Abstract
Objective: Pentoxifylline has previously been shown to increase haemoglobin levels in patients with chronic kidney disease (CKD) and erythropoietin-stimulating agent (ESA)-hyporesponsive anaemia in the HERO multi-centre double-blind, randomized controlled trial. The present study evaluated the effects of pentoxifylline on oxidative stress in ESA-hyporesponsive CKD patients.
Methods: This sub-study of the HERO trial compared 15 patients in the pentoxifylline arm (400 mg daily) and 17 in the matched placebo arm on oxidative stress markers: plasma total F2-isoprostanes, protein carbonyls, glutathione peroxidase (GPX), and superoxide dismutase (SOD) activities.
Results: Pentoxifylline did not significantly alter total F2-isoprostanes (adjusted mean difference (MD) 35.01 pg/ml, P = 0.11), SOD activity (MD 0.82 U/ml, P = 0.07), GPX activity (MD −6.06 U/l, P = 0.09), or protein carbonyls (MD −0.04 nmol/mg, P = 0.52). Replicating results from the main study, pentoxifylline significantly increased haemoglobin concentration compared with controls (MD 7.2 g/l, P = 0.04).
Conclusions: Pentoxifylline did not alter oxidative stress biomarkers, suggesting that alternative mechanisms may be responsible for the agent's ability to augment haemoglobin levels in CKD patients with ESA-hyporesponsive anaemia.
Introduction
Anaemia is a common complication of chronic kidney disease (CKD), especially in patients with end-stage renal disease (ESRD). A recent cross-sectional analysis of participants in the US National Health and Nutrition Examination Survey (NHANES) showed that the prevalence of anaemia in CKD patients during 2007–2010 was 15.4%, rising from 8.4% in stage 1 CKD to 53.4% in stage 5 CKD.Citation1 Although erythropoiesis-stimulating agents (ESA) are widely recommended for anaemia management in CKD,Citation2 approximately 10% of patients demonstrate ESA hyporesponsiveness,Citation3 which in turn is associated with a greatly increased mortality risk.Citation4
Among the factors associated with ESA hyporesponsiveness, oxidative stress has recently attracted significant attention,Citation5 contributing to increased patient morbidity and mortality.Citation6 It results from imbalance between increased pro-oxidant and decreased antioxidant activity and is measured by oxidative markers of peroxidation of lipid, protein, and nucleic acid. In addition, it has been shown to promote inflammation and vice versa,Citation7 thereby accentuating ESA hyporesponsiveness.Citation7,Citation8
Recently, the Handling Erythropoietin Resistance with Oxpentifylline (HERO) trial, a multi-centre, double-blind, randomized, placebo-controlled trial involving 53 adult participants with stage 4 or 5 CKD (including dialysis) and ESA-resistant anaemia, demonstrated that patients randomly allocated to oral pentoxifylline 400 mg daily had significantly increased haemoglobin concentrations relative to a placebo control group after 4 months (MD 7.6 g/l, 95% CI: 1.7–13.5, P = 0.01).Citation9 Although previous non-randomized studies have reported that pentoxifylline-associated improvements in haemoglobin concentration in ESA-hyporesponsive patients might be related to inhibited production of pro-inflammatory cytokines,Citation10–Citation12 the HERO trial found no significant interactions for treatment group by C-reactive protein sub-group, suggesting that the observed beneficial effects of pentoxifylline may not be related to anti-inflammatory actions. No prior studies have examined the effects of pentoxifylline on oxidative stress.
In this pre-specified sub-study of the HERO trial, the impact of pentoxifylline on plasma concentrations of oxidative stress biomarkers (F2-isoprostanes, protein carbonyls, glutathione peroxidase (GPX), and superoxide dismutase (SOD)) was further evaluated in patients with ESA hyporesponsiveness.
Methods
Study design
The HERO trial was registered with the Australian New Zealand Clinical Trials Registry (registration number 12608000199314) and was approved by ethics committees at all participating centres. All patients provided written informed consent prior to trial participation. The trial protocol has been published previously.Citation13 Briefly, the trial included adult patients with stage 4 or 5 CKD (including dialysis patients) on a stable dose of either erythropoietin or darbepoetin for at least 8 weeks who had ESA-hyporesponsive anaemia for which there was no identifiable cause (such as iron deficiency, bleeding, inadequate dialysis, hyperparathyroidism, malignancy, or haematologic disorder). ESA-hyporesponsive anaemia was defined as a haemoglobin concentration ≤120 g/l and an ESA resistance index (ERI; calculated as weight-adjusted weekly ESA dose divided by haemoglobin concentration) ≥1 IU/kg/week/g/l for erythropoietin-treated patients and ≥0.005 µg/kg/week/g/l for darbepoetin-treated patients.Citation9 Participants were randomized in a 1:1 ratio across three variables (study site, CKD stage, and ESA class) to pentoxifylline (Trental®, Sanofi-Aventis, Sydney, Australia) 400 mg daily orally or identical matching placebo for a period of 4 months. All other management, including iron supplementation, was provided according to local unit protocols. Vitamin B, folic acid, and vitamin C supplementation were permitted, provided daily doses were kept constant throughout the study period.
Of the 53 participants in the HERO trial (26 pentoxifylline, 27 control), 32 consented to participate in the oxidative stress sub-study (15 pentoxifylline, 17 control). Plasma concentrations of four oxidative stress biomarkers (F2-isoprostanes, protein carbonyls, GPX, and SOD) were measured at baseline and 4 months and compared with changes in the outcome variables (particularly haemoglobin level).
Total F2-isoprostanes
Samples were analysed in duplicate using a method developed in our laboratory.Citation14 Total F2-isoprostanes were extracted from plasma after saponification with methanolic NaOH. Samples were spiked with 8-iso-PGF2α-d4 (Cayman Chemicals, USA) as an internal standard and incubated at 42°C for 60 minutes. Samples were then acidified to pH 3 with hydrochloric acid (HCl), and hexane added and samples mixed for 10 minutes before centrifugation. The supernatant was removed and the remaining solution extracted with ethyl acetate and dried under nitrogen. Samples were reconstituted with acetonitrile, transferred into vials with silanized glass inserts and dried. Derivatization with pentafluorobenzylbromide and diisopropylethylamine and incubation at room temperature for 30 minutes followed. Samples were then dried under nitrogen before pyridine, bis(trimethylsilyl) trifluoroacetamide 99% and trimethylchlorosilane 1% were added and incubated at 45°C for 20 minutes. Finally, hexane was added and samples were mixed, then 1 μl was injected for analysis using gas chromatography tandem mass spectrometry (Varian, Australia) in negative chemical ionization mode. The laboratory coefficient of variation for this assay was 4.5%.
Protein carbonyls
Protein carbonyls were analysed using an adapted version of the methodology from Levine et al.Citation15 Duplicate plasma samples were incubated with 2,4-dinitrophenylhydrazine in 2.5 M HCl for 1 hour in the dark. Plasma blanks were incubated in 2.5 M HCl only. All samples were then precipitated with 20% trichloroacetic acid (TCA) on ice and centrifuged at 10 000g for 10 minutes. Supernatants were discarded and the pellets resuspended in 10% TCA and again centrifuged as above. Supernatants were removed and the pellets resuspended in 1:1 ethanol:ethylacetate solution. After centrifugation as above the pellets were washed twice more with the ethanol:ethylacetate solution. Pellets were then resuspended in 6 M guanidine hydrochloride solution and 220 µl of samples and blanks were transferred to microplate wells and absorbance read at 370 nm with correction at 650 nm using a microplate reader (Fluostar Optima, BMG Labtech, Offenburg, Germany). Protein carbonyl concentration was normalized to plasma protein content measured using a Pierce BCA protein assay kit (Thermo Scientific, Australia). The laboratory coefficient of variation for this assay was 11.9%.
Superoxide dismutase
Plasma SOD activity was quantified by modifying a method that measures the ability of the enzyme to inhibit reduction of the tetrazolium dye, 3-(4,5-dimethylthiazol-2-yl)-2,5-diphenyltetrazolium bromide (MTT).Citation16 Samples were analysed in duplicate with 300 µl of either a 4:1 or a 5:1 plasma sample (this depends on which dilution will give inhibition in the range of between 40 and 60% – see below) added to 120 µl of a 3:5 chloroform/ethanol solution. This was vortexed for approximately 90 seconds and then centrifuged for 5 minutes at 4000g. 50 µl of the top layer containing ethanol/water and SOD was removed and added to 750 µl buffer. The solution (diluted extract) was vortexed and left on ice until assay. The assay mixture consisted of 25–40 µl of the diluted extract (depending on obtaining inhibition in the range of between 40 and 60% – see below), 75 µl of 1 mM hypoxanthine, 50 µl of 1.25 mM MTT, 150 µl of 15 mU/ml of xanthine oxidase (XO) and a volume of potassium phosphate monobasic/borate/EDTA buffer (20.8 mM phosphate, 15.6 mM sodium borate, and 0.5 mM EDTA, pH 8.2) in an eppendorf tube. The volume of potassium phosphate monobasic/borate/EDTA buffer was selected to obtain a final volume of 750 µl (for example if 25 µl of the diluted extract was used, then 450 µl of the buffer would be used). This was incubated for 20 minutes at 37°C and then the reaction was terminated with the addition of 750 µl of isopropyl alcohol, which also helped to dissolve the MTT formazan crystals formed. The eppendorf tubes were then vortexed and 300 µl aliquots were loaded into wells on a 96-well microplate and absorbance measured at 540 nm on a microtitre plate reader (FLUOstar Optima, BMG Labtech, Germany). One unit of SOD was defined as the amount of SOD required to inhibit the MTT reduction by 50%. Inhibition was calculated based on the absorbances of the two blanks (0 and 100% superoxide production – corresponding to 100 and 0% inhibition, respectively). Zero% superoxide production was prepared in the same manner as the samples with the XO enzyme and sample eliminated and the total volume adjusted to 750 µl with buffer. The 100% superoxide production tube was prepared as per the 0% tube with the inclusion of the XO enzyme. The laboratory coefficient of variation for this assay was 7.6%.
Glutathione peroxidase
Plasma GPX activity was measured via the oxidation of NADPH to NADP+ by modifying methods.Citation17 Samples were analysed in duplicate. Plasma was diluted to 200:1 with HPLC grade water. The main reagent was made by mixing 9.25 ml of 100 mM KH2PO4 buffer containing 1 mM EDTA (pH 7.0), 250 µl of 100 U/ml glutathione reductase, 500 µl of 23 mM glutathione and 1.25 ml of 2 mM NADPH in 11 mM NaHCO3. The main reagent and sample (24 µl of 1:200 hemolysate plus 30 µl of deionized H2O added by the Cobas) were added to the cuvette and the absorbance monitored at 340 nm for 200 s on a Cobas Mira automated spectrophotometer (Roche Diagnostics, Switzerland). 18.5 µl of mM tert-butyl hydroperoxide plus 16 µl of deionized H2O were then added as start reagent and the absorbance was monitored for another 225 seconds. The difference in absorbance per minute between the two steps was used to calculate the enzyme activity. The laboratory coefficient of variation for this assay was 2.4%.
Erythropoietic outcomes
ERI, the primary outcome from the main study, was calculated as the weight-adjusted weekly ESA dose divided by haemoglobin concentration. ERI for darbepoetin-treated patients was converted to an erythropoietin-equivalent value using a dose conversion factor of 200:1.Citation18 Secondary outcomes from the main study were: (1) haemoglobin concentration; (2) ESA dose; (3) serum ferritin; (4) serum transferrin saturation; and (5) reticulocyte count.
Statistical analysis
Results are expressed as frequencies for categorical variables, mean ± SD for continuous normally distributed variables and median (inter-quartile range) for continuous non-normally distributed variables. All outcomes were analysed in accordance with the intention-to-treat principle. Treatment groups were compared on plasma levels of oxidative stress biomarkers and other outcomes at 4 months, adjusted for baseline values of each outcome of interest, using analysis of covariance. Associations between baseline measurements of oxidative stress markers and changes in haemoglobin and ERI were assessed using a Pearson's correlation test. P values <0.05 were considered statistically significant.
Results
Patients
The baseline characteristics of the oxidative stress sub-study participants, including levels of oxidative stress markers, ESA and ERI, were comparable between the pentoxifylline and control groups (Table ) and with those of the main HERO trial study groups.Citation19 All patients, except one in the control group, were in stage 5 CKD and undergoing haemodialysis.
Table 1 Baseline characteristics by treatment group
Oxidative stress markers
At the end of the 4-month study period, baseline adjusted mean plasma total F2-isoprostanes and SOD activities were higher in the pentoxifylline group compared with controls, while baseline adjusted mean protein carbonyls and plasma GPX activities were lower. However, these differences were not statistically significant (Table ). Changes in plasma total F2-isoprostanes, GPX, SOD, and protein carbonyls from baseline to month 4 in the pentoxifylline and control groups are shown in Figs. –, respectively.
Figure 1 Plasma total F2-isoprostane concentrations at baseline and 4 months in the pentoxifylline and control groups.

Figure 2 Plasma GPX concentrations at baseline and 4 months in the pentoxifylline and control groups.
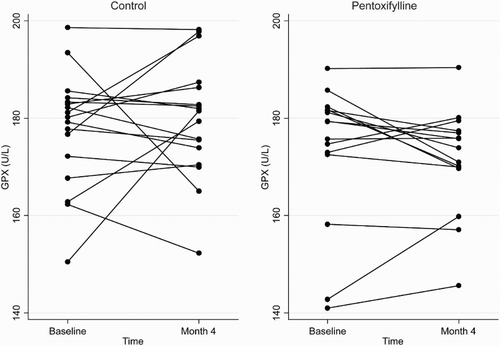
Figure 3 Plasma SOD concentrations at baseline and 4 months in the pentoxifylline and control groups.
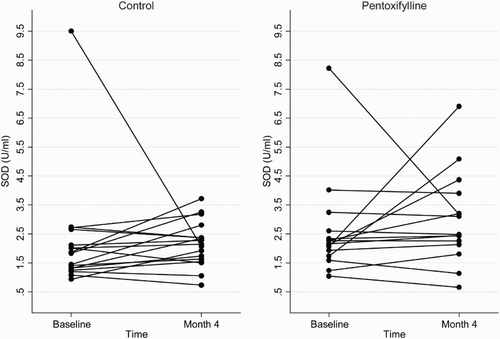
Figure 4 Plasma protein carbonyl concentrations at baseline and 4 months in the pentoxifylline and control groups.
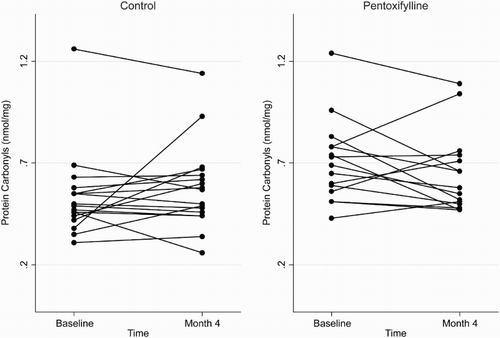
Table 2 Oxidative stress markers at 4 months by treatment group (mean values and mean differences adjusted for baseline values)
Erythopoietic outcomes
Baseline adjusted mean haemoglobin concentration at the end of the 4-month study period was significantly higher in the pentoxifylline group compared with controls (adjusted mean difference 7.2 g/l, 95% CI: 0.3–14.2, P = 0.04; Table ). No significant differences were observed between the two groups with respect to ERI, ESA dose, serum ferritin, serum transferrin saturation, or reticulocyte count after adjustment for their baseline levels (Table ).
Table 3 Primary and secondary outcomes at 4 months by treatment group (mean values and mean differences adjusted for baseline values)
Correlation between baseline oxidative stress markers and outcomes
Baseline measurements of oxidative stress markers were correlated with haemoglobin and ERI measured at baseline, 4 months, and the change from baseline to 4 months. All correlations were relatively small and mostly not statistically significant (Table ).
Table 4 Correlation between baseline oxidative stress markers and haemoglobin, ESA dose, and ESA resistance index
Discussion
This pre-specified sub-study of the HERO randomized controlled trial demonstrated that oral administration of pentoxifylline in a dose of 400 mg daily for 4 months did not significantly modify plasma concentrations of the oxidative stress biomarkers, total F2-isoprostanes, protein carbonyls, GPX, or SOD. This is the first examination of oxidative stress in the setting of CKD and ESA-hyporesponsive anaemia.
Previous studies have demonstrated evidence of increased oxidative stress in CKD progression.Citation20 For example, F2-isoprostanes, which are considered the gold standard measure of lipid peroxidative damage, are substantially elevated in patients with CKD,Citation21 especially those receiving chronic haemodialysis (HD),Citation22 related to healthy people. Indeed, they may be a more reliable marker of oxidative stress in HD, since they are not removed by HD.Citation23 Protein carbonyls, a measure of protein oxidation, are also increased in CKD patientsCitation20,Citation24 and are negatively correlated with estimated glomerular filtration rate (eGFR).Citation25 On the other hand, the activities of the antioxidant enzymes, SOD (which converts superoxide to hydrogen peroxide) and GPX (which is responsible for the conversion of hydrogen peroxide and other organic peroxides to water and oxygen),Citation26 are lower in HD patients than healthy controls.Citation27
Enhanced oxidative stress in CKD patients may result in ESA-hyporesponsive anaemia,Citation5,Citation7,Citation10 by mediating alterations in cellular iron homeostasis, leading to functional iron deficiency,Citation5 and reduction of erythrocyte life spanCitation28 and erythropoietin (EPO) synthesis.Citation29 Decreased SOD and GPX activities were both correlated with lower haemoglobin, higher EPO requirements,Citation8 and poorer ESA response in haemodialysis patients.Citation30 However, the results of the present study suggest that pentoxifylline increased haemoglobin levels in ESA-hyporesponsive, anaemic CKD patients with decreased ESA dose and ERI by mechanisms other than mitigation of oxidative stress.
Similar to these findings, Rusu et al.Citation30 reported that antioxidant treatment with vitamin E 400 mg/day for 8 weeks improved ESA responsiveness, increased haemoglobin levels, and decreased EPO dosage in patients with an ERI greater than 200 U/week/haematocrit. Moreover, a meta-analysis of six randomized controlled trials (n = 326, range 18–153) of ascorbic acid administration in addition to standard anaemia management found that ascorbic acid resulted in an increase in haemoglobin concentration and a decrease in EPO requirements.Citation31 However, these studies were limited by short duration and generally suboptimal methodological quality related to unclear randomization process, unclear allocation concealment, lack of blinding, heterogeneity among trial populations, and poor reporting of adverse events. Furthermore, none of these studies examined changes in plasma levels of oxidative stress markers so it is uncertain whether the observed effects of ascorbic acid were mediated by reduced oxidative stress or by other mechanisms.
In this respect, it should be noted that previous studies have not observed effects of ascorbic acid and vitamin E on oxidative stress biomarkers in patients with CKD. In one study reported by Kamgar et al.,Citation32 plasma F2-isoprostane and protein carbonyls levels in ESRD patients remained unchanged after 8 weeks of treatment with combination antioxidant therapy (including vitamin E 800 IU and vitamin C 250 mg daily), compared with placebo. Furthermore, in a randomized, double-blind, placebo-controlled, clinical trial, Himmelfarb et al.Citation33 reported that treatment of haemodialysis patients with gamma-tocopherol (vitamin E) and docosahexaenoic acid (vitamin C) for 8 weeks did not significantly change plasma levels of F2-isoprostane and protein carbonyls compared with placebo. Similar to these results, the present sub-study did not observe any significant difference in these oxidative stress markers between groups.
A strength of this sub-study was the fact that it used optimized methods for quantifying oxidative stress markers that have been shown to be reliable, sensitive, and reproducible.Citation14 The study was also performed within the context of multinational, multi-centre randomized controlled trial, such that the internal and external validity of the findings were high. Balanced against these strengths, the study was limited by a relatively small sample size, such that the possibility of a type 2 statistical error cannot be discounted. The other limitation was that not all participants had complete samples for oxidative stress analyses, raising the possibility of ascertainment bias. Furthermore, although four key biomarkers of oxidative stress were examined, it is possible that different results may have been obtained if erythrocytes rather than plasma were used or if other oxidative stress markers had been examined, such as F2-isofurans, malondialdehyde or 4-hydroxynonenal. Finally, the conversion of darbepoetin dose to an erythropoietin-equivalent value using the recommended conversion factor of 200:1 is not exact and potentially introduced variability, although this was mitigated by the inclusion of ESA class in the adaptive randomization allocation algorithm, which balanced erythropoietin and darbepoetin use between each group.
Conclusions
In conclusion, pentoxifylline did not alter oxidative stress biomarkers, suggesting that alternative mechanisms may be responsible for the agent's ability to augment haemoglobin levels in CKD patients with ESA-hyporesponsive anaemia. Further research into the possible mechanisms by which pentoxifylline corrects ESA-hyporesponsive anaemia, including larger trials to ascertain the influence of oxidative stress on haemoglobin response, are warranted.
Collaborators
Trial Management Committee (in addition to Writing Committee)
Emmanuel d'Almeida (Department of Nephrology, John Hunter Hospital, Newcastle, Australia); Rob Fassett (Department of Nephrology, Royal Brisbane and Women's Hospital, Brisbane, Australia); Carl Kirkpatrick (Center for Medicine Use and Safety, Monash University, Melbourne, Australia); Richard Phoon (Department of Nephrology, Westmead Hospital, Sydney, Australia).
Data and Safety Monitoring Board
Andrew Tonkin (Chair), Department of Epidemiology & Preventive Medicine, Monash University, Melbourne, Australia; Andrew Forbes (Statistician), Department of Epidemiology & Preventive Medicine, Monash University, Melbourne, Australia; Adeera Levin, Department of Medicine, University of British Columbia, Vancouver, Canada; David C. Wheeler, Center for Nephrology, Royal Free and University College Medical School, London, the United Kingdom.
Investigators
New South Wales: Concord Repatriation General Hospital (Meg Jardine, Jenny Burman, Samantha Hand); John Hunter Hospital (Emmanuel D'Almeida, Leanne Garvey); Liverpool Hospital (Michael Suranyi, Margaret Gilbert); Prince of Wales (Zoltan Endre, Katheen McNamara); Royal Prince Alfred Hospital (Paul Snelling, Jenny Burman, Samantha Hand); Queensland: Nambour General Hospital (Kumar Mahadevan, Andrea Pollock); Princess Alexandra Hospital (David Johnson, Diana Leary); Royal Brisbane Women's Hospital (Sharad Ratanjee, Julie Kirby); South Australia: Flinders Medical Center (Rajiv Juneja, Kathy Hill); Victoria: Austin Health (Natasha Cook, Pascal Bisscheroux); Eastern Health Integrated Renal Service-Box Hill Hospital (Lawrence McMahon, Annette Kent); Royal Melbourne Hospital (Eugenie Pedagogos, Matija Raspudic); Western Australia: Fremantle Hospital (Paolo Ferrari, Uli Steinwandel); Sir Charles Gairdner Hospital (Sharan Dogra, Susan Pellicano).
Project Management Team
Australasian Kidney Trials Network, Brisbane, Queensland, Australia (Alicia Morrish, Elaine Pascoe, Peta-Anne Paul-Brent, Donna Reidlinger, Anish Scaria, Liza Vergara).
Disclaimer statements
Contributors L.Z. undertook primary analysis of data for this manuscript, interpreted results, wrote first draft of the manuscript, read and approved final manuscript. David Johnson was the principal investigator. He conceived the study, participated in design and co-ordination, data analysis and interpretation of results, helped to draft the manuscript, and read and approved the final manuscript. Sunil Badve participated in design and co-ordination, data interpretation and analysis, drafting of the manuscript, and read and approved the final manuscript. E.P. and A.S. were the trial statisticians; they drafted the Statistical Analysis Plan, performed the statistical analyses, participated in interpretation of the results, helped to draft the manuscript, and read and approved the final manuscript. All other authors were members of the HERO Trial Management Committee. They participated in study design, data analysis, and interpretation of results, read and commented on interim drafts and approved the final manuscript. The trial collaborators were lead investigators and study co-ordinators at local study sites. They participated in study design at the initial investigators’ meeting, oversaw local data collection, and read and approved the final manuscript.
Funding None.
Conflicts of interest D.W.J. has previously received consultancy fees from Sanofi-Aventis. He has also previously received consultancy fees, speakers’ honoraria, research grants, and travel sponsorships from Amgen, Roche, and Janssen-Cilag. He was the recipient of a Roche Foundation for Anaemia Research (RoFAR) Grant, which partly funded the HERO trial, and a Queensland Government Health Research Fellowship. Carmel Hawley has previously received consultancy fees, speakers’ honoraria, research grants and/or travel sponsorships from Amgen, Roche, and Janssen-Cilag. A.C. has previously received consultancy fees, speakers’ honoraria and/or research grants from Amgen, Roche, Baxter, Fresenius, and Merck. R.W. has previously received consultancy fees, speakers’ honoraria, research grants and travel sponsorships from Amgen, Roche, and Janssen-Cilag and has served on Advisory Boards for Amgen, Roche, and Janssen-Cilag. All other authors have no conflict of interest to declare.
Ethics approval The HERO trial was registered with the Australian New Zealand Clinical Trials Registry (registration number 12608000199314) and was approved by ethics committees at all participating centres.
Acknowledgements
Support: The HERO trial was funded by research grants from Roche Foundation for Anaemia Research (RoFAR), Amgen, Janssen-Cilag and the National Health and Medical Research Council of Australia. The funders had no role in study design; collection, analysis, and interpretation of data; writing the report; or the decision to submit the report for publication.
References
- Stauffer M, Fan T. Prevalence of anemia in chronic kidney disease in the United States. PLoS One 2014;9(1):e84943.
- Kdoqi. KDOQI Clinical Practice Guideline and Clinical Practice Recommendations for anemia in chronic kidney disease: 2007 update of hemoglobin target. Am J Kidney Dis 2007;50(3):471–530.
- Hung SC, Lin YP, Tarng DC. Erythropoiesis-stimulating agents in chronic kidney disease: what have we learned in 25 years? J Formos Med Assoc 2014;113(1):3–10.
- Solomon SD, Uno H, Lewis EF, Eckardt KU, Lin J, Burdmann EA, et al. Erythropoietic response and outcomes in kidney disease and type 2 diabetes. N Engl J Med 2010;363(12):1146–55.
- Smrzova J, Balla J, Barany P. Inflammation and resistance to erythropoiesis-stimulating agents–what do we know and what needs to be clarified? Nephrol Dial Transplant2005;20(Suppl. 8):viii2–7.
- Locatelli F, Canaud B, Eckardt KU, Stenvinkel P, Wanner C, Zoccali C. Oxidative stress in end-stage renal disease: an emerging threat to patient outcome. Nephrol Dial Transplant 2003;18(7):1272–80.
- Stenvinkel P. Anaemia and inflammation: what are the implications for the nephrologist? Nephrol Dial Transplant 2003;18(Suppl. 8):viii17–22.
- Tutal E, Sezer S, Bilgic A, Aldemir D, Turkoglu S, Demirel O, et al. Influence of oxidative stress and inflammation on rHuEPO requirements of hemodialysis patients with CRP values ‘in normal range’. Transplant Proc 2007;39(10):3035–40.
- Johnson DW, Pascoe EM, Badve SV, Dalziel K, Cass A, Clarke P, et al. A randomized, placebo-controlled trial of pentoxifylline on erythropoiesis-stimulating agent hyporesponsiveness in anemic patients with CKD: the handling erythropoietin resistance with oxpentifylline (HERO) trial. Am J Kidney Dis 2015;65(1):49–57.
- Iain CM,, Cooper AC. Erythropoietin resistance: the role of inflammation and pro-inflammatory cytokines. Nephrol Dial Transplant 2002;17(Suppl. 11):39–43.
- Cooper A, Mikhail A, Lethbridge M, Kemeny D, Macdougall I. Pentoxifylline improves hemoglobin levels in patients with erythropoietin-resistant anemia in renal failure. J Am Soc Nephrol 2004;15(7):1877–82.
- Mohammadpour A, Nazemian F, Khaiat M, Tafaghodi M, Salari P, Charkazi S, et al. Evaluation of the effect of pentoxifylline on erythropoietin-resistant anemia in hemodialysis patients. Saudi J Kidney Dis Transpl 2014;25(1):73–8.
- Johnson DW, Hawley CM, Rosser B, Beller E, Thompson C, Fassett RG, et al. Oxpentifylline versus placebo in the treatment of erythropoietin-resistant anaemia: a randomized controlled trial. BMC Nephrol 2008;9:8.
- Briskey D, Wilson G, Fassett R, Coombes J. Optimized method for quantification of total F(2)-isoprostanes using gas chromatography-tandem mass spectrometry. J Pharm Biomed Anal 2014;90:161–6.
- Levine R, Garland D, Oliver C, Amici A, Climent I, Lenz A, et al. Determination of carbonyl content in oxidatively modified proteins. Methods Enzymol 1990;186:464–78.
- Madesh M, Balasubramanian K. Microtiter plate assay for superoxide dismutase using MTT reduction by superoxide. Indian J Biochem Biophys 1998;35(3):184–8.
- Wheeler C, Salzman J, Elsayed N, Omaye S, Korte DJ. Automated assays for superoxide dismutase, catalase, glutathione peroxidase, and glutathione reductase activity. Anal Biochem 1990;184(2):193–9.
- Roger S, Cooper B. What is the practical conversion dose when changing from epoetin alfa to darbepoetin outside of clinical trials? Nephrology (Carlton) 2004;9(4):223–8.
- Johnson DW, Pascoe EM, Badve SV, Dalziel K, Cass A, BEc PC, et al. A randomized, placebo-controlled trial of pentoxifylline on erythropoiesis-stimulating agent hyporesponsiveness in anemic patients with CKD: the Handling Erythropoietin Resistance with Oxpentifylline (HERO) trial. Am J Kidney Dis 2015;65(1):49–57.
- Oberg BP, McMenamin E, Lucas FL, McMonagle E, Morrow J, Ikizler TA, et al. Increased prevalence of oxidant stress and inflammation in patients with moderate to severe chronic kidney disease. Kidney Int 2004;65(3):1009–16.
- Ramos LF, Shintani A, Ikizler TA, Himmelfarb J. Oxidative stress and inflammation are associated with adiposity in moderate to severe CKD. J Am Soc Nephrol 2008;19(3):593–9.
- Handelman GJ, Walter MF, Adhikarla R, Gross J, Dallal GE, Levin NW, et al. Elevated plasma F2-isoprostanes in patients on long-term hemodialysis. Kidney Int 2001;59(5):1960–6.
- Wiswedel I, Peter D, Gardemann A, Carluccio F, Hampl H, Siems W. Serum concentrations of F2-isoprostanes and 4-hydroxynonenal in hemodialysis patients in relation to inflammation and renal anemia. Biomark Insights 2008;3:419–28.
- Tbahriti HF, Kaddous A, Bouchenak M, Mekki K. Effect of different stages of chronic kidney disease and renal replacement therapies on oxidant-antioxidant balance in uremic patients. Biochem Res Int 2013;2013:358985.
- Aveles PR, Criminacio CR, Goncalves S, Bignelli AT, Claro LM, Siqueira SS, et al. Association between biomarkers of carbonyl stress with increased systemic inflammatory response in different stages of chronic kidney disease and after renal transplantation. Nephron Clin Pract 2010;116(4):c294–9.
- Zachara BA, Gromadzińska J, Wąsowicz W, Zbróg Z. Red blood cell and plasma glutathione peroxidase activities and selenium concentration in patients with chronic kidney disease: a review. Acta Biochim Pol 2006;53(4):663–77.
- Iasioli S, Schiavon R, Petrosino L, Cavallini L, De Fanti E, Zambello A, et al. Do different dialytic techniques have different atherosclerotic and antioxidant activities? ASAIO J 2001;47(5):516–21.
- Gallucci M, Lubrano R, Meloni C, Morosetti M, Manca di Villahermosa S, Scoppi P, et al. Red blood cell membrane lipid peroxidation and resistance to erythropoietin therapy in hemodialysis patients. Clin Nephrol 1999;52(4):239–45.
- Suliman HB, Ali M, Piantadosi CA. Superoxide dismutase-3 promotes full expression of the EPO response to hypoxia. Blood 2004;104(1):43–50.
- Rusu A, Rusu F, Zalutchi D, Muresan A, Gherman Caprioara M, Kacso I. The influence of vitamin E supplementation on erythropoietin responsiveness in chronic hemodialysis patients with low levels of erythrocyte superoxide dismutase. Int Urol Nephrol 2013;45(2):495–501.
- Deved V, Poyah P, James MT, Tonelli M, Manns BJ, Walsh M, et al. Ascorbic acid for anemia management in hemodialysis patients: a systematic review and meta-analysis. Am J Kidney Dis 2009;54(6):1089–97.
- Kamgar M, Zaldivar F, Vaziri N, Pahl M. Antioxidant therapy does not ameliorate oxidative stress and inflammation in patients with end-stage renal disease. J Natl Med Assoc 2009;101(4):336–44.
- Himmelfarb J, Phinney S, Ikizler TA, Kane J, McMonagle E, Miller G. Gamma-tocopherol and docosahexaenoic acid decrease inflammation in dialysis patients. J Ren Nutr 2007;17(5):296–304.