Abstract
Objectives: The study was focused on the activity of propolis from Amaicha del Valle, Argentina (ProAV) as a promoter and scavenger of Riboflavin (Rf) – photogenerated reactive oxygen species (ROS).
Methods: Through a kinetic and mechanistic study, employing stationary and time-resolved photochemical and electrochemical techniques, the protecting activity of ProAV was investigated.
Results: In the absence of light and Rf, ProAV exerted a relatively efficient inhibitory effect on 1,1-diphenyl-2-picrylhydrazyl radicals and acts as a protector of artificially promoted linoleic acid oxidation. Under aerobic visible-light-irradiation conditions, in the presence of Rf as the only light-absorber species, a complex picture of competitive processes takes place, starting with the quenching of singlet and triplet electronically excited states of Rf by ProAV. The species O2(1 g), O2•−, H2O2, and OH• are generated and interact with ProAV.
Discussion: ProAV behaves as an efficient ROS scavenger. It is scarcely photo-oxidized by interaction with the mentioned ROS. Quantitative results indicate that ProAV is even more resistant to photo-oxidation than the recognized antioxidant trolox. Two dihydroxychalcones, mostly present in the ProAV composition, are responsible for the protecting activity of the propolis.
Introduction
A wide variety of potentially harmful agents such as free radicals and reactive oxygen species (ROS) can be generated in the human body and in different kinds of foods. Organisms have several ways to protect oneself against such species, and most of these defensive measures involve the use of antioxidants that inhibit or delay the oxidation of other molecules by preventing the initiation or propagation of oxidizing chain reactions. There are two basic categories of antioxidants: synthetic and natural. Both types are generally formed by compounds containing phenolic structures.Citation1–Citation3 Synthetic antioxidants such as butylated hydroxyanisole and butylated hydroxytoluene (BHT) have been employed since the early 1900s; however, there are restrictions on the use of these compounds, due to their own potentially detrimental effects on human.Citation4,Citation5 Thus, interest in natural antioxidants has considerably increased the amount of research into different types of native substances.Citation6,Citation7
Useful natural antioxidants may turn out to be found in the preparations of Apis bees. Apis bees prepare a resin-like material named propolis to fill holes and embalm predators that have died inside their hives. This material is composed of plant resins, bees wax, and secretions from worker bees’ head glands. Importantly, propolis has shown biological activity, and it has, therefore, been introduced it as a potentially active principle for pharmaceutical preparations.Citation8–Citation10
These biological properties are mainly ascribed to a few substances, such as polyphenols, flavonoids, phenolic acids, and their esters among which flavonoids in particular are considered top contributors.Citation11,Citation12 Most research regarding antioxidants has in fact been devoted to the antioxidant activity of flavonoids, due to their ability to reduce free radical formation and scavenge oxidative agents.Citation13
Regarding to the study of antioxidants, it is possible to use photochemical techniques.
Riboflavin (Rf), also known as vitamin B2, is one of the most important natural absorbers of visible light and is present in a wide variety of biological systems and foods. It has been postulated to be a sensitizer for photopromoted reactions (mainly photo-oxidations) that can produce physiological changes in surrounding molecules.Citation14–Citation16 It is well known that Rf and its derivatives generate the ROS singlet molecular oxygen (O2(1Δg)) and superoxide radical anion (O2•─) upon adequate photoirradiation.Citation17–Citation19 In the presence of an electron donor, photogeneration of ROS hydrogen peroxide (H2O2) and hydroxy radical (OH•) by Rf has also been reported.Citation20,Citation21
This manuscript describes the results of our systematic kinetic study on the potential reactive interactions of Rf-photogenerated ROS with propolis and two chalcones present in this molecule. Specifically, we measured the activities of propolis both as a promoter and scavenger of Rf-photogenerated ROS and evaluated its stability in the presence of degradative species.
Experimental
Materials
A propolis sample (ProAV) was collected from beehives in Amaicha del Valle (Tucumán, Argentina) and stored at –18 °C.
Rf, superoxide dismutase (SOD) from bovine erythrocytes, catalase (CAT) from bovine liver; mannitol, l-tryptophan, beta-carotene, Folin–Ciocalteu's phenol reagent, and mono deuterated MeOH (MeOD) were purchased from Sigma Chemical. Co.; Rose Bengal (RB), sodium azide (NaN3), and furfuryl alcohol (FFA), were provided by Aldrich, Merck, and Riedel de Haën, respectively. The radical 1,1-diphenyl-2-picrylhydrazyl (DPPH) was purchased from Aldrich. HPLC-quality methanol (MeOH) was acquired from Sintorgan. MeOD was employed in the time-resolved determinations of O2(1Δg) phosphorescence to increase its lifetime. Water was triply distilled. An ethanolic extract of propolis ProAV (EE) was obtained as described below, and 2′,4′-dihydroxychalcone (diOHCh) and 2′,4′-dihydroxy-3′-methoxychalcone (diOHMeCh) were isolatedCitation22 in the laboratory of Dr Alejandro Tapia of the University of San Juan in San Juan, Argentina.
Methods
Preparation of propolis EE and isolation of diOHCh and diOHMeCh
EE was prepared according to the method described by Szliszka et al.Citation23 The extraction yield was 69%. Isolation of the chalcones diOHCh and diOHMeCh from Zuccagnia punctata Cav. collected in Amaicha del Valle was performed according to the methods of Agüero et al.Citation22
Total phenolic compounds
Total polyphenol (TP) contents in EE were determined by the Folin–Ciocalteau colorimetric method, according to Kumazawa et al.Citation24 An EE solution was mixed with the Folin–Ciocalteau reagent, and 0.5 ml 10% Na2CO3. EE was analyzed at a final concentration of 20 μg/ml. The total polyphenol content was expressed as grams of gallic acid (GA) equivalent/100 g of sample.
Free radical scavenging activity on DPPH
The free radical scavenging effects of ProAV and catechin (as a reference) were assessed by the fading of an ethanolic solution of DPPH radical as previously reported by Lima et al.Citation25 EE was assayed at concentrations of 100 μg/ml. The fading percentage was calculated as follows:(1) Color loss indicated the free radical scavenging efficiency.
Antioxidant activity on linoleic acid oxidation
Analysis of antioxidant activity on linoleic acid oxidation was carried out using a modified version of the method developed by Ahn et al.Citation26 We dissolved 5 mg of βbeta-carotene in a solution of 10 ml of chloroform and 134 µl of linoleic acid, and then added 380 µl of Tween 20. Test samples were evaluated at a final concentration of 90 μg/ml, and BHT was used as the reference. The percent antioxidant activity was expressed relative to the initial absorbance of the sample during a 300-minute incubation using equation (Equation2(2) ):
(2) where % AA is the percent antioxidant activity; Ao is the initial absorbance at 470 nm and t = 0; At is the absorbance at 470 nm and measurement time t (intervals of 60 minutes for 300 minutes).
Absorption and fluorescence experiments
Ground-state absorption spectra were recorded using a Hewlett Packard 8453 diode array spectrophotometer. For stationary Rf fluorescence experiments, an RF 5301-PC Shimadzu spectrofluorimeter was used. The excitation and emission wavelengths were 446 and 515 nm, respectively.
A classical Stern–Volmer treatment of the data was applied using equation (Equation3(3) ), where I0 and I are the respective fluorescence intensities of Rf in the presence and absence of the compound that eventually acts as a quencher, Q, and Ksv (Ksv = 1
kq × 1
τ0) is the Stern–Volmer constant for the quenching of excited singlet Rf (1Rf*), with 1
kq (see Scheme ) and 1
τ0 being the rate constants for the dynamic quenching of 1Rf* and lifetime of 1Rf*, respectively.
(3)
Time-resolved O2(1Δg) phosphorescence detection
The total quenching rate constant (kt, see Scheme ) for O2(1Δg) deactivation by Q was determined from the near-infrared time-resolved phosphorescence, and employing equation (Equation4(4) ), in which τ and τ0 are the O2(1Δg) lifetimes in the presence and in the absence of Q, respectively.
(4) The 532-nm wavelength output from a Nd:Yag laser (Spectron) was used as the excitation source. The emitted (O2(1Δg)) phosphorescence at 1270 nm was detected at right angles using an Edinburgh Instruments EI-P germanium detector, after having passed through a 1270-nm interference filter and two Wratten filters. The output of the detector was coupled to a 400-MHz digital oscilloscope (HP 54504A) and to a personal computer for signal processing. Air-equilibrated solutions were employed in all cases.
Stationary photolysis and oxygen uptake experiments
Stationary aerobic photolysis of aqueous solutions containing ProAV and either Rf or RB were carried out in a PTI unit, provided with a high-pass monochromator and 150-W Xe lamp irradiating at a wavelength of 445 ± 10 nm or in a home-made photolyser for five non-monochromatic irradiation (150 W quartz-halogen lamp). In the latter case, 430-nm wavelength cutoff filters were used.
The Rf- or RB-sensitized photo-oxygenation rates of ProAV, diOHCh, or diOHMeCh were determined by evaluation of the initial slopes of oxygen consumption vs. irradiation time, employing a specific oxygen electrode (Orion 97–08).
Reaction (chemical) rate constants for the interaction Q-O2(1Δg) (kr, see Scheme ) were obtained as described by Tratniek & Hoigné,Citation27 from the ratio of the first order slopes of the vitamin B6 family (B6D) and reference consumption, each at the same concentration, yielding kr/krR. The reference was FFA, with a krR = 1.2 × 108 M–1s–1.
The anaerobic rates of Rf photodecomposition were determined in the absence and presence of different concentrations of ProAv by the decrease in the 446-nm absorption band of the vitamin following 5-minute irradiation with visible light (wavelength cutoff of 430 nm) employing MeOH as a solvent in Ar-bubbled solution.
Results
Overall antioxidative activity of ProAV
The extraction yield for EE was 69.4% with a TP value of 22.3 expressed as grams of GA per 100 g EE.
Fig. A shows the radical scavenging activity of DPPH treated with various concentrations of ProAV, as well as DPPH treated with various concentrations of the radical trapper catechin.
Figure 1 (A) Percent DPPH radical scavenging activity by catechin (•●) and ProAV (□) as a function of substrate concentration. (B) Percent decrease of the absorbance at 470 nm as a function of incubation time of a solution of beta-carotene/linoleic acid in the absence (•●) and in the presence of BHT (▴▴) and ProAV (▪).
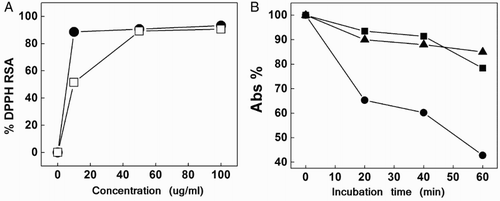
Fig. B shows the similar antioxidant activities of ProAV and the recognized artificial antioxidant BHTCitation28 on linoleic acid oxidation.
These preliminary assays roughly demonstrate the potential of ProAV as an overall defensive agent against aggressive oxidative species, but they do not provide detailed information about the fate of ProAV under oxidative stress conditions or data on the kinetic and mechanistic aspects governing such processes.
The Rf (A446 = 0.4)-sensitized photoirradiation of air-equilibrated solutions of ProAV (57 μg/ml) in MeOH-H2O (1:1;v/v) produces different changes in the absorption spectrum of the propolis with respect to the propolis sample without Rf. The irradiation wavelength was greater than 430 nm, and in a spectral region where only Rf absorbs the incident light. Fig. shows the difference absorption spectra of the mixture (Rf plus ProAV vs. Rf) at different irradiation times.
Figure 2 Changes in the UV-Vis absorption spectrum of MeOH-H2O (1:1; v/v) solutions containing Rf (A446 = 0.4) and 57 μg/ml ProAV vs. A446 = 0.4 upon irradiation with visible light. Inset (A) oxygen consumption vs. photoirradiation time for MeOH-H2O (1:1; v/v) solutions containing Rf (A446 = 0.40) plus (a) 38 μg/ml ProAV and (b) 38 μg/ml Trolox. Inset (B) changes in the UV-Vis absorption spectrum in a MeOH solution of RB (A630 = 0.4) and 64 μg/ml ProAV vs. RB (A630 = 0.4) upon irradiation with visible light. In all cases, a cutoff at 430 nm was used.
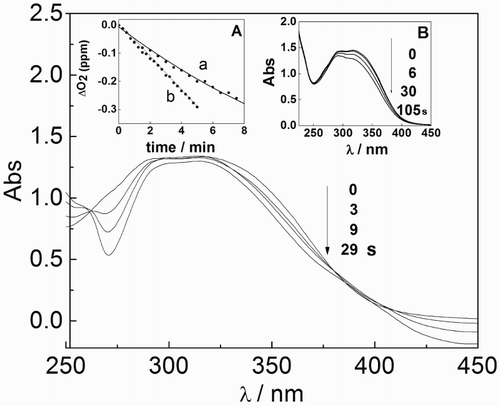
Parallel photoirradiation of a solution containing 38 μg/ml ProAV, but otherwise the same as that described above, gave rise to oxygen consumption. Oxygen uptake was not observed in the absence of light or ProAV. To standardize the evaluation of the oxygen uptake rate, the same experiment was performed by changing the ProAV concentration and employing an identical W/W concentration of the well-known artificial antioxidant Trolox.Citation29 The results are shown in the inset of Fig. .
These experiments suggest that ProAV can intercept Rf-photogenerated oxidative species. It is particularly relevant in this regard that (a) ProAV was chemically affected by irradiation with visible light in the presence of Rf as a dye sensitizer, (b) oxygenated species participated in the process, and (c) electronically excited states of Rf participated in the overall interaction.
Individualization of the active excited states of the sensitizer, as well as determination of optimal sensitizing conditions, oxygen requirements, and local concentrations necessary for the effective interaction of the involved reaction partners may help to elucidate and interpret the reaction mechanism. These issues were previously been discussed on the basis of the well-known reaction sequence shown in Scheme .Citation30 Q represents an electron donor species, namely ProAV in most of the experiments in this study. In some cases, the propolis sample was replaced with diOHCh or diOHMeCh to compare the behaviors of these substrates. Note that these flavonoid substrates were the most abundant antioxidant components detected in the ProAV sample.
According to Scheme , the absorption of incident light promotes Rf to electronically excited singlet (1Rf*) and triplet (3Rf*) states (5). Both states can be quenched by Q through reactions (Equation6(21) ) and (8) – the latter by means of an electron transfer process that produces the respective semireduced and semioxidized forms (i.e. Rf•− + Q•+). The species Rf•− is rapidly protonated. Reactions (10) through (12) represent the known generation of the ROS O2•−, OH•, and hydrogen peroxide (H2O2) upon interaction of the neutral radical of the vitamin with reactants naturally present in the medium. Direct generation of O2•− through quenching of 3Rf* by dissolved oxygen (step (7)) has been reported, with a quantum yield of 0.009.Citation17 Reaction (13) represents the eventual oxidation of Q by the generated ROS. An energy-transfer reaction from 3Rf* to ground-state triplet molecular oxygen O2(3Σ−
g) dissolved in the medium can occur, producing the excited-state oxygen species O2(1Δg) with a reported quantum yield of 0.49 in MeOH (reaction (14)).Citation31,Citation32 This species can decay either by a collision with surrounding solvent molecules (reaction (15)) or by interaction with Q and/or Rf through an exclusive physical interaction (reaction (16)) or chemical photo-oxidation (reaction (17)). The overall rate constant for O2(1Δg) quenching (kt) is defined as the sum of the rate constants for processes (16) and (17).
Quenching of 1Rf* and 3Rf*by ProAV
Rf yielded an intense fluorescence emission band centered at 515 nm (Fig. ), with a emission quantum yield (ΦF) of 0.25. In the presence of ProAV, the fluorescence quenching of 1Rf* produced a decrease in the stationary emission intensity, but the shape of the fluorescence spectrum did not change (Fig. , inset A). The main panel of Fig. shows the quenching plot, from which a Stern–Volmer constant of 7 × 10−4 L/(mg s−1) was obtained when employing Rf in MeOH (A446 = 0.1). The reported value for the fluorescence lifetime of Rf in MeOH is 2 ns.Citation17
Figure 3 Stern–Volmer plot for the quenching of 1Rf* by ProAV. I0 and I represent the stationary fluorescence intensities of Rf (A446 = 0.1) in the absence and presence of ProAV. Inset (A) (a) fluorescence spectrum of Rf (A446 = 0.1) in MeOH; (b) the same in the presence of 200 μg/ml ProAV. Inset (B) relative values for the rate of Rf (A446 = 0.2) degradation upon irradiation with 445 ± 10-nm wavelength light in argon-saturated methanolic solution in the absence (1 in the abscissa axis) and in the presence of 15, 36, and 60 μg/ml ProAV (2–4 in the abscissa axis, respectively).
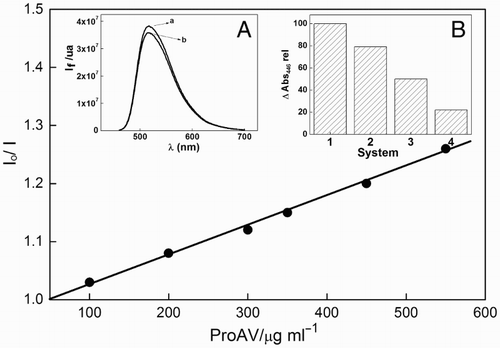
Anaerobic photodegradation of Rf under visible light predominantly proceeds through the triplet state, and the rate can be estimated with absorption spectroscopy. Irradiation of Ar-saturated solutions of Rf in MeOH in the absence and presence of different ProAV concentrations but otherwise under identical experimental conditions showed that this rate decreased as the concentration of the propolis increased from 0 to 60 μg/ml (Fig. , inset B). At these ProAV concentrations, practically no fluorescence quenching of Rf occurred. A simple calculation employing the Stern–Volmer constant for fluorescence quenching indicates that ∼7% inhibition of the 1Rf* emission should be expected for a ProAV concentration of 60 μg/ml. A decrease of ∼78% in the degradation rate of Rf was observed for this concentration of ProAV (Fig. , inset B). On this basis, the experimental data strongly support the idea that a long-lived triplet state, one that is an intermediate in the photolysis of Rf, can be efficiently quenched by relatively low concentrations of ProAV. The reported lifetime for 3Rf* in MeOH is 12 μs.
Quenching of O2(1Δg) by ProAV and diOHCh
The interaction of ProAV and diOHCh with O2(1Δg) was explored by employing time-resolved O2(1Δg) phosphorescence detection and RB as a dye sensitizer that generate O2(1Δg) in an exclusive fashion with a quantum yield of ∼0.7 in MeOH.Citation33 The RB-sensitized photoirradiation of ProAV (RB (A630 = 0.4)) in MeOH produced evident changes in the absorption spectra of the propolis sample (Fig. , inset B). In addition, the decrease of the emission lifetime of O2(1Δg) in the presence of 68 mg/l ProAV in MeOD (Fig. , inset) unambiguously demonstrates the O2(1Δg) quenching by the propolis sample with the oxidative species. Rate constant values kt = 3.4 × 107 M−1 s−1 and 2.8 × 107 M−1 s−1 were obtained for diOHCh and diOHMeCh in MeOD. These values are on the same order of magnitude as the kt values reported for other flavonoids.Citation33 The eventual kr value was tested by comparison with the well-known reference compound FFA.
Figure 4 Stern–Volmer plots for the quenching of O2(1Δg) phosphorescence by diOHCh in MeOD in the presence (a) and in the absence (b) of 10 mM NaOH. Inset: temporal decay of the phosphorescence intensity of O2(1Δg) in the absence (a) and presence (b) of 68 μg/ml ProAV in MeOD. Photosensitizer: RB (A532 = 0.18).
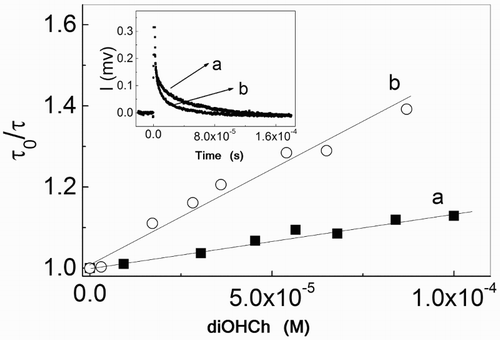
Figure 5 Bar graph of the relative rate values of oxygen uptake by MeOH-H2O (1:1, v/v) solutions of Rf (A446 = 0.4) and 38 µg/ml ProAV as a function of photoirradiation time (cutoff = 430 nm) in the absence (1) and presence of 10 μg/ml CAT (2); 10 μg/ml mannitol (3); 10 μg/ml SOD (4); and 5 mM NaN3 (5). Bar (6) corresponds to the relative rate value of oxygen uptake by Rf (A446 = 0.4) alone.
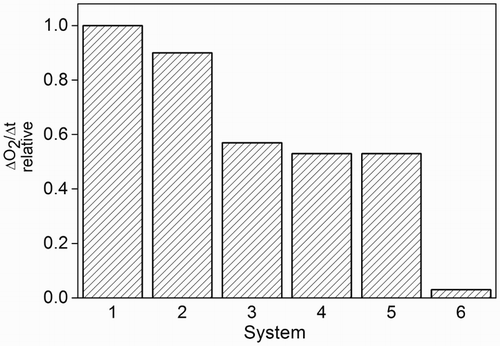
Practically no oxygen uptake by 0.5 mM diOHCh or diOHMeCh plus RB (A630 = 0.4) in MeOH was observed. Hence, they were both determined to have a kr value ˂10−6M−1 s−1. The kr/kt ratio, which indicates the fraction of overall quenching of O2(1Δg) by the substrate that effectively leads to a chemical transformation, indicates that the flavonoids are exclusive physical quenchers of the oxidative species.
The rate constant for diOHCh was also determined in the presence of 10 mM NaOH. Under this condition, the hydroxyl groups of the flavonoid are ionized, and a significant increase in the kt value should be observed.Citation34 In fact a kt value of 1.4 × 108 M−1 s−1 was determined for diOHCh in the methanolic alkaline medium. Experimental kinetic data for the interaction of diOHCh with O2(1Δg) are shown in the main panel of Fig. .
The interactions of ProAV and diOHCh with ROS
To evaluate the potential interactions of Rf-photogenerated ROS with ProAV, we carried out oxygen consumption experiments in the presence of specific ROS interceptors. The presence of 10 mM NaN3, 1 μg/ml SOD, 1 μg/ml CAT, and 10 mM mannitol was each observed to decrease the rate of oxygen uptake of MeOH-H2O (1:1, v/v) solutions containing Rf (A446 = 0.4) plus 38 mg/l ProAV as compared to that recorded in the additives’ absences. Similar experiments with ROS interceptors have been performed to assess the participation of O2(1Δg), O2•–, H2O2, and OH• in oxidative events.Citation35–Citation37 The enzyme SOD dismutates the O2•– species (reaction (20)), whereas CAT decomposes H2O2 (reaction (21)), mannitol deactivates the species OH• (reaction (22)), and NaN3 physically quenches O2(1Δg) (reaction (16)) (Scheme , with NaN3 instead of Q).(20)
(21)
(22)
Photoprotective effect of diOHMeCh toward tryptophan oxidation
To test the eventual protective effect of diOHMeCh on the ROS-mediated oxidation of proteins, we assessed Rf-sensitized photo-oxidation of the amino acid tryptophan (Trp).Citation29 The rates of oxygen consumption by 0.5 mM Trp, 0.5 mM diOHMeCh, and a mixture of both substrates in MeOH were determined. These rates are considered a measure of the global photo-oxidative progress and were determined by monitoring up to 15% of the conversion of the starting material. In relative terms, the obtained rate values were 1, 0.31, and 0.37 respectively. In other words, the rate of Trp photodegradation in the presence of diOHMeCh decreased by ∼63% relative to that in the absence of the polyphenol.
It is well known that Trp is photo-oxidizable upon Rf-sensitization.Citation38 The degradative process operates through a combination of radical-mediated and O2(1Δg)mediated mechanisms. Garcia and SilvaCitation38 reported on the generation of O2•–, H2O2, OH•, and O2(1Δg) in Rf-sensitized Trp photodegradation, and we determined a kr = 3.5 × 107 M−1 s−1 for reaction (17) with this amino acid instead of Q.Citation39
Discussion and conclusions
Overall antioxidant activity of ProAV
DPPH radical scavenging action and antioxidant activity toward linoleic acid clearly demonstrate the overall ability of ProAV to defend against such aggressive detrimental agents.Citation27,Citation28 The antioxidative action of ProAV is accompanied by its concomitant degradation. This degradation mainly involves an exclusively O2(1Δg)-mediated pathway in the case of RB sensitization and a group of radical-driven reactions in addition to the above-mentioned O2(1Δg) degradation in the case of Rf-sensitization. Nevertheless, the rate at which ProAV faded was kinetically moderate, and less than twice the rate of fading in experiments with Trolox (Fig. ), a recognized artificial antioxidant.Citation29
The promotion and quenching of ROS by ProAV
ProAV reacts with O2(1Δg), O2•–, H2O2, and OH•. The H2O2 and OH• species were not observed to spontaneously form during Rf photoirradiation. Hence, the initial action of ProAV could be considered as promoting the generation of these ROS. The use of this possible pathway has been demonstrated for a series of electron donors in the presence of photoirradiated Rf, including several flavonoids.Citation30
The radical reaction initially produces RfH• and ProAV•+ (reaction (8)). The latter species, representing one or more electron-donating components of propolis, possibly decays to products and/or reacts with ROS generated in the medium. 3Rf* could be quenched either by O2(3Σg−) (reaction (14)) or ProAV (reaction (8)). Whether one of these processes is kinetically dominant depends on the respective concentrations of the quenchers and on the values of the rate constants of reactions (8) and (14). Different flavonoids acting as efficient electron donors toward 3Rf*, a species that can simultaneously produce O2(1Δg).Citation40 The observation that under identical experimental conditions, the detection tests for O2(1Δg) on one hand and O2•–, H2O2, and OH• on the other hand, clearly indicates that under these conditions, pathways (8) and (14) are kinetically competitive to some extent.
Photoprotection of Trp photo-oxidation by diOHCh
The dominant physical scavenging of O2(1Δg) represents a positive result arising in the context of oxidative interactions between biomolecules. In this case, the very low values for the kr/kt quotients exhibited by diOHCh and by diOHMeCh are examples of a desirable quality. The final result is the elimination of O2(1Δg) without appreciable scavenger loss.
This activity protects proteins, DNA, and other cell matrix components.Citation41 This photoprotective effect exerted by diOHCh was evident based on the clear decrease in the rate of oxygen uptake of the system containing Trp and chalcone compared to that of the amino acid alone upon Rf sensitization.
Disclaimer statements
Contributors None.
Funding None.
Conflicts of interest There are no conflicts of interest.
Ethics approval Ethical approval is unnecessary.
References
- Larson RA. The antioxidants of higher plants. Phytochemistry 1988;27:969–78. doi: 10.1016/0031-9422(88)80254-1
- Hudson BJ. Food antioxidants. London: Elsevier Applied Food Science; 1990.
- Hall, III CA, Cuppett SL. Structure-activities of natural antioxidants. In: Aruoma O.I. and Cuppett S.L., (Eds.), Antioxidant methodology: In vivo and in vitro concepts. AOCS Press, Illinois; 1997. pp. 141–172.
- Branen AL. Toxicology and biochemistry of butylatedhydroxy anisole and butylatedhydroxy toluene. J Am Oil Chem Soc 1975;52:59–63. doi: 10.1007/BF02901825
- Ito N, Fukushima S, Hasegawa A, Shibata M, Ogiso T. Carcinogenicity of butylatedhydroxy anisole in F344 rats. J Natl Cancer Inst 1983;70:343–7.
- Löliger J. The use of antioxidants in foods. In: Aruoma OI, Halliwell B, (eds.) Free radicals and food additives. Taylor & Francis. London; 1991. pp. 121–50.
- Haung D, Ou B, Prior RL. The chemistry behind antioxidant capacity assays. J Agric Chem 2005;53:1841–56. doi: 10.1021/jf030723c
- Asís M. El propóleo. Un valioso producto apícola. In: CIDA, (ed.) Propóleo. El oro púrpura de las abejas. Ministerio de la Agricultura. La Habana; 1991. pp. 53–71.
- Guerra AG, Méndez RB. Principales aplicaciones. In: de la Torriente P, (ed.) Propóleos, un camino hacia la salud. Ministerio de la Agricultura. La Habana; 1997. pp. 47–59.
- Farré R, Frasquet I, Sanchez A. Propolis and human health. Ars Pharmaceutica 2004;45:21–43.
- De Vecchi E, Drago L. Propolis’ antimicrobial activity: what's new? Infez Med 2007;15:7–15.
- Luo C, Zou X, Li Y, Sun C, Jiang Y, Wu Z. Determination of flavonoids in propolis-rich functional foods by reversed phase high performance liquid chromatography with diode array detection. Food Chem 2011;127:314–20. doi: 10.1016/j.foodchem.2011.01.006
- Pietta PG. Flavonoids as antioxidants. J Nat Prod 2000;63:1035–42. doi: 10.1021/np9904509
- Heelis PF. The photochemistry of flavins. In: Muller F, (ed.) Chemistry and biochemistry of flavoenzymes. (CRS Press: Boca Raton, FL); 1991. Vol 1.
- Takimoto K, Tano K, Hashimoto M, Hori M, Akasaka S, Utsumi H. Delayed transfection of DNA after riboflavin mediated photosensitization increases G: C to C: G transversions of supF gene in Escherichia coli mutY strain. Mutat Res 1999;445:93–8. doi: 10.1016/S1383-5718(99)00138-2
- Yettella RR, Min DB. Effects of Trolox and ascorbic acid on the riboflavin photosensitized oxidation of aromatic amino acids. Food Chem 2010;118:35–41. doi: 10.1016/j.foodchem.2009.04.022
- Heelis PF. The photophysical and photochemical properties of flavins (isoalloxazines). Chem Soc Rev 1982;11:15–39. doi: 10.1039/cs9821100015
- Redmond RW, Gamlin JN. A compilation of singlet oxygen yields from biologically relevant molecules. Photochem Photobiol 1999;70:391–475. doi: 10.1562/0031-8655(1999)070<0391:ACOSOY>2.3.CO;2
- Dzhagarov BM, Kruk NN, Konovalova NV, Solodunov AA, Stepuro II. Photosensitized formation of singlet oxygen by vitamins of the B group. J Appl Spectros 1995;62:285–9. doi: 10.1007/BF02606482
- Montaña MP, Pappano N, Debattista N, Ávila V, Posadaz A, Bertolotti SG, et al. The activity of 3- and 7-hydroxyflavones as scavengers of superoxide radical anion generated from photo-excited riboflavin. Can J Chem 2003;81:909–14. doi: 10.1139/v03-097
- Montaña MP, Massad WA, Criado S, Biasutti A, García NA. Stability of flavonoids in the presence of riboflavin-photogenerated reactive oxygen species. A kinetic and mechanistic study on quercetin, morin and rutin. Photochem Photobiol 2010;86:827–34. doi: 10.1111/j.1751-1097.2010.00754.x
- Agüero MB, González M, Lima B, Svetaz L, Sánchez L, Zacchino S, et al. Argentinean propolis from Zuccagnia punctata Cav. (Caesalpinieae) exudates: phytochemical characterization and antifungal activity. J Agric Food Chem 2010;58:194–201. doi: 10.1021/jf902991t
- Szliszka E, Czuba ZP, Domino M, Mazur B, Zydowicz G, Krol W. Ethanolic extract of propolis (EEP) enhances the apoptosis-inducing potential of TRAIL in cancer cells. Molecules 2009;14:738–54. doi: 10.3390/molecules14020738
- Kumazawa S, Hamasaka T, Nakayama T. Antioxidant activity of propolis of various geographic origins. Food Chem 2004;84:329–39. doi: 10.1016/S0308-8146(03)00216-4
- Lima B, Tapia AA, Luna L, Fabani MP, Schmeda-Hirschmann G, Podio NS, et al. Main flavonoids, DPPH activity, and metal content allow determination of the geographical origin of propolis from the Province of San Juan (Argentina). J Agric Food Chem 2009;57:2691–8. doi: 10.1021/jf803866t
- Ahn MR, Kumazawa S, Usui Y, Nakamura J, Matsuka M, Zhu F, et al. Antioxidant activity and constituents of propolis collected in various areas of China. Food Chem 2007;101:1383–92. doi: 10.1016/j.foodchem.2006.03.045
- Tratniek PG, Hoigné J. Oxidation of substituted phenols in the environment: a QSAR analysis of rate constants for reaction with singlet oxygen. Environ Sci Technol 1991;25:1596–1604. doi: 10.1021/es00021a011
- Criado S, Allevi C, Ceballos C, García NA. Visible-light-promoted degradation of the commercial antioxidants butylated hydroxyanisole (BHA) and butylated hydroxytoluene (BHT): a kinetic study. Redox Rep 2007;12:282–8. doi: 10.1179/135100007X239252
- Wilkinson F, Helman WP, Ross A. Rate constants for the decay of the lowest electronically excited singlet state of molecular oxygen in solution. An expanded and revised compilation. J Phys Chem Ref Data 1995;24:663–1021. doi: 10.1063/1.555965
- Amat-Guerri F, García NA. Photodegradation of hydroxylated N-heteroaromatic derivatives in natural-like aquatic environments. A review of kinetic data of pesticide model compounds. Chemosphere 2005;59:1067–82. doi: 10.1016/j.chemosphere.2004.12.028
- Fritz BJ, Matsui K, Kasai S, Yoshimura A. Triplet lifetime of some flavins. Photochem Photobiol 1987;45:539–41. doi: 10.1111/j.1751-1097.1987.tb05415.x
- Gutiérrez S, Criado S, Bertolotti S, García NA. Dark and photoinduced interactions between Trolox, a polar-solvent-soluble model for vitamin E, and riboflavin. J Photochem Photobiol B 2001;62:133–9. doi: 10.1016/S1011-1344(01)00170-1
- Wilkinson F, Helman WP, Ross AB. Quantum yields for the photosensitized formation of the lowest electronically excited singlet state of molecular oxygen in solution. J Phys Chem Ref Data 1993;22:133–262. doi: 10.1063/1.555934
- García NA. Singlet molecular oxygen-mediated photodegradation of aquatic phenolic pollulants. A kinetic and mechanistic overview. J Photochem Photobiol B 1994;22:185–96. doi: 10.1016/1011-1344(93)06932-S
- Escalada JP, Pajares A, Gianotti J, Massad W, Bertolotti S, Amat-Guerri F, et al. Dye-sensitized photodegradation of the fungicide carbendazim and related benzimidazoles. Chemosphere 2006;65:237–44. doi: 10.1016/j.chemosphere.2006.02.057
- Silva E, Herrera L, Edwards AM, De La Fuente J, Lissi E. Enhancement of riboflavin-mediated photo-oxidation of glucose 6-phosphate dehydrogenase by uronic acid. Photochem Photobiol 2005;81:206–11. doi: 10.1562/2004-07-14-RA-233.1
- Silva E, Edwards AM, Pacheco D. Visible light-induced photooxidation of glucose sensitized by riboflavin. J Nutr Biochem 1999;10:181–5. doi: 10.1016/S0955-2863(98)00093-X
- Garcia J, Silva E. Flavin-sensitized photooxidation of amino acids present in a parenteral nutrition infusate: protection by ascorbic acid. J Nutr Biochem 1997;8:341–5. doi: 10.1016/S0955-2863(97)00024-7
- Posadaz A, Biasutti A, Casale C, Sanz J, Amat-Guerriand F, García NA. Rose bengal-sensitized photooxidation of the dipeptides Trp-Phe, Trp-Tyr and Trp-Trp. Kinetics, mechanism and photoproducts. Photochem Photobiol 2004;80:132–8. doi: 10.1562/2004-03-03-RA-097.1
- Montaña P, Pappano N, Debattista N, Ávila V, Posadaz A, Bertolotti SG, et al. The activity of 3- and 7-hydroxyflavones as scavengers of superoxide radical anion generated from photo-excited riboflavin. Can J Chem 2003;81:909–14. doi: 10.1139/v03-097
- Spikes JD, Shen HR, Kopeccková P, Kopecek J. Photodynamic crosslinking of proteins. III. Kinetics of the FMN- and rose bengal-sensitized photooxidation and intermolecular crosslinking of model tyrosine-containing N-(2-Hydroxypropyl) methacrylamide copolymers. Photochem Photobiol 1999;70:130–7. doi: 10.1111/j.1751-1097.1999.tb07980.x