Abstract
Excessive free iron in blood and in organ tissues (so called iron overload) has been observed in degenerative diseases such as atherosclerosis, cancer, neurological, and certain autoimmune diseases, in which fibrin-like deposits are also found. Although most of the body iron is bound to hemoglobin and myoglobin in a divalent ferrous form, a certain amount of iron exists in blood as a trivalent (ferric) ion. This particular chemical state of iron has been shown to be toxic to the human body when not controlled by endogenous and/or dietary chelating agents. Experiments described in this paper show for the first time that ferric ions (Fe3+) can generate hydroxyl radicals without participation of any redox agent, thus making it a special case of the Fenton reaction. Ferric chloride was also demonstrated to induce aggregation of purified fibrinogen at the same molar concentrations that were used for the generation of hydroxyl radicals. Iron-aggregated fibrinogen, by contrast to native molecule, could not be dissociated into polypeptide subunit chains as shown in a polyacrylamide gel electrophoresis. The mechanism of this phenomenon is very likely based on hydroxyl radical-induced modification of fibrinogen tertiary structure with the formation of insoluble aggregates resistant to enzymatic and chemical degradations. Soluble modified fibrinogen species can be determined in blood of thrombotic patients by the reaction with protamine sulfate and/or by scanning electron microscopy. In view of these findings, it is postulated that iron-induced alterations in fibrinogen structure is involved in pathogenesis of certain degenerative diseases associated with iron overload and persistent thrombosis. It is concluded that the detection of hydroxyl radical-modified fibrinogen may be utilized as a marker of a thrombotic condition in human subjects.
Introduction
Iron is a transition metal that plays an essential role in many physiological functions in living organisms. Iron absorption from the diet occurs mainly in the duodenum by a tightly regulated process. Metabolism of iron in the human body is well controlled, so no large quantities of this metal are released at a given time. However, certain proportion of iron exists in blood in a free form in the so called ‘labile pool’.Citation1 Current understanding of the role of iron in pathologic conditions suggests its involvement in the in vivo production of oxygen-centered free radicals.Citation2–Citation5 An abundant source of redox active iron is heme, which is released from oxidized hemoglobin.Citation6 Heme oxygenase opens the porphyrin ring producing biliverdin, carbon monoxide and redox-active iron, concentration of which in blood is regulated by ferritin and hepcidin.Citation7
There is ample evidence that iron-induced reactive oxygen species are involved in the pathogenesis of numerous degenerative disorders.Citation8–Citation12 Specifically, a linkage has been shown to exist between iron body stores and cardiovascular risk factors.Citation13–Citation15 Little is known, however, about the mechanism by which iron overload contributes to these diseases that are generally believed to be associated with the activation of blood coagulation and intravascular deposition of fibrin.
Under physiological conditions fibrin generated at the site of vessel wall injury is gradually, albeit completely removed by the fibrinolytic enzyme system to give place for growth of the connective tissue. In addition to thrombin physicochemical properties of fibrinogen can be modified by certain chemical agents and free radicals. For example, exposure of purified fibrinogen to ascorbic acid/cupric ion system results in the formation of an insoluble fibrin-like precipitate.Citation16 However, it was recently discovered in this laboratory that soluble human fibrinogen can be converted into an insoluble polymer by the action of ferric ions without any additional redox agents. Moreover, it was also found out that iron-modified fibrinogen is remarkably resistant to enzymatic and chemical degradations. These findings may explain why iron overload is closely associated with numerous degenerative diseases in which insoluble fibrin-like deposits cause permanent inflammation and thrombosis.
Methods
Measurement of hydroxyl radicals
A stock solution of 1 mM coumarin-3-carboxylic acid (Sigma-Aldrich) was diluted 1:2 with PBS, pH 7.4, and stored at room temperature before the use.Citation17 Fifty microliters aliquots of ferric chloride or ferrous sulfate solutions at various molar concentrations were added to black plastic microplate wells containing each 100 μl of the coumarin reagent. After 2-min incubation at RT fluorescence intensity (impulses/second) of the hydroxylated product of coumarin was measured for one second in PerkinElmer 1420 Multilabel Counter at 350/450 nm of excitation/emission wavelength.
Fibrinogen aggregation by iron
Commercial preparation of human fibrinogen (Sigma-Aldrich, 96% clottable) was dialyzed against PBS, pH 7.4, to remove sodium citrate present in this product, and a stock solution (20 mg/ml) was stored at −20°C. Just before use, a working solution was prepared by diluting fibrinogen with PBS to 2 mg/ml and kept at room temperature. Aliquots (100 μl) of ferric chloride or ferrous sulfate at various molar concentrations were added to a polystyrene round bottom microplate wells each containing 50 μl of fibrinogen solution. Fibrinogen precipitated in the presence of iron was measured by recording optical density values at 680 nm in the ELISA reader within two minutes after the addition of reagents against blanks containing fibrinogen and/or iron in PBS buffer.
Polyacrylamide gel electrophoresis
A solution of human dialyzed fibrinogen (2 mg/ml) was divided into two portions. One, serving as a control, was stored at room temperature, and the other one was incubated with ferric chloride (10 μM) for 3 hours. The precipitated polymer formed in the iron-treated sample was washed with PBS buffer. Afterwards both treated and untreated samples of fibrinogen were mixed with a buffer containing beta-mercaptoethanol, boiled for 3 minutes and subjected to SDS-polyacrylamide gel (10%) electrophoresis. After extensive washing of the gel, protein bands were visualized by staining with Coumassi Brilliant Blue. It should be noted that iron-treated fibrinogen polymer did not dissolve upon boiling in reducing buffer, but formed a semi-solid gel.
Scanning electron microscopy (SEM)
Blood was obtained from six healthy control subjects (3 males and 3 females ranging between 20 and 45 years) [Ethical clearance from the University of Pretoria Ethics committee]. These individuals did not have any chronic conditions, did not smoke and did not use any medication. Their ferritin (showing the body’s total iron storage capacity) and transferrin (a plasma protein that transports iron) were within the normal ranges being 10–120 ng/ml and 2.5–3.8 g/l respectively. The micrographs from the healthy individuals were compared to our data base of thousands of micrographs, and found to be comparable. Furthermore, we used whole blood from 12 thromboembolic stroke patients with ages ranging form 40 to 60 years. These patients’ micrographs compare well to our thromboembolic ischemic stroke micrograph database consisting of over 80 patients.
All samples for SEM were prepared using 10 μl aliquots of blood placed directly on 0.2 nm Isopore Membrane Filter. In experiments with iron two microliters of 15 mM ferric chloride solution was mixed with whole blood on a membrane. Before further processing the membranes were incubated at room temperature for 5 minutes and then were immersed in 0.075M sodium phosphate buffer, pH 7.4, and finally placed on a shaker for 20 minutes. The samples were then fixed in a 2.5% formaldehyde/glutaraldehyde and viewed with a ZEISS FEG scanning electron microscope.
Results
In the course of experiments on modification of human fibrinogen with hydroxyl radicals, it was found out that this type of radical can be generated in aqueous solutions just in the presence ferric chloride without any redox agent. As can be seen from only Fe3+ but not Fe2+, generated hydroxyl radicals with maximum intensity at 9 μM ferric chloride. This is the first experimental demonstration that trivalent iron alone, without any additional reagent, can generate powerful hydroxyl radicals that, in turn, cause dramatic modifications of fibrinogen properties. The results of experiments presented in show that human fibrinogen was polymerized only in the presence of trivalent iron (ferric chloride), as recorded by increased OD values, and not by the divalent iron ions (ferrous sulfate). Maximum aggregation was reached at 9 μM concentration of ferric chloride, and no aggregation was observed with ferrous sulfate up to 11 μM concentration.
Figure 1. Effect of trivalent iron (ferric chloride) and divalent iron (ferrous sulfate) on the generation of hydroxyl radicals measured as fluorescence intensity of hydroxy-derivative of coumarin.
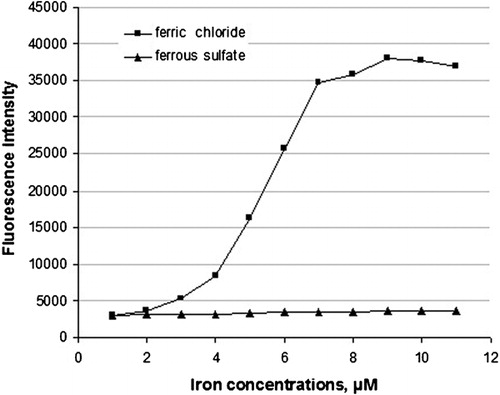
Figure 2. Conversion of soluble fibrinogen into insoluble aggregate, induced with iron and monitored as optical density OD values.
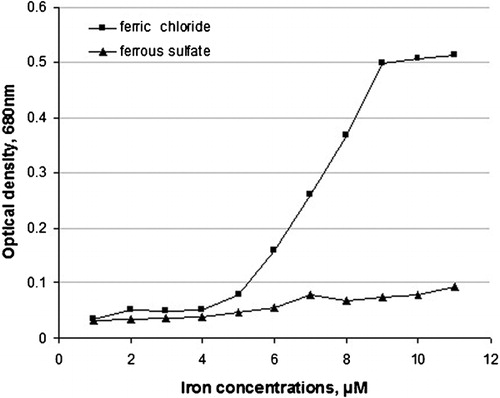
Another novel observation described in this paper is that iron-modified fibrinogen could not be dissociated into subunit polypeptide chains under the reducing conditions of electrophoresis (). This result indicates that the iron-induced modification of physicochemical properties of fibrinogen is not due to a simple disulfide rearrangement, but to more profound alterations of tertiary structure of the polypeptide chains. In fact, modified fibrinogen not only could not be dissociated into its subunit chains, but also was insoluble to the concentrated chaotropic agent (SDS).
Figure 3. SDS-polyacrylamide gel electrophoresis of native fibrinogen unreduced – line 1; reduced – line 2; and of iron-modified fibrinogen unreduced – line 3; reduced – line 4).
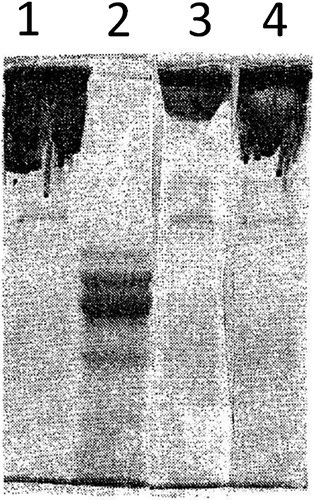
As recently shown by means of SEM blood of people with thrombotic tendency contain dense fibrin-like deposits.Citation18 Here we demonstrate that such deposits are also present in patients with stroke () and are strikingly similar to those produced with ferric chloride when added to plasma obtained from healthy individuals (). It should be noted that fibrin-like deposits are also formed after the addition of divalent iron ions to normal blood. This seemingly controversial result is explained in terms of rapid oxidation of Fe2+ to Fe+3 by ferroxidase associated with blood ferritin.Citation19
Discussion
Metabolism of iron in the human body is well controlled so no large quantities of Fe3+ are released at a given time. The level of free iron in the body fluids of mammals is regulated by transferrin family of proteins, which binds ferric ions with high affinity.Citation20 The release of redox-active iron and heme into the blood-stream is toxic to the vasculature, contributing to the development of thrombosis. It was demonstrated that ferric chloride treatment of isolated mouse aorta perfused with whole blood, was associated with endothelial denudation, collagen exposure, and occlusive thrombus formation.Citation21 This form of iron also potentiated venous and arterial thrombosis induced by bacterial lipopolysaccharide that may explain pathologic complications of infections.Citation22 In addition to free iron free hemoglobin may also be biologically hazardous, because of the potential to catalyze hydroxyl-radical generation in areas of inflammation.Citation23–Citation25 Moreover, chronic hemolysis is known to lead to thrombosis and vascular complications.Citation26–Citation28
There is plethora of data indicating the relationship between body iron overload and pathogenesis of numerous degenerative diseases such as atherosclerosis, Parkinson’s and Alzheimer’s diseases, multiple sclerosis, age-related macular degeneration, prion disease and other neurological conditions.Citation29–Citation35 It is noteworthy that these degenerative pathologies can be prevented by natural products and synthetic substances that act as free radical scavengers and iron chelators.Citation36–Citation39
It should be noted that fibrin-like material, often ascribed to thrombosis, is present in practically all the degenerative diseases associated with iron overload. Classically, fibrin is produced from fibrinogen as a result the activation of blood coagulation cascade. Fibrinogen is a protein of a molecular weight 340 kDa composed of three pairs of polypeptide chains joined by 32 disulfide bridges, and is present in normal human plasma at a concentration of 250–350 mg/dl. Fibrinogen molecules, when acted upon with enzyme thrombin, are converted into fibrin monomers followed by their linear and lateral aggregation to form fibrin clot. Under normal physiological conditions fibrin clots are then completely degraded by the fibrinolytic enzyme system to give room for connective tissue and for proper healing of the wound. However, in pathologic circumstances of the degenerative diseases, thrombus/fibrin deposits remain intact even after thrombolytic therapy.
Although it is generally believed that thrombosis is caused by excessive fibrin formation, it is now being recognized that equally important, if not essential, is the rate of fibrin clot removal. In connection with this a variety of fibrinolytic agents has been designed for acute thrombosis such as myocardial infarctions and strokes. Yet their effectiveness is limited by the timing of the installment of the thrombolytic therapy, the window of opportunity being only 3–4 hours after thrombosis onset.Citation40 It is now being understood that the relative ineffectiveness of thrombolytic therapies is not due to their failure to activate fibrinolysis, but to the unusual resistance of fibrin to proteolytic degradation.Citation41 Although modification of fibrin(ogen) structure by hydroxyl free radicals was suggested to be the culprit, no cause of their excessive production was identified. However, positive results obtained with chelation therapy following hemorrhagic stroke, indicate the important role of iron in this debilitating disease.Citation42
As shown in , ferric (Fe3+), but not ferrous (Fe2+) ions reacted readily with human fibrinogen in solution causing its transformation into an insoluble aggregate. In view of the fact that ferric ions generate highly reactive hydroxyl radicals (), it is very likely that this type of free radicals is also responsible for structural modifications of fibrinogen in vivo. Although the interaction of iron with fibrinogen simply mimics blood clotting, yet it has profound pathologic consequences. The most important one is that the iron-modified fibrinogen does not elicit spontaneous fibrinolysis, as does regular fibrin clot. This fact may explain why ferric chloride induces in vivo thrombus-like arterial obstructions that are not spontaneously degradable.Citation22, Citation43 Deposition of glomerular fibrin associated with inhibition of fibrinolysis in rabbits treated with bacterial lipopolysaccharideCitation44 may also be explained in terms of the release of free iron from the arterial endothelial cells.Citation45
In addition, it should be noted that previous experiments with transition metals and fibrinogen were done in the presence of ascorbic acid. However, in the experiments described in this paper no redox substance was used whatsoever. Hence, the only explanation for the formation of hydroxyl radicals in an aqueous solution is the reaction of ferric ion with hydroxyl group of ionized water according to the following reaction:
where an electron from the hydroxyl group of water is transferred to iron with the formation of a hydroxyl radical and a ferrous ion. Thus, this novel observation can be considered as a special case of the Fenton reaction.
Whatever the mechanism, the fibrin-like polymer generated by hydroxyl radicals is remarkably resistant to proteolytic degradation. This polymer is also refractory to decomposition by strong reducing agents that normally dissociates fibrinogen into its subunit polypeptide chains (). Since practically all proteins composed of disulfide-linked subunits can be dissociated under the reducing conditions, the unusual resistance of Fe-induced fibrinogen polymer suggests its special structure. Tertiary structure of blood proteins is such that it prevents undesirable aggregation by holding their hydrophobic groups inside the interior of protein molecules that are stabilized by intra-molecular disulfide bonds.Citation46 Once disulfide bonds are broken the polypeptide chains become unfolded with the exposure of hydrophobic domains, which then interact between each other to form insoluble aggregates.Citation47 It is of great importance to note that such aggregates cannot be degraded by reducing agents or by proteolytic enzymes. Consequently, it is often very difficult to identify fibrin-like deposits in pathologically affected organs in various chronic diseases, because no soluble antigen can be released into the supernatants of the extracted tissues. In the case of Alzheimer’s brain tissue fibrinogen antigen was detected only under extreme conditions of high proteolytic activity.Citation48 In concordance with this, the presence of insoluble fibrin(ogen) deposits can only be demonstrated by a direct immunochemical staining of the tissue sections.Citation49–Citation51
However, before HR-modified fibrinogen becomes an insoluble precipitate, it forms a soluble complex with native fibrinogen similarly to the previously described soluble fibrin monomer complexes (SFMC).Citation51 Due to the increased hydrophobicity of such complexes they are readily precipitable with basic proteins such as protamine sulfateCitation52 and this reaction was utilized to determine the level of SFMC in patients with heart attacks.Citation53 The notion that SFMC are generated in vivo with thrombin was later on challenged by experiments in which PS precipitable proteins was shown to be generated in vitro by the incubation of the anticoagulated whole blood under anoxic conditions.Citation54 Therefore, all the reactions described previously with the use of protamine sulfate are applicable to the hydroxyl radical-modified fibrinogen complexes. This is due to the fact that positive charges enhance hydrophobic interactionsCitation55 leading eventually to the loss of protein solubility. Diabetes mellitus is another case in which there is an increased concentration of modified fibrinogen present in blood in the form a macromolecular complex resistant to fibrinolytic degradation.Citation56 Similar type of a plasma complex, termed Advanced Oxidation Protein Products was found to be significantly increased in uremic patients.Citation57 It was shown by means of SEM that plasma of stroke patients contains dense fibrin-like depositsCitation18 similar to those induced with iron in whole blood.Citation58 Such deposits may explain reduced clot permeability and susceptibility to fibrinolysis observed in patients with heart diseases.Citation59
In view of the accumulating data showing the importance of iron overload in degenerative diseases, it is possible that insufficient chelation of iron may also be responsible for the formation of fibrin-like deposits in such diseases. Thus, a balance between free iron and the chelation capacity of blood may also be considered as a risk factor for thrombosis. An important class of dietary chelating substances includes polyphenols that can also directly scavenge hydroxyl radicals.Citation2 The known role of fruit and vegetable diet in the prevention of cardiovascular disease may be due to their content of polyphenolic substances.Citation60,Citation61 These natural phytochemicals, may provide protection against many chronic diseases not only by their free radical scavenging properties, but also by chelation of iron.62–67
In conclusion, it is described for the first time in this paper that ferric ions can generate hydroxyl radicals under physiological conditions without any redox agent, thus constituting a special case of the Fenton reaction. It should be emphasized that the single event of the transfer of one electron from ferric ion to the hydroxyl group of water has such profound pathologic consequences. The most biologically active hydroxyl radicals generated as a result of this reaction, attack fibrinogen molecules converting them to insoluble fibrin-like deposits. By contrast to thrombin-induced fibrin clots, hydroxyl radical-generated polymer is remarkably resistant to enzymatic and chemical degradations. Consequently, it is practically impossible to identify this type of deposits in blood and/or tissue of patients with thrombotic and degenerative diseases by conventional methods. However, the intermediate soluble complexes of modified fibrinogen can be determined utilizing their property to form insoluble precipitates with basic proteins. In addition, and more importantly, dense fibrin-like deposits identified in a drop of blood by means of SEM offer a useful determination of a marker of thrombosis.
References
- Kruszewski M. Labile iron pool: the main determinant of cellular response to oxidative stress. Mutat Res. 2003;531:81–92.
- Lipinski B. Hydroxyl radical and its scavengers in health and disease. Oxid Med Cell Longev. 2011;2011:809696.
- Lee DW, Andersen JK. Iron elevation in the aging Parkinsonian brain: a consequence of impaired iron homeostasis? J Neurochem. 2010;112:332–9.
- Thomas C, Mackey MM, Diaz AA, Cox DP. Hydroxyl radical is produced via the Fenton reaction in submitochondrial particles under oxidative stress: implications for diseases associated with iron accumulation. Redox Rep. 2009;14:102–8.
- Weinberg EB. The hazards of iron loading. Metallomics. 2010;2:732–40.
- Reeder BJ, Svistunenko DA, Cooper CE, Wilson MT. The radical and redox chemistry of myoglobin and hemoglobin: from in vitro studies to human pathology. Antioxid Redox Signal. 2004;6:954–66.
- Balla J, Vercellotti GM, Jeney V, Yachie A, Varga Z, Jacob HS, et al.. Heme, heme oxygenase, and ferritin: how the vascular endothelium survives and dies in an iron-rich environment. Antioxid Redox Signal. 2007;9:2119–37.
- Andersen JK. Iron elevations in the aging Parkinsonian brain: a consequence of impaired iron homeostasis? J Neurochem. 2010;112:332–9.
- Ahluwalia N, Genoux A, Ferrieres J, Perret B, Carayol M, Drouet L, et al.. Iron status is associated with carotid atherosclerotic plaques in middle-aged adults. J Nutr. 2010;140:812–6.
- Brissot P, Ropert M, Lan LeC, Loreal O. Nontransferrin bound iron: A key role in iron overload and iron toxicity. Biochim Biophys Acta. 2012;1820:403–10.
- Kell DB. Towards a unifying, system biology understanding of large-scale cellular death and destruction caused by poorly liganded iron: Parkinson’s, Huntington’s, Alzheimer’s, prions, bactercides, chemical toxicology and others as example. Arch Toxicol. 2010;84:825–89.
- Yuan XM, Li W. The iron hypothesis of atherosclerosis and its clinical impact. Ann Med. 2003;35:578–91.
- Aigner E, Aigner E, Hinz C, Steiner K, Rossmann B, Pfleger J, et al.. Iron stores, liver transaminase levels and metabolic risk in healthy teenagers. Eur J Clin Invest. 2010;40:155–63.
- Franchini M, Targher G, Mntagnana M, Lippi G. Iron and thrombosis. Ann Hematol. 2008;87:167–73.
- Kruszewski M. The role of labile iron pool in cardiovascular diseases. Acta Biochim Pol. 2004;51:471–80.
- Marx G, Chevion M. Fibrinogen coagulation without thrombin: reaction with vitamin C and copperII. Thromb Res. 1985;40:11–8.
- Manevich Y, Held KD, Biaglow JE. Coumarin-3-carboxylic acid as a detector for hydroxyl radicals generated chemically and by gamma radiation. Radiat Res. 1997;148:580–91.
- Pretorius E, Oberholzer HM, van der Spuy WJ, Meiring JH. Age-related changes in fibrin networks and platelets of individuals over 75: a scanning electron microscopy study showing ‘thrombotic preparedness’. J Thromb Thrombolysis. 2010;29:271–5.
- Yang X, Chasteen ND. Ferroxidase activity of ferritin: effects of pH, buffer and Fe(II) and Fe(III) concentrations on Fe(II) autoxidation and ferroxidation. Biochem J. 1999;338:615–8.
- Alayash AI, Patel RP, Cashon RE. Redox reactions of hemoglobin and myoglobin: biological and toxicological implications. Antioxid Redox Sign. 2001;3:313–27.
- Woollar KJ, Sturgeon S, Chin-Dusting JP, Salem HH, Jackson SP. Erythrocyte hemolysis and hemoglobin oxidation promote ferric chloride-induced vascular injury. J Biol Chem. 2009;284:13110–8.
- Wang X, Xu L. An optimized murine model of ferric chloride-induced arterial thrombosis for thrombosis research. Thromb Res. 2005;115:95–100.
- Alayash AI, Patel RP, Cashon RE. Redox reaction of hemoglobin and myoglobin: biological and toxicological implications. Antioxid Redox Sign. 2001;3:313–27.
- Puppo A, Halliwell B. Formation of hydroxyl radicals from hydrogen peroxide in the presence of iron. Is hemoglobin a biological Fenton reagent? Biochem J. 1988;249:185–90.
- Dyke VanBR, Saltman P. Hemoglobin: a mechanism of the generation of hydroxyl radicals. Free Radic Biol Med. 1996;20:985–9.
- Belcher JD, Beckman JD, Balla G, Balla J, Vercellotti G. Heme degradation and vascular injury. Antioxid Redox Signal. 2010;12:233–48.
- Kato GJ, Taylor JG. Pleiotropic effects of intravascular haemolysis on vascular homeostasis. Br J Haematol. 2010;148:690–701.
- Rother RP, Bell L, Hillmen P, Gladwin MT. The clinical sequelae of intravascular hemolysis and extracellular plasma hemoglobin: a novel mechanism of human disease. JAMA. 2006;293:1653–62.
- Shah SV, Rajapurkar MM. The role of labile iron in kidney diseases and treatment with chelation. Hemoglobin. 2009;33:378–85.
- Jomova K, Vondrakova D, Lawson M, Valko M. Metals, oxidative stress and neurodegenerative disorders. Mol Cell Biochem. 2010;345:91–104.
- Salonen JT, Nyyssonen K, Korpela H, Tuomilehto J, Seppanen R, Salonen R. High stored iron levels are associated with excess risk of myocardial infarction in eastern Finnish men. Circulation. 1992;86:803–11.
- Nagy E, Eaton JW, Jeney V, Soares MP, Varga Z, Galajda Z, et al.. Red cells, hemoglobin, heme, iron, and atherogenesis. Arterioscler Thromb Vasc Biol. 2010;30:1347–53.
- Brewer GJ. Risk of copper and iron toxicity during aging in humans. Chem Res Toxicol. 2010;23:319–26.
- Muckenthaler MU. Iron toxicity in diseases of aging: Alzheimer’s disease, Parkinson’s disease and atherosclerosis. J Alzheimers Dis. 2009;16:879–95.
- Morkedal B, Laugsand LE, Romundstad PR, Vatten LJ. Mortality from ischaemic heart disease: sex-specific effects of transferrin saturation, serum iron, and total iron binding capacity. The HUNT study. Eur J Cardiovasc Prev Rehabil. 2011;18:687–94.
- Prior RL, Wu X. Anthocyanins: structural characteristics that result in unique metabolic patterns and biological activities. Free Radic Res. 2006;40:1014–28.
- Ferrara DE, Taylor WR. Iron chelation and vascular function. In search of the mechanisms. Arterioscler Thromb Vasc Biol. 2005;25:2235–40.
- Matthews AJ, Vercellotti GM, Menchaca HJ, Bloch PH, Michalek VN, Marker PH, et al.. Iron and atherosclerosis: inhibition by the iron chelator deferiprone L(1). J Surg Res. 1997;73:35–40.
- Capellini MD, Taher A. Deferasirox (Exjade) for the treatment of iron overload. Acata Hematol. 2009;122:165–72.
- Leys D, Deplanque D. Thrombolysis beyond the three-hour time window. Clin Exp Hypertens. 2006;28:313–6.
- Lipinski B. Modification of fibrin structure as a possible cause of thrombolytic resistance. J Thromb Thrombolysis. 2010;29:296–8.
- Robinson SR, Dang TN, Dringen R, Bishop GM. Hemin toxicity: a preventable source of brain damage following hemorrhagic stroke. Redox Rep. 2009;14:228–35.
- Eckly A, Hechler B, Freund M, Zerr M, Cazevane JP, Lanza F, et al.. Mechanism underlying Fe(Cl3)-induced arterial thrombosis. J Thromb Haemost. 2011;9:779–89.
- Lipinski B, Nowak A, Gurewich V. The organ distribution of I-125-fibrin in the generalized Shwartzman reaction and its relation to leukocytes. Br J Haematol. 1974;28:221–31.
- Li L, Frei B. Prolonged exposure to lipopolysaccharide increases iron, heme, and p22 levels and NADPH oxidase activity in human aortic endothelial cells: inhibition by desferrioxamine. Arterioscl Thromb Vasc Biol. 2009;29:732–8.
- Lins L, Brausseur R. The hydrophobic effect in protein folding. FASEB J. 1995;9:535–40.
- van Oss CJ. Long-range and short-range mechanisms of hydrophobic attraction and hydrophilic repulsion in specific and aspecific interactions. J Mol Recognit. 2003;16:177–90.
- Lipinski B, Sajdel-Sulkowska EM. New insight into Alzheimer’s disease: demonstration of (fibrinogen)-serum albumin insoluble deposits in brain tissue. Alzheimer Dis Assoc Disord. 2006;20:324–6.
- Kim YM, Chung SI, Lee SY. Roles of plasma proteins in the formation of silicotic nodules in rats. Toxicol Lett. 2005;158:1–9.
- Wasiutynski A, Wiczynski GM, Morton M. Fibrinogen/fibrin and albumin colocalize on the surface of placental villi a novel element of feto-maternal barrier. Ann Diagn Paediatr Pathol. 2002;6:125–9.
- Lipinski B, Wegrzynowicz Z, Budzynski AZ, Kopec M, Latallo ZS, Kowalski E. Soluble unclottable complexes formed in the presence of fibrinogen degradation products (FDP) during the fibrinogen-fibrin conversion and their potential significance in pathology. Thromb Diath Haemorrh. 1967;17:65–9.
- Lipinski B, Worowski K. Detection of soluble fibrin monomer complexes in blood by means of protamine sulphate test. Thromb Diathes Haemorrh. 1968;20:44–9
- Lipinski NowakB, Drzewiecki J. Levels of soluble fibrin monomer complexes level in the course of myocardial infarction. Br Heart J. 1972;34:593–6.
- Lipinski B, Gurewich V. Non-clottable, protamine sulfate precipitable protein of blood. Studies on its in vitro generation and incorporation into the fibrin clot. Thromb Res. 1972;1:569–72.
- Andrews BA, Schmidt AS, Asenjo JA. Correlation for the partition behavior of proteins in aqueous two-phase systems: Effects of surface hydrophobicity and charge. Biotechnol Bioeng. 2005;90:380–90.
- Lipinski B, Federman SM, Krolewski AS. Plasma macromolecular protein complex: Interaction with fibrin and fibrinolysis. Thromb Res. 1995;78:461–5.
- Witko-Sarsat V, Friedlander M. Capeillere-Blandidn C, Nguyen AT, Canteloup S, Dayer JM, et al. Advanced oxidation protein products as a novel marker of oxidative stress in uremia. Kidney Int. 1996;49:1304–13.
- Lipinski B, Pretorius E, Oberholzer HM, van der Spuy WJ. Interaction of fibrin with red blood cells: the role of iron. Ultrastruct Pathol. In press.
- Undas A, Szuldrzynski K, Stepien E, Zalewski J, Godlewski J, Tracz W, et al.. Reduced clot permeability and susceptibility to lysis in patients with acute coronary syndrome: effects of inflammation and oxidative stress. Athertosclerosis. 2008;196:551–7.
- Stoclet JC, Chataigneau T, Ndiaye TM, Oak MH, Bedoui EI, Chataigneau M, et al.. Vascular protection by dietary polyphenols. Eur J Pharmacol. 2004;500:299–313.
- Mladenka P, Zatloukalova L, Filipsky T, Hrdina R. Cardiovascular effects of flavonoids are not caused only by direct antioxidant activity. Free Radic Biol Med. 2010;49:963–75.
- Jomova K, Valko M. Importance of iron chelation in free radical-induced oxidative stress and human disease. Curr Pharm Des. 2011;17:3460–73.
- Philpott M, Lim CC, Ferguson LR. Dietary protection against free radicals: a case for multiple testing to establish structure-activity relationships for antioxidant potential of anthocyanic plant species. Int J Mol Sci. 2009;10:1081–110.
- Visavadiya NP, Soni B, Dalwadi N. Free radical scavenging and antiatherogenic activities of Sesamum indicum seed extracts in chemical and biological model systems. Food Chem Toxicol. 2009;47:2507–15.
- Yadav AS, Bhatnagar D. Free radical scavenging activity, metal chelation and antioxidant power of some of the Indian spices. Biofactors. 2007;31:219–27.
- Bengmark S, Mesa MD, Gil A. Plant-derived health: the effects of turmeric and curcuminoids. Nutr Hosp. 2009;24:273–81.