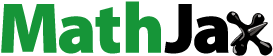
Abstract
Objectives: This study is an extension to our finding of direct anti-oxidant activities of lanthanide(III) complexes with the heterocyclic compound, 5-aminoorotic acid (AOA). In this experiment, we used AOA and coumarin-3-carboxylic acid as the two heterocyclic compounds with anti-oxidant potential, to produce the complexes with different lanthanides.
Methods: Lanthanide(III) complexes were tested on the iron-driven Fenton reaction. The product of this reaction, the hydroxyl radical, was detected by HPLC.
Results: All complexes as well as their ligands had positive or neutral effect on the Fenton reaction but their behavior was different. Both pure ligands in low concentration ratio to iron were inefficient in contrast to some of their complexes. Complexes of neodymium, samarium, gadolinium, and partly of cerium blocked the Fenton reaction at very low ratios (in relation to iron) but the effect disappeared at higher ratios. In contrast, lanthanum complexes appeared to be the most promising. Both blocked the Fenton reaction in a dose-dependent manner.
Conclusion: Lanthanide(III) complexes were proven to block the iron-driven production of the hydroxyl radical. Second, the lanthanide(III) element appears to be crucial for the anti-oxidant effect. Overall, lanthanum complexes may be promising direct anti-oxidants for future testing.
Introduction
Anti-oxidants have the ability to protect cellular organelles from free radical oxidative damage but the majority can act as pro-oxidants as well, apparently due their high reducing capacity. Citation1–Citation3 Such behavior can also be found in anti-oxidant compounds with low or moderate iron-binding capacity. Citation2 These compounds can behave very differently depending on their ratio to iron, due to mixed effects, e.g. direct scavenging of free radicals like the hydroxyl radical, iron reduction, and chelation. For this reason, a given compound can be pro- and anti-oxidant depending on other conditions.
The lanthanides have not been largely examined from biological point of view but it appears to possess some interesting pharmacological activities. It needs no discussion that the lanthanides have been investigated and widely described from the chemical viewpoint and they provide fascinating new possibilities for research in the coming decades. Their great potential derives in part from their intrinsic anti-oxidant properties. Citation4,Citation5 Lanthanide inhibition of reactive oxygen species (ROS) propagation involves the strong oxyphilicity inherent in the lanthanides. The availability of oxygen sites on these free radicals makes them excellent targets for Ln(III) coordination.
In addition to the lanthanides, various heterocyclic compounds also have anti-oxidant properties. This study forms a continuation of our interest in the coordination chemistry of the heterocyclic compounds and their lanthanide(III) complexes.
One heterocyclic compound, orotic acid, an intermediate in pyrimidine biosynthesis, and its derivatives are promising as future drugs. Citation6,Citation7 One of these derivatives, 5-aminoorotic acid (AOA, Fig. ) which has relatively unknown coordination chemistry, was analyzed in this study.
Coumarins are very widely distributed in nature and some have proven anti-bacterial, Citation8 anti-fungal, Citation9 anti-inflammatory, Citation10 anti-coagulant, Citation11 anti-HIV, Citation12,Citation13 and anti-tumor activity. Citation14–Citation19 The ability of coumarins to bind metal ions represents Citation20 an additional mean of modulating their pharmacological responses. It has been found that the binding of a metal to the coumarin moiety retains or enhances its biological activity. Citation15–Citation17 The position and the type of the substituent attached to the aromatic ring of coumarin molecules have a pronounced influence on the anti-oxidant activity of these compounds. Citation21
In a series of papers, we investigated the molecular structure, energetic, and spectroscopic properties of different coumarin derivatives and their lanthanide complexes, as well as their pharmacological activity. Citation15–Citation17 , Citation22–Citation26 These studies highlighted the potential for developing novel lanthanide complexes mainly as anti-cancer therapeutics. However, more analogs are required to obtain better activity profiles for this class of compounds.
This study is an extension of our earlier publications on the synthesis of metal complexes with biologically active ligands to include the anti-oxidant activity of AOA (Fig. ), coumarin-3-carboxylic acid (CCA, Fig. ), and their lanthanide(III) complexes. The intention was to investigate the lanthanide(III) effect, bearing in mind the therapeutic importance of orotic acid derivatives with a substituent in position 5 of the pyrimidine nucleus and the activity of 3-substituted coumarins. Since the above mentioned impact of concentration ratio between the tested compound and iron is high, this study tested both types of lanthanide(III) complexes in different concentrations in order to analyze in detail their effects on iron-driven production of the highly reactive hydroxyl radical using a previously established method. Citation2,Citation27
Material and methods
Materials
Ferrous sulfate heptahydrate, ferric chloride, hydroxylamine, ferrozine, sodium acetate, acetic acid, 4-(2-hydroxyethyl)-1-piperazineethanesulfonic acid (HEPES), HEPES sodium salt, salicylic acid, 2,3-dihydroxybenzoic acid, and 2,5-dihydroxybenzoic acid were purchased from Sigma-Aldrich Inc. (St Louis, USA), hydrogen peroxide was from Coopharma s.r.o. (Prague, Czech Republic), phosphoric acid was from Lachema a.s. (Brno, Czech Republic), and dimethyl sulfoxide (DMSO) and methanol for HPLC, super gradient grade, were from Lach-Ner s.r.o (Neratovice, Czech Republic).
Complexes
The synthesis of the lanthanide(III) complexes of AOA and CCA as well as their theoretical, analytical, spectral, and structural characterization have been previously reported by us. Citation22–Citation26 , Citation28,Citation29 Shortly: the compounds used for preparing the solutions were Merck products, p.a. grade: Ce(NO3)3·6H2O, La(NO3)3·6H2O, Nd(NO3)3·6H2O, Sm(NO3)3·6H2O, Gd(NO3)3·6H2O, and Dy(NO3)3·5H2O. CCA and AOA (Sigma-Aldrich) were used for the preparation of metal complexes as ligands.
The complexes of CCA were synthesized by reaction of cerium(III), lanthanum(III), neodymium(III), samarium(III), gadolinium(III), and dysprosium(III) inorganic salts and the ligand, in amounts equal to metal/ligand molar ratio of 1:2. The synthesis of the complexes was achieved using different ratios (1:1, 1:2, 1:3) but in all cases the product had a composition of 1:2. The complexes were prepared by adding ethanol solutions of Ln(III) salts to ethanol solutions of the ligand. The reaction mixture was stirred with an electromagnetic stirrer at 25°C for 1 hour and at the moment of mixing, precipitates were obtained. These were filtered, washed several times with water and ethanol, and dried in a desiccator to constant weight. The complexes were insoluble in water, partly soluble in methanol and ethanol, and soluble in DMSO. The data from the elemental analysis of the obtained compounds served as the basis for determining their empirical formulae: Ce(CCA)2(NO3)·H2O, La(CCA)2(NO3)·2H2O, Nd(CCA)2(NO3)·H2O, Sm(CCA)2(NO3)·H2O, Gd(CCA)2(NO3)·H2O, and Dy(CCA)2(NO3)·H2O, where .
The complexes of AOA were synthesized by reaction of cerium(III), lanthanum(III), and neodymium(III) salts and the ligand, in amounts equal to a metal/ligand molar ratio of 1:3. The complexes were prepared by adding an aqueous solution of cerium(III), lanthanum(III), and neodymium(III) salts to an aqueous solution of the ligand, subsequently raising the pH of the mixture gradually to ca. 5.0 by adding a dilute solution of sodium hydroxide. The reaction mixture was stirred with an electromagnetic stirrer at 25°C for 1 hour. At the moment of mixing of the solutions, precipitates were obtained. These were filtered, washed several times with water, and dried in a desiccator to constant weight. The complexes were insoluble in water, partly soluble in methanol and ethanol, and soluble in DMSO. The obtained formula for the Ln(III) complexes of AOA are as follows: Ce(AOA)3·3H2O, La(AOA)3·3H2O, and Nd(AOA)3·3H2O, where .
Fenton chemistry experiments
This method is based on trapping hydroxyl radicals by an indicator (salicylic acid) with the resulting production of 2,3-dihydroxybenzoic acid and 2,5-dihydroxybenzoic acids, which are then spectrophotometrically measured using HPLC. Citation27
Sample preparation
Freshly prepared solution (200 µl) of ferrous ions in ultrapure water (5 mM) was mixed with the same volume of methanolic solution consisting of tested compound (different concentrations) and salicylic acid (25 mM). After 2 minutes of mixing, 200 µl of hydrogen peroxide (25 mM) in ultrapure water were added and the mixture was analyzed by HPLC.
HPLC-DAD method for analysis of hydroxyl radical production
A Dionex Ultimate 3000 liquid chromatograph equipped with diode array detector was used. Spectra were recorded for all peaks in the wavelength range of 190–750 nm.
For the separation of salicylic acid, 2,3-dihydroxybenzoic acid, and 2,5-dihydroxybenzoic acid, an Eclipse Plus C18 column (4.6 × 100 mm, 3.5 μm, Agilent, Santa Clara, CA) was used. The mobile phase consisted of 40% methanol and 60% of aqueous solution of phosphoric acid (0.085%) and isocratic elution was carried out for 6 minutes. The flow rate of the mobile phase was 1.2 ml/min. For quantification, six-point calibration curves were obtained with pure standards. Identification of substances in samples was accomplished by comparing elution times and spectral characteristics with the standards. All experiments were checked by addition of an internal standard (known amounts of 2,3-dihydroxybenzoic and 2,5-dihydroxybenzoic acids). The results were calculated as the mean of the sample analysis (250 µl) (1) mixed with 50 µl methanol and (2) with added internal standards (50 µl of methanolic solution of 2,3-dihydroxybenzoic and 2,5-dihydroxybenzoic acids).
Iron chelation and reduction experiments
These methods are based on a sensitive indicator for ferrous ions – ferrozine. The complex of ferrozine with ferrous ions is then measured at 562 nm by spectrophotometry. Non-chelated or reduced ferric ions can be visualized after reduction with a suitable reductant (hydroxylamine). Citation20
Shortly, 50 µl of AOA or CCA solutions in DMSO in various concentrations were mixed in different 15 mM buffers (150 µl; acetate for pH 4.5 and 5.5 and HEPES for pH 6.8 and 7.5) with 50 µl of ferrous or ferric ions (250 µM) dissolved in ultrapure water for 2 minutes. In the case of ferric chelation, hydroxylamine at a concentration of 10 mM was then added to reduce non-chelated ferric to ferrous ions. Ferrozine (50 µl, 10 mM) was then added and absorbance measured immediately and after 5 minutes using the Synergy HT Multi-Detection Microplate Reader (BioTek Instruments, Inc., Winooski, Vermont, USA). In all experiments, the solvent (DMSO) was used as a control. In iron reduction experiments, 10 mM of hydroxylamine was used as well as positive control (100% of iron reduction).
Statistical analysis
The data on tested compounds for each ratio were analyzed using the two-way ANOVA with Tukey multiple comparison test. For iron reduction experiments, the percent reduction was compared by use of t-test vs. the blank sample with DMSO. The minimal relevant level of significance was considered at P < 0.05. Results are shown as means ± SEM.
Results and discussion
Given that the ligands used are well-known biologically active compounds, we compared them with the lanthanide(III) complexes which we have characterized. Citation22–Citation26 , Citation28,Citation29 Detailed analysis of AOA and Ln(III)–AOA systems revealed that the binding mode in the complexes was bidentate through the carboxylic oxygen atoms. It has been suggested that CCA binds to Ln(III) ions through both oxygen atoms of the carboxylic and carbonylic groups.
All lanthanide complexes as well as the pure ligands were tested for iron-mediated hydroxyl radical formation (the Fenton reaction). All tested compounds had neutral or positive effect on this reaction: no compound behaved as a pro-oxidant in any of the concentrations tested. However, there were marked differences between compounds related to their anti-oxidant activity. In general, two types of behavior were observed: (a) progressively anti-oxidant, i.e. with increasing ratio of the tested compound to iron, inhibition of the Fenton reaction increased or (b) bell-shaped, i.e. inhibition of the Fenton reaction was observed in lower ratios but at higher ratios, the effect was neutral.
Both ligands alone had slight anti-oxidant effects but at much higher concentrations than their complexes. In the case of AOA, the anti-oxidant effect was apparent in the ratio 2:1, AOA to Fe2+, respectively, while in the case of CCA from the ratio 1:1 (Fig. A). These differences aside, the curves were different only at the ratio 0.5 (1:2, ligand to the Fe2+, respectively). Since their effects on Fenton chemistry were quite small, we tested the interaction of both ligands with iron. In line with their low effect on Fenton chemistry, CCA did not significantly chelate or reduce iron at any of the tested conditions (data not shown), while AOA chelated only ferric but not ferrous iron at pH 4.5 (Fig. A). However, its chelation activity was low, expressed particularly in higher ratios, and markedly dropped after 5 minutes, suggesting that the complex of AOA with Fe3+ is not very stable. Similarly, AOA reduced iron but this reduction was low, reaching a maximum of 4–20% of reduced iron at the ratio 100:1, AOA/Fe respectively, depending on pH (Fig. B). These data reporting only modest or no interaction of ligands with iron suggest that the mild inhibitory effects of both ligands on Fenton chemistry are caused by their direct interaction with the hydroxyl radical (direct anti-oxidant effect).
Figure 3 Effect of complexants and their lanthanide complexes on Fenton reaction. (A) Comparison of ligands, (B) AOA and its lanthanide(III) complexes, and (C and D) CCA and its lanthanide(III) complexes. *P < 0.05 vs. AOA or CCA. Dotted area represents the error of the method. The ratio of the compound to Fe is equal to the ratio of the lanthanide to Fe.
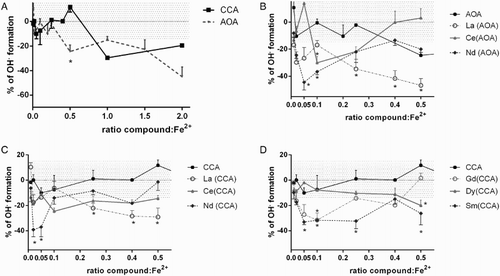
Figure 4 Interaction of AOA with iron. The upper part (A) shows iron chelation by AOA. The bottom part (B) shows ferric reduction by AOA. The statistical significance for iron reduction is shown at P < 0.05 by arrows for different pH conditions. If not specified, the graphs show results of measurements done 5 minutes after addition of ferrozine.
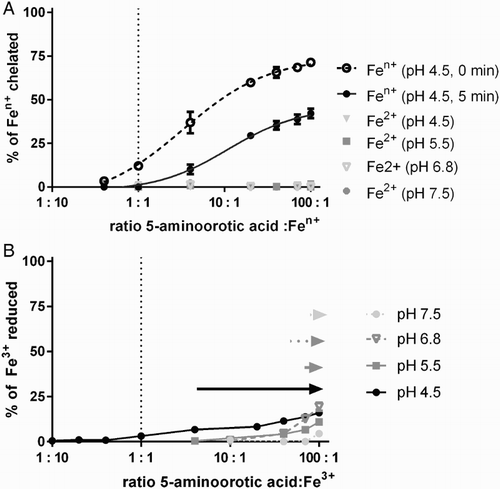
In the case of AOA complexes, both types of behaviors described in relation to Fenton chemistry were observed (Fig. B). The neodymium complex was very active at low ratios. Compared to the ligand, AOA at ratios 1:20 (i.e. 0.05) and 1:10 (0.1), the neodymium–AOA–complex was significantly more active (P < 0.001). The cerium complex in the ratio 1:10 (P < 0.05) was also more active than AOA while the lanthanum complex was not significantly active in this ratio. On the other hand, both cerium and neodymium complexes corresponded to bell-shaped curves and their anti-oxidant activity disappeared with increasing concentrations while lanthanum complex behaved as progressive anti-oxidant and whose activity was apparent and progressive from the ratio of 1:4.
In the case of lanthanide complexes with CCA, the results were analogous but the activity was lower than the corresponding lanthanide–AOA–complex. This signifies that again, neodymium-formed complexes were clearly active at low ratios (ratios 1:50 and 1:20, P < 0.01 vs. CCA, Fig. C) but similarly active to the ligand CCA at higher ratios while the lanthanum complex was again progressively anti-oxidant and its anti-oxidant effect occurred as well from the ratio of 1:4. The only exception was the cerium complex, which was almost inactive. Of the other tested complexes, of particular note was the samarium complex with CCA (Fig. D) which was active from the ratio of 1:20 to 1:4 (P < 0.05). The gadolinium–CCA–complex formed bell-shaped curves (active at the ratio of 1:10, P < 0.05) too. The dysprosium complex was inactive at low ratios but significant although mild activity was observed at the ratio of 1:2 (P < 0.001).
Certainly the involvement of the lanthanides in ROS removal is quite different from the inhibition of ROS by organic compounds. Most organic anti-oxidants scavenge free radicals by single electron exchange with radicals, thus transforming themselves into radicals and hence act as ‘pro-oxidants’ even in the absence of a free transient metal. A lanthanide(III) very easily interacts with either free radicals or peroxides but it is not transformed into a radical. However, mechanistic understanding of the role of lanthanide(III) as a scavenger of reactive oxidative species is very scanty. The lability of lanthanide complexes, strong oxyphilicity, rapid water exchange reactions, the non-directionality of lanthanide ligand bonds, and varying coordination numbers, all contribute to lanthanide interaction with biomolecules. The ionic size of lanthanide(III) varies from one to another lanthanide. In addition, the ionic size of a particular lanthanide also varies significantly with the respective coordination number. This can explain the different coordinating potential as well as different biological behavior of the respective lanthanides under different pathophysiological conditions. The importance of concentration/ratio of tested compound to metal is crucial (e.g. Fig. ), as it is for other anti-oxidants. In our preliminary analysis of only one concentration, there was a small difference between the acute effects of the AOA and its complexes. Citation26
There are two major issues coming from the study. Firstly, as expected, lanthanide(III) complexes alone did not potentiate the Fenton chemistry but blocked it or had neutral effect depending on lanthanide present in the complex. This may be an important factor compared to classical anti-oxidants. As far we know, the most potent inhibitor of the Fenton chemistry in low concentrations is the flavonoid taxifolin which is very efficient in extremely low ratios of flavonoid to iron (1:1000) but in approaching the ratio 1:1, its activity ceases. Citation2 In this study, the tested complexes of neodymium and gadolinium and partly as well that of cerium behaved similarly but their potency was lower and the maximum activity was observed approximately in the ratio of 1:20. This feature may limit their use in practice. Lanthanum complexes are of much greater interest, since they inhibited the Fenton reaction in a concentration-dependent manner. For comparison, such desired activity has only been observed in some non-chelating flavonoids or strong iron chelators such as deferoxamine. Citation2
Secondly, the resulting effect on Fenton chemistry depended on the lanthanide(III) element in particular, as can be seen from Fig. . However, the potency can be clearly modulated by selection of ligand. This is of particular interest, since some of these complexes may be unstable in plasma. Citation26 In solution as in this study, the complexes, at least in low ratios, were likely stable as their activity was different from and higher than pure ligands in most cases. However, it is possible that some lanthanide(III) complexes which have bell-shaped effects on Fenton chemistry are less stable in higher ratios and therefore their curves approach those of the ligands. The serious drawback for testing the complexes is their low solubility in the reaction medium. It would be of interest in future studies to analyze ratios in which the complex is in much higher concentrations than iron. This will necessitate other method with higher sensitivity which will enable to decrease the concentration of both the iron and the complex.
In conclusion, this study extends our previous results showing preliminary anti-oxidant effects of lanthanide complexes of AOA. The results show that (1) lanthanide(III) complexes with both AOA and CCA had positive or neutral effect on hydroxyl radical production by Fenton chemistry and (2) the lanthanide(III) element seems to be crucial for the anti-oxidant effect.
Disclaimer statements
Contributors J.M.: experiments, P.M. and L.S.: study design and coordination, I.K.: synthesis of tested compounds, P.M. and I.K.: preparation of the manuscript.
Funding The work was supported by the grant of The Czech Science Foundation (project No. P303/12/G163). I.K. acknowledges the financial support from the University Grant Commission (project No. 32/ 2014).
Conflicts of interest None.
Ethics approval This work does not include animals and it is not a clinical study.
Acknowledgements
We would like to thank Dr Alexander Oulton for English correction.
References
- Laughton MJ , Halliwell B , Evans PJ , Hoult JR . Antioxidant and pro-oxidant actions of the plant phenolics quercetin, gossypol and myricetin. Effects on lipid peroxidation, hydroxyl radical generation and bleomycin-dependent damage to DNA. Biochem Pharmacol 1989;38(17):2859–65. doi: 10.1016/0006-2952(89)90442-5
- Macakova K , Mladenka P , Filipsky T , Riha M , Jahodar L , Trejtnar F , et al. Iron reduction potentiates hydroxyl radical formation only in flavonols. Food Chem 2012;135(4):2584–92. doi: 10.1016/j.foodchem.2012.06.107
- Prochazkova D , Bousova I , Wilhelmova N . Antioxidant and prooxidant properties of flavonoids. Fitoterapia 2011;82(4):513–23. doi: 10.1016/j.fitote.2011.01.018
- Misra SN , Ramchandriah G , Gagnani MA , Shukla RS , Devi MI . Absorption spectral studies involving 4f–4f transitions as structural probe in chemical and biochemical reactions and compositional dependence of intensity parameters. Appl Spectrosc Rev 2003;38(4):433–93. doi: 10.1081/ASR-120026330
- Wang K , Li RC , Cheng Y , Zhu B . Lanthanides – the future drugs? Coord Chem Rev 1999;192:297–308. doi: 10.1016/S0010-8545(99)00072-7
- Helios K , Duczmal M , Pietraszko A , Michalska D . The first Ni(II) complexes of 5-nitroorotate ligand with the tridentate and bidentate coordination modes. Crystal and molecular structures, vibrational spectra and magnetic properties. Polyhedron 2013;49(1):259–68. doi: 10.1016/j.poly.2012.10.018
- Michalska D , Hernik K , Wysokinski R , Morzyk-Ociepa B , Pietraszko A . Copper(II)–pi interaction in cis-[Cu(orotato)(NH3)2] and the crystal structure of [Cu(orotato)(H2O)4]·H2O: X-ray, vibrational spectroscopy and density functional study. Polyhedron 2007;26(15):4303–13. doi: 10.1016/j.poly.2007.05.052
- Azizian J , Mohammadi AA , Bidar I , Mirzaei P . KAl(SO4)2·12H2O (alum) a reusable catalyst for the synthesis of some 4-substituted coumarins via Pechmann reaction under solvent-free conditions. Monatsh Chem 2008;139(7):805–8. doi: 10.1007/s00706-007-0833-9
- Satyanarayana VSV , Sreevani P , Sivakumar A , Vijayakumar V . Synthesis and antimicrobial activity of new Schiff bases containing coumarin moiety and their spectral characterization. ARKIVOC 2008;2008(17):221–33.
- Garazd MM , Muzychka OV , Vovk AI , Nagorichna IV , Ogorodniichuk AS . Modified coumarins. 27. Synthesis and antioxidant activity of 3-substituted 5,7-dihydroxy-4-methylcoumarins. Chem Nat Compd 2007;43(1):19–23. doi: 10.1007/s10600-007-0055-8
- Smitha G , Reddy CS . ZrCl4-catalyzed Pechmann reaction: synthesis of coumarins under solvent-free conditions. Synth Commun 2004;34(21):3997–4003. doi: 10.1081/SCC-200034821
- Kostova I . Coumarins as inhibitors of HIV reverse transcriptase. Current HIV Res 2006;4(3):347–63. doi: 10.2174/157016206777709393
- Kotali A , Lafazanis IS , Harris PA . Synthesis of 6,7-diacylcoumarins via the transformation of a hydroxy into a carbonyl group. Synth Commun 2008;38(22):3996–4006. doi: 10.1080/00397910802250911
- Kostova I . Synthetic and natural coumarins as cytotoxic agents. Curr Med Chem Anticancer Agents 2005;5(1):29–46. doi: 10.2174/1568011053352550
- Kostova I , Momekov G . New cerium(III) complexes of coumarins – synthesis, characterization and cytotoxicity evaluation. Eur J Med Chem 2008;43(1):178–88. doi: 10.1016/j.ejmech.2007.03.007
- Kostova I , Stefanova T . Synthesis, characterization and cytotoxic/cytostatic activity of La(III) and Dy(III) complexes. J Trace Elem Med Biol 2010;24(1):7–13. doi: 10.1016/j.jtemb.2009.06.004
- Kostova I , Trendafilova N , Momekov G . Theoretical, spectral characterization and antineoplastic activity of new lanthanide complexes. J Trace Elem Med Biol 2008;22(2):100–11. doi: 10.1016/j.jtemb.2007.10.005
- Nofal ZM , El-Zahar MI , El-Karim SSA . Novel coumarin derivatives with expected biological activity. Molecules 2000;5(2):99–113. doi: 10.3390/50200099
- Sashidhara KV , Kumar A , Kumar M , Srivastava A , Puri A . Synthesis and antihyperlipidemic activity of novel coumarin bisindole derivatives. Bioorg Med Chem Lett 2010;20(22):6504–7. doi: 10.1016/j.bmcl.2010.09.055
- Mladenka P , Macakova K , Zatloukalova L , Rehakova Z , Singh BK , Prasad AK , et al. In vitro interactions of coumarins with iron. Biochimie 2010;92(9):1108–14. doi: 10.1016/j.biochi.2010.03.025
- Balabani A , Hadjipavlou-Litina DJ , Litinas KE , Mainou M , Tsironi CC , Vronteli A . Synthesis and biological evaluation of (2,5-dihydro-1H-pyrrol-1-yl)-2H-chromen-2-ones as free radical scavengers. Eur J Med Chem 2011;46(12):5894–901. doi: 10.1016/j.ejmech.2011.09.053
- Kostova I , Rastogi VK , Kiefer W , Kostovski A . New lanthanum (III) complex – synthesis, characterization, and cytotoxic activity. Arch Pharm (Weinheim) 2006;339(11):598–607. doi: 10.1002/ardp.200600077
- Kostova I , Rastogi VK , Kiefer W , Kostovski A . New cerium(III) and neodymium(III) complexes as (III) cytotoxic agents. Appl Organomet Chem 2006;20(8):483–93. doi: 10.1002/aoc.1113
- Kostova I , Peica N , Kiefer W . Theoretical and spectroscopic studies of lanthanum (III) complex of 5-aminoorotic acid. Chem Phys 2006;327(2–3):494–505. doi: 10.1016/j.chemphys.2006.05.029
- Kostova I , Peica N , Kiefer W . Theoretical and spectroscopic studies of 5-aminoorotic acid and its new lanthanide(III) complexes. J Raman Spectrosc 2007;38(2):205–16. doi: 10.1002/jrs.1625
- Kostova I , Traykova M , Rastogi VK . New lanthanide complexes with antioxidant activity. Med Chem 2008;4(4):371–8. doi: 10.2174/157340608784872181
- Nappi AJ , Vass E . Hydroxyl radical formation via iron-mediated Fenton chemistry is inhibited by methylated catechols. Biochim Biophys Acta 1998;1425(1):159–67. doi: 10.1016/S0304-4165(98)00062-2
- Kostova I , Momekov G . Synthesis, characterization and cytotoxicity evaluation of new cerium(III), lanthanum(III) and neodymium(III) complexes. Appl Organomet Chem 2007;21(4):226–33. doi: 10.1002/aoc.1205
- Kostova I , Momekov G , Stancheva P . New samarium(III), gadolinium(III), and dysprosium(III) complexes of coumarin-3-carboxylic acid as antiproliferative agents. Met-Based Drugs 2007;2007:15925. doi: 10.1155/2007/15925