathophysiological responses to hypobaric hypoxia may be similar to responses seen in critical illness.Citation10,Citation11 Circulating immune cells play an important role in the pathophysiology of infections, and prolonged systemic infection has been associated with the down-regulation of immune-cell activityCitation12,Citation13 and mitochondrial abnormalities in blood cells.Citation14 Depleted levels of reduced glutathione, an important intra-mitochondrial antioxidant, in combination with increased generation of RONS, inhibit oxidative phosphorylation and adenosine-5'-triphosphate (ATP) generation. This acquired intrinsic disturbance in cellular energy metabolism contributes to reduced activity of mitochondrial electron transport chain enzyme complexes and impaired ATP biosynthesis.Citation15,Citation16 Bioenergetic failure of human peripheral monocytes due to mitochondrial dysfunction has been reported in patients in conditions associated with increased oxidative stress.Citation17 A decrease in reduced glutathione levels and an increase in oxidized glutathione concentration have also been reported in blood cells during exposure to simulated high altitudes.Citation18
As an increase in oxidative stress occurs in both conditions – critical illness and exposure to hypobaric hypoxia – we hypothesized that changes occurring in the mitochondrial function of immune cells under high-altitude conditions would be similar to changes observed in critically ill patients. The aim of the study was to investigate changes in mitochondrial enzyme activities of peripheral blood monocytes (PBM) in healthy volunteers subjected to prolonged hypoxic conditions and oxidative stress in the context of a high-altitude expedition to climb Pik Lenin (7145 m) in Kirgizstan. Additionally, the effects of dietary supplementation of antioxidants on mitochondrial function of blood cells were to be evaluated. We hypothesized that prolonged hypobaric hypoxia and oxidative stress would lead to down-regulation of mitochondrial function of PBM assessed as mitochondrial enzyme activities, and that dietary supplementation of antioxidants attenuates the effects of hypoxia and oxidative stress on mitochondrial function.
Methods
Participants
A total of 12 volunteers living at low altitudes were included in the study. The subjects were required to be adult (between the ages of 18 and 65 years), healthy, well-trained mountaineers with previous trekking experience and basic mountaineering skills. The sample size was determined by the maximum processable number of blood samples at the high-altitude study sites. Exclusion criteria were any type of cardiac, renal, hepatic or respiratory diseases or acute or chronic infectious or inflammatory disease, presence of any metabolic disorder, regular intake of medications, and the development of high-altitude pulmonary edema or high-altitude cerebral edema after rapid ascent to altitudes below 3500 m.
Procedures and investigations
This was a prospective, single-center, randomized, double-blind, placebo-controlled study. Using a computer-based randomized allocation procedure, subjects were assigned in a double-blind fashion to receive either antioxidant dietary supplements or placebo over the period of 2 months prior to departure until completion of the climb. The antioxidant dietary supplements consisted of 800 I.E. vitamin E, 1000 mg vitamin C, 200,000 I.E. vitamin A, and 600 mg N-acetylcysteine daily. Antioxidant dietary supplements and placebo of identical appearance were provided by the state pharmacy of the canton of Aarau (Spitalapotheke, Kantonsspital Aarau). Study group allocation was performed by the pharmacy; allocation was not revealed to the subjects or the investigators until completion of the data collection and the processing of the blood samples. Serial blood samples were collected at 490 m (baseline) and at altitude study sites located at 3550 m (expedition day 4), 4590 m (day 7) and 5530 m (day 11) above sea level. At every altitude, arterial oxygen saturation was determined by finger pulse oximetry (Onyx 9500, Nonin Medical, Inc., Plymouth, MA, USA).
Blood samples were processed immediately after collection at baseline and altitude study sites. CD14+ cells were isolated directly from whole blood with Dynabeads® CD14 (Invitrogen Dynal AS, Oslo, Norway); 125 µl of Dynabeads (4×108/ml) was used per 1.5 ml blood. The Dynabeads and the whole blood were incubated in 1.5 ml tubes for 20 minutes with gentle rotation and afterwards the tubes were placed in a magnet for 2 minutes (MPC 6; Dynal). For positive isolation of PBM, the supernatants were discarded and the beads with attached CD14+ cells were washed three times by resuspending in phosphate buffer saline, and the beads with attached cells were frozen. During the transport from the study sites at high altitude to the laboratory in Switzerland, the blood samples were kept at −30°C. During the rapid descent to the basecamp, the samples were kept in a pre-cooled insulation container. For helicopter transport from the basecamp to the nearest hospital (Bishkek), the samples were placed in a refrigerator. A commercial refrigerated transport service with standard temperature monitoring was used to transfer the samples from Kyrgyzstan to the laboratory in Switzerland. After being transported to the laboratory, the beads were lysed by the addition of 100 μl of CelLytic™ MT cell lysis reagent (Sigma-Aldrich, St Louis, MO, USA, C3228). The protein concentration of monocyte lysates was determined with the Quant-iTM assay kit and read with the Qubit-TM fluorometer (Invitrogen, Basel, Switzerland). The lysates were frozen at −80°C for enzymatic activity measurements.
Primary outcome
The activity of citrate synthase of monocyte lysates (10 μg protein/assay) was measured using the citrate synthase assay kit (Sigma-Aldrich, St Louis, MO, USA, CS0720) according to the manufacturer's instructions. The kit uses a colorimetric reaction to measure the rate of reaction between acetyl coenzyme A and oxaloacetic acid. Changes in absorbance were measured using a microplate reader. The activity of cytochrome c oxidase of monocyte lysates (10 μg protein/assay) was measured using the cytochrome c oxidase assay kit (Sigma-Aldrich, St Louis, MO, USA, CYTOCOX1) according to the manufacturer's instructions. The kit uses a colorimetric assay based on observation of the decrease in absorbance of ferrocytochrome c measured at 550 nm, which is caused by its oxidation to ferricytochrome c by cytochrome c oxidase. Changes in absorbance were measured using a microplate reader. The citrate synthase and the cytochrome c oxidase assay were supplied with control enzyme solutions which were used as positive controls (data not shown).
Statistical methods
Multivariate repeated measurements ANOVA (rmANOVA) followed by a least significant difference (LSD) post hoc test was used to compare results at different altitudes and to assess the effect of antioxidant dietary supplements versus placebo. Statistical analysis was performed using a standard statistic software package (PASW Statistics 18, version 18.0.0).
Results
In the examined climbers (seven males, five females, age between 25 and 58), ascent to high altitudes led to significant hypoxia as measured by pulse oximetry. Two of the climbers aborted the climb after reaching 4590 m due to fatigue and did not reach the highest camp. Mean oxygen saturation decreased to 91 ± 3% at 3550 m, 86 ± 3% at 4590 m and 81 ± 5% at 5530 m (P < 0.0001) and did not differ between the antioxidants and the placebo group (). All participants in both groups received either antioxidants or placebo as intended and were analyzed for the primary outcome. No side effects of antioxidant treatment or placebo were observed. Citrate synthase activity increased with altitude (P = 0.003) and was significantly higher at all altitudes compared to baseline levels at 490 m (). Prolonged hypobaric hypoxia induced an overall increase in cytochrome c oxidase enzymatic activity (P = 0.003), mainly due to the increase at 4590 m (). Citrate synthase is an enzyme present in the mitochondrial matrix which remains highly constant in the mitochondria and it is commonly used to determine mitochondrial density.Citation19–Citation21 Citrate synthase activity was used to normalize the enzymatic activity of cytochrome c oxidase. Normalization of cytochrome c oxidase enzymatic activity by citrate synthase activity (relative enzymatic activity) yielded an overall decrease in relative cytochrome c oxidase enzymatic activity during the stay at high altitudes (P = 0.012). When compared to baseline levels, the relative enzymatic activities were significantly lower at 3550 and 5530 m (). Neither citrate synthase activity nor cytochrome c oxidase enzymatic activity differed significantly between the subject group receiving antioxidant dietary supplements and the placebo group.
Figure 1. Oxygen saturation of subjects on different altitudes. Oxygen saturation of subjects at different altitudes. Statistics: P: RMA (repeated measurements ANOVA), followed by LSD post hoc test (*P < 0.05 compared with lowest altitude, 490 m).
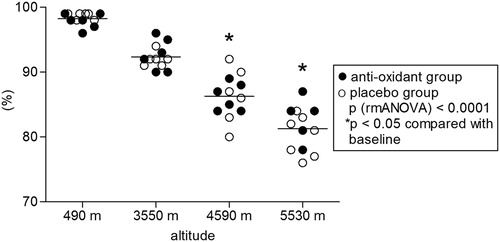
Figure 2. Citrate synthase activity. Effect of hypobaric hypoxia on citrate synthase activity of PBM, isolated from 12 climbers throughout the high-altitude expedition. The enzymatic activity of cell extract was assessed using the citrate synthase assay kit (Sigma, Switzerland) and changes in absorbance were measured using a microplate reader. Activity is expressed in mUnits (nmole/ml/min)/mg of whole cellular homogenate protein. P: RMA (repeated measurements ANOVA), followed by LSD post hoc test (*P < 0.05 compared with lowest altitude, 490 m).
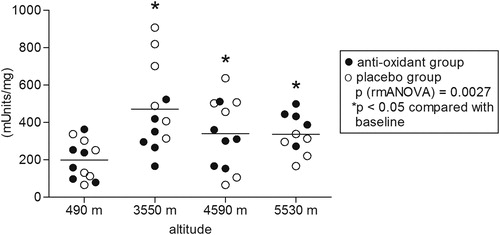
Figure 3. Cytochrome c oxidase activity. Effect of hypobaric hypoxia on cytochrome c oxidase activity of PBM, isolated from 12 climbers throughout the high-altitude expedition. The enzymatic activity in whole cell extract was assessed using the cytochrome c oxidase activity assay kit (Sigma, Switzerland) and changes in absorbance were measured using a microplate reader. Activity is expressed in units (μmole/ml/min)/mg of whole cellular homogenate protein. Statistics: P: RMA (repeated measurements ANOVA), followed by LSD post hoc test (*P < 0.05 compared with lowest altitude, 490 m).
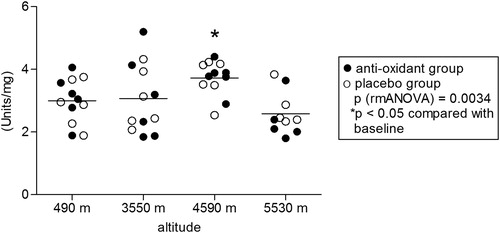
Figure 4. Cytochrome c oxidase activity/citrate synthase activity ratio. Normalization of cytochrome c oxidase enzymatic activity by citrate synthase activity (relative enzymatic activity) yields a decrease in cytochrome c oxidase enzymatic activity during hypobaric hypoxia. Statistics: P: RMA (repeated measurements ANOVA), followed by least significant difference (LSD) post hoc test (*P < 0.05 compared with lowest altitude, 490 m).
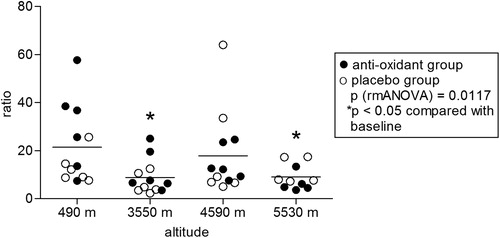
Discussion
The main finding of this study is that hypobaric hypoxia at high altitudes leads to an increase in citrate synthase activity of monocyte mitochondria, whereas cytochrome c oxidase enzymatic activity remained stable in two of three altitude measurements. This finding indicates a decrease in relative cytochrome c oxidase enzymatic activity at 3550 and 5530 m, suggesting that an increase in mitochondrial mass may represent a compensatory mechanism to preserve oxidative phosphorylation of monocytes at high altitude. We did not detect any effect antioxidant dietary supplements did not have on the observed changes in mitochondrial enzymatic activities.
Data on altitude-induced effects on peripheral blood cell mitochondria are scarce. However, the use of blood cells for evaluation of mitochondrial function at high altitudes represents a novel and attractive approach. Blood samples can be obtained from volunteers and processed relatively easily even in challenging field conditions, whereas sampling of other tissues is hindered by obvious ethical and technical constraints. Mariggiò et al.Citation22 reported a decrease in mitochondrial membrane potential in peripheral blood lymphocytes in healthy volunteers several days after completion of a 21-day stay at maximum altitude of 6400 m. The authors concluded that the observed decrease was linked to higher oxidative stress in the context of hypoxic conditions. We are not aware of studies evaluating mitochondrial function in human blood cells during the course of exposure to hypobaric hypoxia. Contrary to reported findings in skeletal muscle cells showing a decrease in mitochondrial volume density, our data in PBM showed an increase in citrate synthase activity as a marker enzyme for the mitochondrial matrix representing mass and/or number of mitochondria. Simultaneously, we found an increase in cytochrome c oxidase activity as a marker enzyme of oxidative phosphorylation only at the medium altitude of 4590 m, whereas activity at 5530 m had returned to baseline values. These findings indicate that hypoxic conditions led to a decrease in relative cytochrome c oxidase activity, suggesting a decrease in relative mitochondrial oxidative phosphorylation capacity, which was counterbalanced by a corresponding increase in mitochondrial mass.
Thus far, research on mitochondrial function at high altitudes has concentrated on skeletal muscle cells. Early records of change in muscle oxidative capacity under hypoxic conditions were reported by Reynafarje.Citation23 The author found higher cytochrome c oxidase activity in muscle biopsies of high-altitude residents compared to low-landers. This paper and similar findings led to the assumption that long-term local tissue hypoxia stimulates muscle oxidative capacity in order to compensate for lack of oxygen.Citation24 However, studies in muscle samples obtained from high-altitude climbers before and after expeditions did not support this hypothesis. Examining subjects before and after high-altitude exposure, different authors found decreases in enzyme activities of aerobic-oxidative metabolism which were related to decrements in mitochondrial volume density, suggesting a cellular loss of mitochondrial structure rather than qualitative changes of the mitochondrial function.Citation25–Citation29 In these subjects, significant reduction of muscle-fiber cross-sectional area, and an unchanged capillary-to-muscle-fiber ratio and therefore increased capillary density, have been reported. The effect of hypoxic exposure on citrate synthase activity which is a marker of mitochondrial volume density and oxidative capacity remains controversial. Citation30–Citation33 Factors such as exposure time to hypoxia, the time of tissue sampling and type of analyzed tissue or cell might contribute to the observed differences. In one study in Sprague-Dawley rats exposed to acute (24 hours) or chronic hypobaric hypoxic (5 weeks) conditions, cardiac, hepatic and skeletal muscle citrate synthase enzymatic activity remained unchanged, suggesting that mitochondrial content was unchanged.Citation30 In another study in male subjects, leg muscle tissue samples were taken at sea level and after 75 days altitude exposure at 5250 m or higher. The authors reported that altitude exposure did not affect the leg muscle citrate synthase activity, reflecting unaltered mitochondrial capacity.Citation31 In another study, pikas from high altitude had higher citrate synthase activity in heart and diaphragm when compared with pikas from low altitude. However, skeletal muscle citrate synthase activity levels did not differ.Citation32
The observed decrease in relative mitochondrial oxidative phosphorylation capacity in our climbers resembles the changes reported in immune cells of septic patients.Citation14,Citation34 In our subjects, oxidative phosphorylation capacity was preserved by compensatory mechanisms, similar to a compensated state seen in the context of an inflammatory response.Citation35,Citation36 Contrasting this regulatory process in immune cells at high altitudes and in sepsis is the loss of mitochondrial structure – rather than functional change of the mitochondrial activity – in muscle cells at high altitudes.Citation25–Citation29
In our study, antioxidant dietary supplements did not have any effect on the observed mitochondrial abnormalities. In human subjects at high altitude, results of trials on antioxidative treatment have shown mixed results. An antioxidant supplement mixture containing beta-carotene, vitamin E, vitamin C, selenium, and zinc did not reduce markers of oxidative stress.Citation37 In another study, oral antioxidant supplementation with preparations containing vitamins C and E in humans at high altitude was shown to decrease breath pentanes.Citation8 One study reported a significant reduction in symptoms of acute mountain sickness in subjects taking an oral antioxidant cocktail38 – a finding that was not confirmed by subsequent similar research.Citation39 The conflicting results might be explained by the significant heterogeneity of these studies in terms of duration and severity of hypoxic exposure. In an animal model of hypoxia and sepsis, attenuation of the increase of lipid peroxidationCitation40 and reversal of mitochondrial dysfunction by antioxidant treatment have been reported.Citation41,Citation42
The main study limitations are determined by the logistic restrictions of the research facilities at high altitudes. This is reflected by the relatively small sample size of the present study which was determined by technical and logistical restrictions in the context of research at very high altitudes rather than by sample size calculation. Tissue sampling in healthy volunteers is restricted to muscle and blood cells for obvious ethical reasons. It was not possible to measure complex I-, II-, or IV-dependent mitochondrial oxygen consumption, as this would have required on-site high-resolution respirometry. We observed relatively wide inter-individual differences in enzymatic activities. The variable individual reactions to environmental stress factors such as low ambient temperature, UV radiation, physical exercise, or susceptibility to and occurrence of high-altitude sickness might partially explain this finding.
Our results confirm that changes in mitochondrial enzymatic activities of blood cells do occur at high altitude. Our findings suggest that in PBM collected under hypoxic conditions, changes in mitochondrial function parameters occur that are similar to observations in immune cells of septic patients. Future research at high altitudes might elucidate mechanisms leading to changes in mitochondrial function of immune cells in hypoxic conditions not related to low ambient oxygen supply.
Conclusions
In our study, we found that long-term hypoxia leads to specific changes in mitochondrial function of human PBM which are not attenuated by dietary supplementation of antioxidants. Sampling and isolation of PBM is possible in high-altitude research facilities and could provide valuable insight into changes of immune cell mitochondrial function and associated regulatory mechanisms in hypoxic states. Future studies should include larger subject groups and a more detailed evaluation of potentially involved cytokines.
Acknowledgements
The authors thank all the climbers, mountain guides, high-altitude porters and research staff of the SwissExped Pik Lenin high-altitude expedition 2009 for their hard work, endurance, and patience under challenging conditions. Thanks go to Jeannie Wurz for editorial assistance.
Disclaimer statements
Contributors All authors contributed equally.
Funding This study was supported by an unconditional research grant from the Department of Intensive Care Medicine, Bern University Hospital and University of Bern, Switzerland.
Conflicts of interest The authors declare they have no conflict of interest.
Ethics approval The study protocol was approved by the ethical committee of the Canton of Aarau, Switzerland and adheres to the Declaration of Helsinki. Subjects were included in the study after their written informed consent was obtained.
References
- Schoene RB. Illnesses at high altitude. Chest 2008;134(2):402–16.
- Mazzeo RS, Reeves JT. Adrenergic contribution during acclimatization to high altitude: perspectives from Pikes Peak. Exerc Sport Sci Rev 2003;31(1):13–8.
- Mazzeo RS, Child A, Butterfield GE, Mawson JT, Zamudio S, Moore LG. Catecholamine response during 12 days of high-altitude exposure (4,300 m) in women. J Appl Physiol 1998;84(4):1151–7.
- Mazzeo RS, Donovan D, Fleshner M, Butterfield GE, Zamudio S, Wolfel EE, et al. Interleukin-6 response to exercise and high-altitude exposure: influence of alpha-adrenergic blockade. J Appl Physiol 2001;91(5):2143–9.
- Klokker M, Kharazmi A, Galbo H, Bygbjerg I, Pedersen BK. Influence of in vivo hypobaric hypoxia on function of lymphocytes, neutrocytes, natural killer cells, and cytokines. J Appl Physiol 1993;74(3):1100–6.
- Klokker M, Kjaer M, Secher NH, Hanel B, Worm L, Kappel M, et al. Natural killer cell response to exercise in humans: effect of hypoxia and epidural anesthesia. J Appl Physiol 1995;78(2):709–16.
- Dosek A, Ohno H, Acs Z, Taylor AW, Radak Z. High altitude and oxidative stress. Respir Physiol Neurobiol 2007;158(2–3):128–31.
- Chao WH, Askew EW, Roberts DE, Wood SM, Perkins JB. Oxidative stress in humans during work at moderate altitude. J Nutr 1999;129(11):2009–12.
- Schmidt MC, Askew EW, Roberts DE, Prior RL, Ensign WY, Jr, Hesslink RE, Jr Oxidative stress in humans training in a cold, moderate altitude environment and their response to a phytochemical antioxidant supplement. Wilder Environ Med 2002;13(2):94–105.
- Martin D, Windsor J. From mountain to bedside: understanding the clinical relevance of human acclimatisation to high-altitude hypoxia. Postgrad Med J 2008;84(998):622–7; quiz 26.
- Grocott M, Montgomery H, Vercueil A. High-altitude physiology and pathophysiology: implications and relevance for intensive care medicine. Critical Care (London, England) 2007;11(1):203.
- Docke WD, Randow F, Syrbe U, Krausch D, Asadullah K, Reinke P, et al. Monocyte deactivation in septic patients: restoration by IFN-gamma treatment. Nat Med 1997;3(6):678–81.
- Munoz C, Carlet J, Fitting C, Misset B, Bleriot JP, Cavaillon JM. Dysregulation of in vitro cytokine production by monocytes during sepsis. J Clin Invest 1991;88(5):1747–54.
- Belikova I, Lukaszewicz AC, Faivre V, Damoisel C, Singer M, Payen D. Oxygen consumption of human peripheral blood mononuclear cells in severe human sepsis. Crit Care Med 2007;35(12):2702–8.
- Fink MP. Cytopathic hypoxia. Mitochondrial dysfunction as mechanism contributing to organ dysfunction in sepsis. Crit Care Clin 2001;17(1):219–37.
- Crouser ED, Julian MW, Blaho DV, Pfeiffer DR Endotoxin-induced mitochondrial damage correlates with impaired respiratory activity. Crit Care Med 2002;30(2):276–84.
- Japiassu AM, Santiago AP, d'Avila JC, Garcia-Souza LF, Galina A, Castro Faria-Neto HC, et al. Bioenergetic failure of human peripheral blood monocytes in patients with septic shock is mediated by reduced F1Fo adenosine-5'-triphosphate synthase activity. Crit Care Med 2011;39(5):1056–63.
- Joanny P, Steinberg J, Robach P, Richalet JP, Gortan C, Gardette B, et al. Operation Everest III (Comex'97): the effect of simulated sever hypobaric hypoxia on lipid peroxidation and antioxidant defence systems in human blood at rest and after maximal exercise. Resuscitation 2001;49(3):307–14.
- Barrientos A. In vivo and in organello assessment of OXPHOS activities. Methods 2002;26(4):307–16.
- Rustin P, Chretien D, Bourgeron T, Gerard B, Rotig A, Saudubray JM, et al. Biochemical and molecular investigations in respiratory chain deficiencies. Clin Chim Acta 1994;228(1):35–51.
- Hood DA, Zak R, Pette D. Chronic stimulation of rat skeletal muscle induces coordinate increases in mitochondrial and nuclear mRNAs of cytochrome-c-oxidase subunits. Eur J Biochem 1989;179(2):275–80.
- Mariggio MA, Falone S, Morabito C, Guarnieri S, Mirabilio A, Pilla R, et al. Peripheral blood lymphocytes: a model for monitoring physiological adaptation to high altitude. High Altit Med Biol 2010;11(4):333–42.
- Reynafarje B. Myoglobin content and enzymatic activity of muscle and altitude adaptation. J Appl Physiol 1962;17:301–5.
- Hochachka PW, Stanley C, Merkt J, Sumar-Kalinowski J. Metabolic meaning of elevated levels of oxidative enzymes in high altitude adapted animals: an interpretive hypothesis. Respir Physiol 1983;52(3):303–13.
- Howald H, Pette D, Simoneau JA, Uber A, Hoppeler H, Cerretelli P. Effect of chronic hypoxia on muscle enzyme activities. Int J Sport Med 1990;11(Suppl. 1):S10–4.
- MacDougall JD, Green HJ, Sutton JR, Coates G, Cymerman A, Young P, et al. Operation Everest II: structural adaptations in skeletal muscle in response to extreme simulated altitude. Acta Physiol Scand 1991;142(3):421–7.
- Bakkman L, Sahlin K, Holmberg HC, Tonkonogi M. Quantitative and qualitative adaptation of human skeletal muscle mitochondria to hypoxic compared with normoxic training at the same relative work rate. Acta Physiol 2007;190(3):243–51.
- Ferretti G. Limiting factors to oxygen transport on Mount Everest 30 years after: a critique of Paolo Cerretelli's contribution to the study of altitude physiology. Eur J Appl Physiol 2003;90(3–4):344–50.
- Howald H, Hoppeler H. Performing at extreme altitude: muscle cellular and subcellular adaptations. Eur J Appl Physiol 2003;90(3–4):360–4.
- Kennedy SL, Stanley WC, Panchal AR, Mazzeo RS. Alterations in enzymes involved in fat metabolism after acute and chronic altitude exposure. J Appl Physiol 2001;90(1):17–22.
- Mizuno M, Savard GK, Areskog NH, Lundby C, Saltin B. Skeletal muscle adaptations to prolonged exposure to extreme altitude: a role of physical activity? High Altit Med Biol 2008;9(4):311–7.
- Sheafor BA. Metabolic enzyme activities across an altitudinal gradient: an examination of pikas (genus Ochotona). J Exper Biol 2003;206(Pt 7):1241–9.
- Faoro V, Fink B, Taudorf S, Dehnert C, Berger MM, Swenson ER, et al. Acute in vitro hypoxia and high-altitude (4,559 m) exposure decreases leukocyte oxygen consumption. Am J Physiol Regul Integ Comp Physiol 2011;300(1):R32–9.
- Adrie C, Bachelet M, Vayssier-Taussat M, Russo-Marie F, Bouchaert I, Adib-Conquy M, et al. Mitochondrial membrane potential and apoptosis peripheral blood monocytes in severe human sepsis. Am. J Respir Crit Care Med 2001;164(3):389–95.
- Marshall JC, Charbonney E, Gonzalez PD. The immune system in critical illness. Clin Chest Med 2008;29(4):605–16, vii.
- Hotchkiss RS, Karl IE. The pathophysiology and treatment of sepsis. N Eng J Med 2003;348(2):138–50.
- Pfeiffer JM, Askew EW, Roberts DE, Wood SM, Benson JE, Johnson SC, et al. Effect of antioxidant supplementation on urine and blood markers of oxidative stress during extended moderate-altitude training. Wilder Environ Med 1999;10(2):66–74.
- Bailey DM, Davies B. Acute mountain sickness; prophylactic benefits of antioxidant vitamin supplementation at high altitude. High Altit Med Biol 2001;2(1):21–9.
- Baillie JK, Thompson AA, Irving JB, Bates MG, Sutherland AI, Macnee W, et al. Oral antioxidant supplementation does not prevent acute mountain sickness: double blind, randomized placebo-controlled trial. QJM 2009;102(5):341–8.
- Ilavazhagan G, Bansal A, Prasad D, Thomas P, Sharma SK, Kain AK, et al. Effect of vitamin E supplementation on hypoxia-induced oxidative damage in male albino rats. Aviat Space Environ Med 2001;72(10):899–903.
- Zapelini PH, Rezin GT, Cardoso MR, Ritter C, Klamt F, Moreira JC, et al. Antioxidant treatment reverses mitochondrial dysfunction in a sepsis animal model. Mitochondrion 2008;8(3):211–8.
- Ritter C, Andrades ME, Reinke A, Menna-Barreto S, Moreira JC, Dal-Pizzol F. Treatment with N-acetylcysteine plus deferoxamine protects rats against oxidative stress and improves survival in sepsis. Crit Care Med 2004;32(2):342–9.