Abstract
Objectives: The subject of this study was 3-mercapto-5H-1,2,4-triazino[5,6-b]indole-5-acetic acid (compound 1), an efficient aldose reductase inhibitor of high selectivity. The antioxidant action of 1 was investigated in greater detail by employing a 1,1′-diphenyl-2-picrylhydrazyl (DPPH) test and in the system of isolated rat erythrocytes.
Methods: First, the compound was subjected to the DPPH test. Second, the overall antioxidant action of the compound was studied in the cellular system of isolated rat erythrocytes oxidatively stressed by free radicals derived from the lipophilic tert-butyl hydroperoxide. The uptake kinetics of 1 was studied and osmotic fragility of the erythrocytes was evaluated.
Results: The DPPH test revealed significant antiradical activity of 1. One molecule of 1 was found to quench 1.48 ± 0.06 DPPH radicals. In the system of isolated erythrocytes, the compound was readily taken up by the cells followed by their protection against free radical-initiated hemolysis. Osmotic fragility of the erythrocytes was not affected by 1.
Conclusions: The results demonstrated the ability of 1 to scavenge DPPH and to protect intact erythrocytes against oxidative damage induced by peroxyl radicals. By affecting both the polyol pathway and oxidative stress, the compound represents an example of a promising agent for multi-target pharmacology of diabetic complications.
Introduction
Under hyperglycemic conditions, aldose reductase reduces some of the excess glucose to the organic osmolyte sorbitol in an NADPH-dependent manner. The accumulation of sorbitol increases cellular osmolarity, leading to a deleterious hyperosmotic swellingCitation1–Citation4 which may contribute to the development of diabetic complications such as cataract, retinopathy, neuropathy, and nephropathy. Improper control of glycemia is connected with activation of both oxidative and endoplasmic reticulum stress signaling pathways.Citation5–Citation9 Elevated blood and tissue levels of markers of oxidative stress were documented under diabetic conditions.Citation10–Citation20 In animal studies, antioxidant supplementation was found to attenuate hyperglycemia-related complications.Citation21–Citation28
The above data provide support for the use of aldose reductase inhibitors and antioxidants to minimize consequences of glucose flux through the polyol pathway and hyperglycemia-induced oxidations.Citation29–Citation34 Bifunctional drugs with joint antioxidant/aldose reductase inhibitory activities would be multifactorially beneficial in the treatment of diabetic complications. Compounds such as pyridazines,Citation35 benzopyranes,Citation36 pyridopyrimidines,Citation37 and carboxymethylated pyridoindolesCitation38 have been shown to display antioxidant as well as aldose reductase inhibitory activities under in vitro conditions.
Recently, novel aldose reductase inhibitors based on carboxymethylated mercapto-triazino-indole scaffold have been designed.Citation39 Among the novel compounds, 3-mercapto-5H-1,2,4-triazino[5,6-b]indole-5-acetic acid (compound 1, Fig. ) was the most promising inhibitor, with an IC50 in submicromolar range and high selectivity. Physicochemical parameters matching ‘the rule of five’, along with good water solubility, point to an excellent ‘drug-likeness’ of 1. Currently, compound 1 has been the subject of complex preclinical studies as a prospective agent with a therapeutic potential in the treatment of diabetic complications.
In the present work, we evaluated the efficiency of compound 1 in scavenging stable free radicals of 1,1′-diphenyl-2-picrylhydrazyl (DPPH) in ethanolic solution. Further, we studied the antioxidant action of the compound in the cellular system of intact erythrocytes exposed to tert-butyl hydroperoxide (t-BuOOH) in vitro. The activities of 1 were compared with the activity of the structural congener 2 (Fig. ) with alkylated –SH group and melatonin as a reference antioxidant. Since the critical property for the efficacy of a novel drug in vivo is its ability to penetrate into target tissues, we studied the uptake of compound 1 into isolated red blood cells.
Materials and methods
Chemicals and instruments
Compound 1 was supplied by Matrix Scientific, Columbia, SC, USA. Compound 2, 3-(propan-2-ylsulfanyl)-5H-[Citation1,Citation2,Citation4]triazino[5,6-b]indole-5-acetic acid, was from Ambinter, c/o Greenpharma, Orléans, France. A purity of >99% has been established by LC–MS technique for both compounds.Citation39 Melatonin (≥98%) and DPPH were obtained from Sigma Chemical Co. (St Louis, MO, USA). t-BuOOH was purchased from Sigma-Aldrich Chemie GmbH (Steinheim, Germany). Other chemicals were purchased from local commercial sources and were of analytical grade quality. Spectrophotometric analysis was performed using a Hewlett-Packard Diode Array Spectrophotometer 8452A.
Animals
Male Wistar rats, 8–9 weeks old, weighing 200–250 g, were used. The animals came from the Breeding Facility of the Institute of Experimental Pharmacology (Dobra Voda, Slovak Republic). The study was approved by the Ethics Committee of the Institute and performed in accordance with the Principles of Laboratory Animal Care (NIH publication 83-25, revised 1985) and the Slovak law regulating animal experiments (Decree 289, Part 139, 9 July 2003).
DPPH test
Ethanolic solution of DPPH (50 μM) was incubated in the presence of the compound tested at indicated concentrations and at laboratory temperature. The radical reaction was followed by absorbance decrease at λmax = 518 nm.
Preparation of packed erythrocytes
The animals were killed in light ether anesthesia by exsanguination of the carotid artery. Blood was collected in 3.8% sodium citrate (1 vol. of sodium citrate: 9 vol. of blood) and centrifuged at 500 × g for 15 minutes at 4°C. Plasma and white blood cells were removed by aspiration. The retrieved erythrocytes were washed three times with 6 vol. of ice-cold isotonic phosphate-buffered saline (PBS, pH 7.4, 1.9 mM NaH2PO4, 8.1 mM Na2HPO4, and 150 mM NaCl). The entire procedure was conducted at 0–4°C. After the last washing, the red blood cells were used for further studies. The erythrocyte suspensions used in the experiments were prepared daily.
Hemolysis
The hemolysis studies were performed in rat erythrocyte suspensions in isotonic PBS with a hematocrit of 1.5%. Compound 1 was added from stock solutions in isotonic PBS to the erythrocyte suspensions to the final concentrations as reported. Controls received an equivalent volume of isotonic PBS alone. The samples were then preincubated for 30 minutes at 37°C. t-BuOOH (250 μM final concentration) was added to the samples and incubation continued at 37°C up to 3 hours. Aliquots were withdrawn after different time periods. The incubations were terminated by cooling the suspensions in an ice bath followed by centrifugation at 700 × g for 10 minutes. The degree of hemolysis was assessed by spectrophotometry of the hemoglobin released into the supernatant fraction, as described by Winterbourn.Citation40 The results were calculated as percentage of hemolysis. Total hemolysis (100%) was obtained by incubation of control erythrocytes in 10 mM hypotonic phosphate buffer (pH 7.4, 1.9 mM NaH2PO4, 8.1 mM Na2HPO4) at 37°C for 1 hour. The lag period was determined as an x-axis intersection of the ascending part of the semilogarithmic plot ‘hemolysis (%) vs. log t’.
To analyze the kinetics of t-BuOOH quenching by compound 1, t-BuOOH (250 µM) was incubated in the absence or presence of compound 1 (100 µM or 1 mM) under the conditions of the hemolytic experiment omitting the erythrocytes. t-BuOOH content was determined by modified thiocyanate method of Mihaljevic et al.Citation41 as follows: 1 ml of the incubation mixture was acidified by 1 ml of 10% trichloroacetic acid, followed by addition of 0.4 ml of 10 mM Fe(NH4)2(SO4)2 in 0.2 M HCl, thorough mixing and addition of 0.1 ml of 2.5 M KSCN. After leaving the mixture at ambient temperature for at least 5 minutes, the absorbance at 480 nm was recorded. The content of t-BuOOH was determined from appropriate calibration curves obtained in the absence or presence (100 µM or 1 mM) of compound 1.
Uptake studies
Suspensions of erythrocytes in isotonic PBS were incubated in the presence of 1 in the final concentrations of 100 µM or 1 mM for up to 60 minutes in a shaking water bath at 37°C (hematocrit of 20%). At indicated time intervals, the incubation mixtures were centrifuged at 700 × g for 10 minutes at 4°C to pellet the erythrocytes.
Sodium chloride (0.1 g) was added to the aliquots of the supernatant (0.5 ml) and after addition of ZnSO4 (20%, 0.1 ml), the mixture was kept on ice for 15 minutes for protein precipitation. The mixture was centrifuged at 700 × g for 15 minutes at 4°C. The supernatant (0.5 ml) was diluted with PBS (1.5 ml). The absorbance of the diluted supernatants was measured at 300 nm and the concentrations of 1 were determined by using an appropriate calibration curve.
Distilled water (1 ml) was added to the pellets and incubated at 37°C for 1 hour to hemolyze the erythrocytes. Proteins were precipitated by addition of sodium chloride (0.1 g) and ZnSO4 (20%, 0.1 ml). The mixture was kept on ice for 15 minutes and then centrifuged at 700 × g for 15 minutes at 4°C. The supernatant (0.5 ml) was diluted with PBS (1.5 ml) and the concentration of 1 was determined by spectrophotometry as described above.
The percentage of compound 1 uptake into erythrocytes was then calculated.
Osmotic fragility
The osmotic fragility of the isolated erythrocytes was determined by the degree of hemolysis induced by the changes of osmotic pressure using a step-down protocol with decreasing concentrations of NaCl. The erythrocytes were suspended (hematocrit of 0.4%) in PBS containing increasing concentrations of NaCl from 0–150 mmol/l and 1 in the final concentration of 250 µM. Total hemolysis (100%) was obtained by incubation of control erythrocytes in 10 mmol/l hypotonic phosphate buffer (pH 7.4, 1.9 mmol/l NaH2PO4, 8.1 mmol/l Na2HPO4). The samples were incubated for 1 hour at 37°C in a shaking water bath. After incubation, the erythrocyte suspensions were centrifuged at 700 × g for 15 minutes at 4°C. The hemolysis degree was determined by spectrophotometry of the hemoglobin released into the supernatant, as described by Winterbourn.Citation40
Results
DPPH test
The DPPH assay was used to determine the radical-scavenging potential of the compounds tested. The time-dependent decrease of the characteristic absorbance of the ethanolic solution of DPPH at 518 nm in the presence of 1 and 2 is illustrated in Fig. . As shown in Fig. , compound 1 initiated a substantial absorbance decrease, corresponding to the transfer of the most labile H atoms, which was followed by a much slower absorbance decline representing the residual antiradical activity of the antioxidant degradation products. On the other hand, compound 2 with blocked thiol group affected DPPH absorbance only marginally. The initial velocity of DPPH decolorization determined for the first 30-minute interval for 1 and 2 is shown in Table . For comparison, equimolar melatonin was used as a reference and found to exhibit an about 10 times lower activity.
Figure 2 Continual absorbance decrease of ethanolic solution of DPPH radical (50 μmol/l) in the presence of 200 μM concentration of the compounds tested at λmax = 518 nm. (•) Compound 1 and (○) compound 2. The curves represent results from two typical experiments.
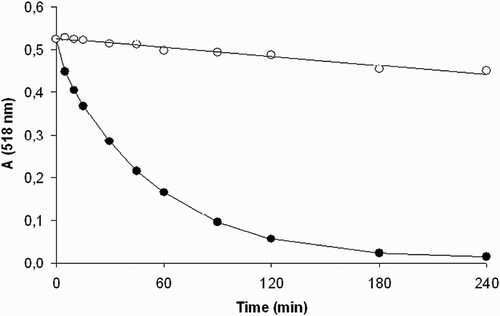
Table 1 DPPH test
Total stoichiometry of DPPH scavenging was determined for 1 (Fig. ). The technique of spectrophotometric titration of fixed concentration of DPPH (50 μM) with increasing concentrations of the antioxidant was used to determine the point of equivalence. In this approach, a sufficiently long reaction time was set (24 hours) to let the reaction run to completion. Figure shows the average absorbance decrease of the ethanolic solution of DPPH radical in the presence of increasing concentrations of 1. By analyzing the individual titration curves, points of equivalence were determined and corresponding stoichiometric factors were calculated. Under the experimental conditions used, one molecule of 1 was found to quench 1.48 ± 0.06 DPPH radicals.
Hemolysis of rat erythrocytes in vitro
As shown in Fig. A, rat erythrocytes exposed to 250 μM t-BuOOH underwent progressive hemolysis, determined by measuring the release of hemoglobin. The onset of hemolysis was shifted from the starting zero point by the time interval assigned as a lag period. In the presence of 1, the lag period increased from 76.86 ± 2.22 minutes for controls to 103.14 ± 5.27 minutes for 100 μM and to >180 minutes for 1 mM concentrations of 1. Melatonin (100 μM) and compound 2 (1 mM) exerted no effects.
Figure 4 (A) Hemolysis curves induced by t-BuOOH. Erythrocyte suspensions (1.5%) were incubated with 250 μM t-BuOOH in the presence of 100 μM (-•-) or 1 mM (-▪-) 1. Control incubations (-Δ-). Each value is the mean ± SD from four to eight experiments. (B) Kinetics of t-BuOOH quenching by compound 1. t-BuOOH (250 µM) was incubated in the absence (-Δ-) or presence of 100 µM (-•-) or 1 mM (-▪-) compound 1 under the conditions of the hemolytic experiment omitting the erythrocytes. Results are mean values ± SD from three experiments.
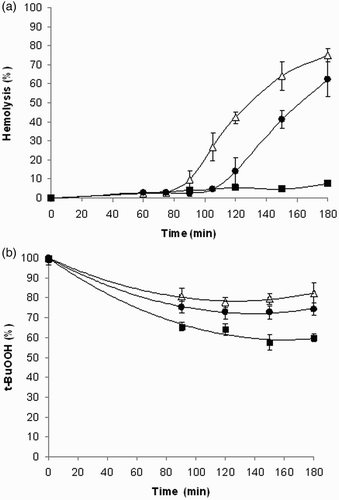
The kinetics of t-BuOOH quenching by compound 1 was analyzed. From the experimental curves obtained (Fig. B), it is apparent that during 3-hour incubation about 20% of t-BuOOH decayed spontaneously. The decrement of t-BuOOH increased by about 5% in the presence of 100 µM compound 1, while at 1 mM concentration of 1, the total drop of t-BuOOH was about 40%. This means that about 75% (60%) of t-BuOOH was still available to interact with the cells at the end of the incubation.
Uptake studies
Figure shows the time course of uptake of 1 by red blood cells in in vitro incubations of the isolated erythrocytes in the presence of 100 μM and 1 mM concentrations of 1.
Osmotic fragility
The effect of 1 on osmotic fragility of the erythrocyte membrane was assessed. As shown in Fig. , compound 1 at the concentration of 250 μM did not affect significantly the course of osmotic hemolysis of erythrocytes.
Figure 6 Effect of compound 1 on osmotic fragility of rat erythrocytes. Erythrocyte suspensions (0.4% hematocrit) in 10 mM phosphate buffer containing increasing concentrations of NaCl were incubated at 37°C for 1 hour in the absence (Δ) or presence (•) of compound 1 (250 μM). Experimental points represent mean values ± SD from at least three independent experiments.
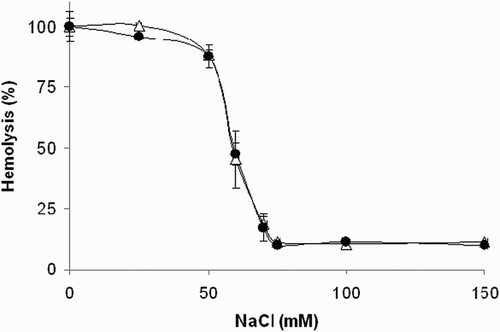
Discussion
Compound 1 belongs to a group of novel carboxymethylated mercapto-triazino-indole derivatives, recently projected as aldose reductase inhibitors.Citation39 The biological activity of 1 was characterized with an IC50 in submicromolar range for aldose reductase inhibition and high selectivity in relation to congeneric aldo-keto reductases. In addition, physicochemical properties of 1 corresponding to excellent ‘drug-likeness’ render the compound a prospective agent with a therapeutic potential in the treatment of diabetic complications. As part of the current preclinical evaluation, the antioxidant activity of compound 1 was demonstrated. Considering an oxidative component in the mechanism of glucose toxicity,Citation13–Citation20 the ability of 1 to scavenge reactive oxygen species may enhance its therapeutic potential in relation to diabetic complications.
DPPH, as a weak hydrogen atom abstractor, is considered a good kinetic model for peroxyl ROO• radicals.Citation42 In the homogeneous system of DPPH in ethanol, the antioxidant activity of a compound tested stems from its intrinsic chemical reactivity towards radicals. An absorbance decrease at 518 nm recorded in the presence of 1 proved its ability to quench DPPH radicals. Based on the kinetics of DPPH decolorization, the antiradical activity of 1 exceeded that of equimolar melatonin. The rather low initial velocity of DPPH decolorization induced by melatonin is in agreement with the findings of Fagali and Catala.Citation43
On placing an isopropyl group on the S atom of 1, thus preventing thione–thiol tautomerism and deprotonation of the –SH group, the antiradical activity was completely lost, indicating that the one-electron-oxidized radical of 1 exists in the thione form rather than in the thiol form. This finding is in agreement with preferred thione tautomeric forms of 1 at neutral pH shown before.Citation39 Analogically, 2-mercaptopyrimidines,Citation44 substituted 1,2,4-triazole-3-thiones,Citation45 and the mercaptoimidazole derivative ergothioneineCitation46 exist in water solutions predominantly in the thione tautomer forms.
In general, the antioxidant efficacy is characterized not only by kinetics of free radical quenching but also by stoichiometry of the scavenging reaction. The total stoichiometry of DPPH scavenging by 1 was determined. Under the experimental conditions used, one molecule of 1 was found to quench 1.48 ± 0.06 DPPH radicals.
In the homogeneous model system of DPPH in ethanol, the antioxidant activity of a compound tested stems from its intrinsic chemical reactivity towards radicals. In membranes, however, the apparent reactivity may be different since it is determined by the distribution ratio of the antioxidant between water and lipid compartments. Considering erythrocyte susceptibility to peroxidation, red blood cells have been used as a model to investigate oxidative damage in biomembranes. Exposure of erythrocytes to free radicals may lead to a number of membrane changes resulting eventually in hemolysis. Lipid peroxidation and protein oxidation are likely to play a key role in the hemolytic process. In this study, oxidative damage of plasma membrane was induced by t-BuOOH. Owing to its high lipophilicity, t-BuOOH is rapidly taken up by red blood cells, which is followed by generating peroxyl radicals.Citation47,Citation48 As shown in Fig. A, rat erythrocytes exposed to 250 μM t-BuOOH underwent progressive hemolysis followed by the release of hemoglobin. The onset of t-BuOOH-induced hemolysis was shifted from the starting zero point by the time interval designated as lag period. In the presence of 1, the lag period increased significantly in a concentration-dependent manner. At 1 mM concentration of 1, no hemolysis occurred during the 3-hour incubation period. Based on lag phase prolongation, it can be deduced that the erythrocytes were protected by 1 against t-BuOOH-induced hemolysis.
As shown in Fig. B, direct scavenging of t-BuOOH by compound 1 is only partially responsible for protection of erythrocytes against t-BuOOH-induced hemolysis. This finding, in addition to the ability of t-BuOOH to diffuse freely into red blood cell cytosol, as reported by Trotta et al.,Citation47 suggests that compound 1 protects erythrocytes against oxidative damage induced by peroxyl radicals generated intracellularly.
The above indication is supported by the results shown in Fig. giving evidence of a ready uptake of compound 1 by red blood cells. At 100 μM concentration of 1, saturation of the uptake was achieved as early as in the 30th minute of incubation. Considering the 20% hematocrit used in the experiment, the ‘saturated’ value of the uptake reached at about 20% is indicative of the passive transport through the plasmatic membrane. At 1 mM concentration of 1, the process of uptake was even faster and the maximal uptake was achieved approximately at the 15th minute. The maximal value of the uptake above 20% points to a potential contribution of an active component in the uptake mechanism.
Since thiols may be specifically toxic to erythrocytes,Citation49 we studied the effect of 1 on hemolysis of isolated red blood cells induced by an osmotic gradient. As demonstrated in a separate experiment, compound 1 at the concentration of 250 μM did not affect significantly the osmotic fragility of the erythrocyte plasmatic membrane.
To conclude, our results demonstrated in a DPPH test the antiradical efficacy of a novel carboxymethylated mercapto-triazino-indole inhibitor of aldose reductase and its ability to protect intact erythrocytes against oxidative damage induced by peroxyl radicals. By affecting both the polyol pathway and oxidative stress, compound 1 represents an example of a promising agent for multi-target pharmacology of diabetic complications.
Disclaimer statements
Contributors: None
Funding: None
Conflicts of interest: Authors declare no conflicts of interest.
Ethics approval: The study was approved by the Ethics Committee of the Institute and performed in accordance with the Principles of Laboratory Animal Care (NIH publication 83-25, revised 1985) and the Slovak law regulating animal experiments (Decree 289, Part 139, 9 July 2003).
Acknowledgments
This study was supported by the grant VEGA 2/0041/15 and VEGA 2/0033/14 as well as by MVTS COST CM1103 and BM1204.
References
- Hotta N. New approaches for treatment in diabetes: aldose reductase inhibitors. Biomed Pharmacother 1995;5:244–50.
- Yabe-Nishimura C. Aldose reductase in glucose toxicity: a potential target for the prevention of diabetic complications. Pharmacol Rev 1998;50:21–33.
- Del Corso A, Cappiello M, Mura U. From a dull enzyme to something else: facts and perspectives regarding aldose reductase. Curr Med Chem 2008;15:1452–61. doi: 10.2174/092986708784638870
- Oates PJ. Aldose reductase, still a compelling factor for diabetic neuropathy. Curr Drug Targets 2008;9:14–36. doi: 10.2174/138945008783431781
- Palsamy P, Bidasee KR, Ayaki M, Augusteyn RC, Chan JY, Shinohara T. Methylglyoxal induces endoplasmic reticulum stress and DNA demethylation in the Keap 1 promoter of human lens epithelial cells and age-related cataracts. Free Radic Biol Med 2014;72:134–48. doi: 10.1016/j.freeradbiomed.2014.04.010
- Liu H, Cao MM, Wang Y, Li LC, Zhu LB, Xie GY, et al.Endoplasmic reticulum stress is involved in the connection between inflammation and autophagy in type 2 diabetes. Gen Comp Endocrinol 2015;210:124–9. doi: 10.1016/j.ygcen.2014.09.006
- Chistiakov DA, Sobenin IA, Orekhov AN, Bobryshev YV. Role of endoplasmic reticulum stress in atherosclerosis and diabetic macrovascular complications. Biomed Res Int 2014;2014:610140.
- O'Brien PD, Hinder LM, Sakowski SA, Feldman EL. ER Stress in diabetic peripheral neuropathy: a new therapeutic target. Antioxid Redox Signal 2014;21(4):621–33. doi: 10.1089/ars.2013.5807
- Fiorentino TV, Prioletta A, Zuo P, Folli F. Hyperglycemia-induced oxidative stress and its role in diabetes mellitus related cardiovascular diseases. Curr Pharm Des 2013;19(32):5695–703. doi: 10.2174/1381612811319320005
- Bhuyan KC, Bhuyan DK. Molecular mechanism of cataractogenesis: III. Toxic metabolites of oxygen as initiators of lipid peroxidation and cataract. Curr Eye Res 1984;3:67–81. doi: 10.3109/02713688408997188
- Altomare E, Vendemiale G, Grattagliano I, Angelini P, Micelli-Ferrari T, Cardia L. Human diabetic cataract: role of lipid peroxidation. Diabetes Metab 1995;21:173–9.
- Altomare E, Grattagliano I, Vendemaile G, Micelli-Ferrari T, Signorile A, Cardia L. Oxidative protein damage in human diabetic eye: evidence of a retinal participation. Eur J Clin Invest 1997;27:141–7. doi: 10.1046/j.1365-2362.1997.780629.x
- Baynes JW, Thorpe SR. Perspectives in diabetes: role of oxidative stress in diabetic complication – a new perspective on an old paradigm. Diabetes 1999;48:1–9. doi: 10.2337/diabetes.48.1.1
- Kubo E, Miyoshi N, Fukuda M, Akagi Y. Cataract formation through the polyol pathway is associated with free radical production. Exp Eye Res 1999;68:457–64. doi: 10.1006/exer.1998.0624
- Kyselova Z, Stefek M, Bauer V. Pharmacological prevention of diabetic cataract. J Diabetes Complications 2004;18(2):129–40. doi: 10.1016/S1056-8727(03)00009-6
- Brownlee M. The pathobiology of diabetic complications: a unifying mechanism. Diabetes 2005;54:1615–25. doi: 10.2337/diabetes.54.6.1615
- Kyselova Z, Garcia SJ, Gajdosikova A, Gajdosik A, Stefek M. Temporal relationship between lens protein oxidation and cataract development in streptozotocin-induced diabetic rats. Physiol Res 2005;54(1):49–56.
- Stefek M, Gajdosikova A, Gajdosik A, Kyselova Z, Djoubissie P-O, Krizanova L. Glyco-oxidative mechanisms in glucose toxicity: biochemical changes of matrix collagen in diabetic rats. Biologia 2005;60(Suppl. 17):109–12.
- Obrosova IG. Increased sorbitol pathway activity generates oxidative stress in tissue sites for diabetic complications. Antioxid Redox Signal 2005;7:1543–52. doi: 10.1089/ars.2005.7.1543
- Monnier VM, Mustata GT, Biemel KL, Reihl O, Lederer MO, Zhenyu D, et al.Cross-linking of the extracellular matrix by the Maillard reaction in aging and diabetes: an update on ‘a puzzle nearing resolution’. Ann N Y Acad Sci 2005;1043:533–44. doi: 10.1196/annals.1333.061
- Ohta Y, Yamasaki T, Niwa T, Majima Y, Ishiguro I. Preventive effect of topical vitamin E-containing liposome instillation on the progression of galactose cataract. Comparison between 5-week- and 12-week-old rats fed a 25% galactose diet. Exp Eye Res 1999;68:747–55. doi: 10.1006/exer.1998.0672
- Agardh E, Hultberg B, Agardh C. Effects of inhibition of glycation and oxidative stress on the development of cataract and retinal vessel abnormalities in diabetic rats. Curr Eye Res 2000;21:543–9. doi: 10.1076/0271-3683(200007)2111-ZFT543
- Packer L, Kraemer K, Rimbach G. Molecular aspects of lipoic acid in the prevention of diabetes complications. Nutrition 2001;17:888–95. doi: 10.1016/S0899-9007(01)00658-X
- Stefek M, Gajdosik A, Tribulova N, Navarova J, Volkovova K, Weismann P, et al.The pyridoindole antioxidant stobadine attenuates albuminuria, enzymuria, kidney lipid peroxidation and matrix collagen cross-linking in streptozotocin-induced diabetic rats. Methods Find Exp Clin Pharmacol 2002;24(9):565–71.
- Ulusu NN, Sahilli M, Avci A, Canbolat O, Ozansoy G, Ari N, et al.Pentose phosphate pathway, glutathione-dependent enzymes and antioxidant defense during oxidative stress in diabetic rodent brain and peripheral organs: effect of stobadine and vitamin E. Neurochem Res 2003;28(6):815–23. doi: 10.1023/A:1023202805255
- Kyselova Z, Gajdosik A, Gajdosikova A, Ulicna O, Mihalova D, Karasu C, et al.Effect of the pyridoindole antioxidant stobadine on development of experimental diabetic cataract and on lens protein oxidation in rats: comparison with vitamin E and BHT. Mol Vis 2005;11:56–65.
- Juranek I, Horakova L, Rackova L, Stefek M. Antioxidants in treating pathologies involving oxidative damage: an update on medicinal chemistry and biological activity of stobadine and related pyridoindoles. Curr Med Chem 2010;17(6):552–70. doi: 10.2174/092986710790416317
- Aldini G, Vistoli G, Stefek M, Chondrogianni N, Grune T, Sereikaite J, et al.Molecular strategies to prevent, inhibit, and degrade advanced glycoxidation and advanced lipoxidation end products. Free Radic Res 2013;47(Suppl. 1 SI):93–137. doi: 10.3109/10715762.2013.792926
- Costantino L, Rastelli G, Gamberini MC, Barlocco D. Pharmacological approaches to the treatment of diabetic complications. Expert Opin Ther Pat 2000;10:1245–62. doi: 10.1517/13543776.10.8.1245
- Miyamoto S. Recent advances in aldose reductase inhibitors: potential agents for the treatment of diabetic complications. Expert Opin Ther Pat 2002;12:621–31. doi: 10.1517/13543776.12.5.621
- Alexiou P, Pegklidou K, Chatzopoulou M, Nicolaou I, Demopoulos VJ. Aldose reductase enzyme and its implication to major health problems of the 21(st) century. Curr Med Chem 2009;16:734–52. doi: 10.2174/092986709787458362
- Srivastava SK, Ramana KV, Bhatnagar A. Role of aldose reductase and oxidative damage in diabetes and the consequent potential for therapeutic options. Endocr Rev 2005;26:380–92. doi: 10.1210/er.2004-0028
- Chatzopoulou M, Alexiou P, Kotsampasakou E, Demopoulos VJ. Novel aldose reductase inhibitors: a patent survey (2006–present). Expert Opin Ther Pat 2012;11:1303–23. doi: 10.1517/13543776.2012.726615
- Maccari R, Ottana R. Targeting aldose reductase for the treatment of diabetes complications and inflammatory diseases: new insights and future directions. J Med Chem 2015;58(5):2047–67. doi: 10.1021/jm500907a
- Coudert P, Albuisson E, Boire JY, Duroux E, Bastide P, Couquelet J. Synthesis of pyridazine acetic acid derivatives possessing aldose reductase inhibitory activity and antioxidant properties. Eur J Med Chem 1994;29:471–7. doi: 10.1016/0223-5234(94)90074-4
- Costantino L, Rastelli G, Gamberini MC, Vinson JA, Bose P, Iannone A, et al.1-Benzopyran-4-one antioxidants and aldose reductase inhibitors. J Med Chem 1999;42:1881–93. doi: 10.1021/jm980441h
- La Motta C, Sartini S, Mugnaini L, Simorini F, Taliani S, Salerno S, et al.Pyrido[1,2-a] pyrimidin-4-one derivatives as a novel class of selective aldose reductase inhibitors exhibiting antioxidant activity. J Med Chem 2007;50:4917–27. doi: 10.1021/jm070398a
- Stefek M, Snirc V, Djoubissie P-O, Majekova M, Demopoulos V, Rackova L, et al.Carboxymethylated pyridoindole antioxidants as aldose reductase inhibitors: synthesis, activity, partitioning, and molecular modelling. Bioorg Med Chem 2008;16:4908–20. doi: 10.1016/j.bmc.2008.03.039
- Stefek M, Soltesova Prnova M, Majekova M, Rechlin C, Heine A, Klebe G. Identification of novel aldose reductase inhibitors based on carboxymethylated mercapto-triazino-indole scaffold. J Med Chem 2015;58(6):2649–57. doi: 10.1021/jm5015814
- Winterbourn CC. Oxidative reactions of hemoglobin. Methods Enzymol 1990;186:265–72. doi: 10.1016/0076-6879(90)86118-F
- Mihaljevic B, Katusin-Razem B, Razem B. The reevaluation of the ferric thiocyanate assay for lipid hydroperoxides with special considerations of the mechanistic aspects of the response. Free Radic Biol Med 1996;21(1):53–63. doi: 10.1016/0891-5849(95)02224-4
- Blois MS. Antioxidant determinations by the use of a stable free radical. Nature 1958;181:1199–200. doi: 10.1038/1811199a0
- Fagali N, Catala A. The effect of melatonin and structural analogues on the lipid peroxidation of triglycerides enriched in ω-3 polyunsaturated fatty acids. Life Sci 2007;81:299–305. doi: 10.1016/j.lfs.2007.05.013
- Stoyanov S, Petkov I, Antonov L, Stoyanova T, Karagiannidis P, Aslanidis P. Thione–thiol tautomerism and stability of 2- and 4-mercaptopyridines and 2-mercaptopyrimidines. Can J Chem 1990;68(9):1482–9. doi: 10.1139/v90-227
- Varynskyi BA, Scherback MA, Kaplaushenko AG, Yurchenko IA. The study of thione–thiol tautomerism of 4-amino-5-(4-nitrophenyl)-2,4-dihydro-3H-1,2,4-triazole-3-thione by HPLC–MS method. J Chem Pharm Res 2014;6(5):1342–50.
- Cheah IK, Halliwell B. Ergothioneine; antioxidant potential, physiological function and role in disease. Biochim Biophys Acta 2012;1822(5):784–93. doi: 10.1016/j.bbadis.2011.09.017
- Trotta RJ, Sullivan SG, Stern A. Lipid peroxidation and haemoglobin degradation in red blood cells exposed to t-butyl hydroperoxide. The relative roles of haem- and glutathione-dependent decomposition of t-butyl hydroperoxide and membrane lipid hydroperoxides in lipid peroxidation and haemolysis. Biochem J 1983;212(3):759–72. doi: 10.1042/bj2120759
- Thornalley PJ, Trotta RJ, Stern A. Free radical involvement in the oxidative phenomena induced by tert-butyl hydroperoxide in erythrocytes. Biochim Biophys Acta 1983;759(1–2):16–22. doi: 10.1016/0304-4165(83)90183-6
- Munday R. Toxicity of thiols and disulphides: involvement of free-radical species. Free Radic Biol Med 1989;7:659–73. doi: 10.1016/0891-5849(89)90147-0