Abstract
P57kip2 is considered as a candidate tumor suppressor gene involvement in cell cycle control. In this study, we explored the expression of p57kip2 in various myelodysplastic syndrome (MDS) subsets by real-time quantitative PCR, as well as the relationship between p57kip2 and CXC receptor 4 (CXCR4). We also searched the role of stromal cell-derived factor-1 (SDF-1)/CXCR4 signal in p57kip2 expression in vitro. The expression of p57kip2 decreased in MDS cases (low grade MDS, P<0··001, n = 46; high grade MDS, P<0··001, n = 21), compared with that in control group. Patients with poor karyotype (according to IPSS) had lower p57kip2 than that in the normal group (P<0··05). P57kip2 expression increased after treatment with decitabine in the cases that had achieved response (P = 0··009, n = 7). Additionally, a positive correlation between p57kip2 and CXCR4 was investigated (r = 0··652, P<0··001, n = 67). P57kip2 expression in bone marrow mononuclear cells of normal controls increased significantly when co-cultured with SDF-1 in vitro, which could be blocked by AMD3100, whereas SDF-1 only induced a mild increase in p57kip2 in MDS. In conclusion, low expression of p57kip2 is common in MDS, which may play an important role in MDS pathogenesis. Reduced response to SDF-1 contributed to low expression of p57kip2 in MDS cases.
Keywords:
Introduction
Myelodysplastic syndrome (MDS) is considered as a primitive stem cell disorder, characterized by variable degrees of ineffective hematopoiesis and susceptibility to leukemic transformation.Citation1 In addition to cytogenetic aberrations, tumor suppressor gene inactivation may be involved in MDS pathogenesis. Cyclin-dependent kinase inhibitors, a large family of proteins that regulate cell cycle progression, cell proliferation and differentiation, have been shown widespread tumor suppression and deregulated in many types of human cancers.Citation2 Cyclin-dependent kinase inhibitors are divided into two families, the INK4 family and the KIP/CIP family. The latter consists of three members: p21CIP1, p27KIP1, and p57KIP2. Just recently, one study has reported that p21 expression is lower in MDS cases and related to apoptosis and disease progression.Citation3 So far, little is known about the role of p57kip2 in MDS pathogenesis.
The human p57kip2 gene resides in 11p15··5. Based on its function on cell cycle control, apoptosis, senescence, cell fate determination, cell migration and cytoskeletal organization,Citation2,Citation4 p57kip2 has been considered as a candidate tumor suppressor gene. The expression of p57kip2 is generally down regulated in multiple cancers through epigenetic changes, microRNA regulation, proteasomal degradation and signaling pathways alteration.Citation5–Citation7 Stromal cell-derived factor-1 (SDF-1) is an important alpha-chemokine that binds primarily to CXCR4, initiating several divergent signal pathways related to chemotaxis, cell survival and/or proliferation.Citation8 High concentrations of SDF-1 have been shown to maintain the hemopoietic stem cell (HSC) poolCitation9,Citation10 via p57kip2 expression which inhibit the cell cycle progression of HSC.Citation7 So p57kip2 is one of the direct downstream targets of SDF-1/CXCR4 signaling. Previous evidence suggests that CXCL12/CXCR4 axis, which is involved in CD34+ cells migration and trafficking of Tregs, is seriously impaired in MDS patients.Citation11,Citation12 Meanwhile, our previous studyCitation13 has found that altered CXCL12/CXCR4 axis in MDS was related to different apoptosis rate of CD34+ cells between low grade MDS and high grade MDS.
Therefore, in the present study, we hypothesized that impaired CXCL12/CXCR4 axis in the BM of MDS may affect p57kip2 expression which plays a role in pathogenesis of MDS. We measured p57kip2 and CXCR4 expression by real-time quantitative PCR (qPCR) in bone marrow mononuclear cells (BMNCs) from MDS patients and observed the changes of p57kip2 after treatment. Additionally, we treated BMNCs from healthy volunteers or MDS patients with different concentrations of SDF-1 respectively, to observe the effect of SDF-1/CXCR4 signal on p57kip2 expression.
Methods
Patients
This is a retrospective study. A total number of 67 de novo MDS cases, 10 AML transformed from MDS (MDS-AML) cases and nine de novo AML cases were included in this study. They were recruited between January 2009 and January 2011. MDS was diagnosed in accordance with the minimum diagnostic criteria established by the conference on MDS.Citation14 All the samples were obtained at diagnosis. The classification and prognostic risk scoring of MDS was performed according to the WHO criteriaCitation15 and the International Prognostic Scoring System (IPSS).Citation16 AML was classified according to the FAB criteria.Citation17 MDS patients were divided into two groups according to IPSS: low grade MDS (IPSS score ⩽1··0) and high grade MDS (IPSS score ⩾1··5). Eighteen sex and age-matched healthy volunteers without acute or chronic disease (tumor, inflammation, autoimmune diseases, etc.) were used as controls (median age 47 years, age range 22–71 years). Additionally, 22 MDS cases were followed up after three courses of decitabine (DAC) treatment (DAC 20 mg/m2 intravenously over 1 hour daily for 5 days every 4 weeks). NCCN clinical practice Guidelines 2011 in myelodysplastic syndromes is used in initial planning therapeutic options.Citation18 In addition, the patient’s preference is a critical determinant. At the time that BM was obtained after treatment, the clinical response was evaluated respectively by modified International Working Group response criteria.Citation19 In our study, the response group was defined as patients achieving a complete remission (CR) or partial remission (PR), or marrow CR (mCR). The ineffective group was defined as patients with disease progression or failure. The research was approved by the Ethics Committee of the Sixth Hospital affiliated with Shanghai Jiao Tong University, and informed consent was obtained for the patients/controls in accordance with the Declaration of Helsinki. Their clinical and hematological features are summarized in .
Table 1. Clinical features of MDS patients
BMNCs cultures
BMNCs were separated by centrifugation over a Ficoll-hypaque gradient (LymphoprepTM, Oslo, Norway). Fresh BMNCs were cultured in RPMI-1640 medium containing 10% FCS (Gibico, New York, USA) supplemented with 2 mM glutamine, 100 units/ml penicillin and 0··1 mg/ml streptomycin, with or without exogenous SDF-1 (5, 50, 500 ng/ml), AMD3100 (1 μg/ml) at 37°C in a humidified atmosphere with 5% CO2 for 24 hours.
qPCR
Total RNA extraction from 1 ml bone marrow mononuclear cells was performed with RNeazy Mini Kit (Qiagen, Hilden, Germany), according to the manufacturer’s instructions. With aid of the RevertAidTM First Strand cDNA Synthesis Kit (Fermentas, Burlington, Canada), cDNA was synthesized with random priming from 10 μl of total RNA, according to the manufacturer’s instructions. For the PCR, 1 μl of cDNA solution was mixed with 5 μl of RealMasterMix (Takara, Dalian, China), 0··2 μl for each of Sense primers and Antisense primers, and 3··6 μl of RNase-free water for a total volume of 10 μl. The PCR was performed in a fluorescent quantitation PCR cycler (LightCycler, Roche, Basel, Switzerland) with the primers used for the amplification of p57kip2, CXCR4, and GAPDH. The primer sequences for the three genes were used as described previously.Citation13,Citation20 The amplification consisted of denaturation at 95°C for 10 seconds, annealing at 58°C (p57kip2) or 62°C (CXCR4) for 20 seconds, and extension at 72°C for 10 seconds (45 cycles). Reactions were performed with appropriate negative control subjects (cDNA were replaced by DEPC-water). The threshold cycle (Ct) was subsequently determined. Relative quantification of marker gene mRNA expression was calculated with the comparative Ct method. The relative quantification value of target, normalized to an endogenous control (GAPDH gene) and relative to a calibrator, was expressed as 2−ΔΔCt (fold difference), where ΔCt = Ct of target genes−Ct of endogenous control gene (GAPDH) and ΔΔCt = ΔCt of samples for target gene−ΔCt of calibrator for the target gene. Two replicas were run for each sample.
Flow cytometry assay
For immunodetection of CD34 or CXCR4 on MDS cells, a whole-blood lysis method was used with directly labeled primary antibodies following the manufacturer’s instructions (Becton–Dickinson, Franklin Lakes, NJ, USA). Briefly, about 5×105 BMNCs were used. For CD34 detection, 10 μl of anti-CD34-PerCP were used and for CXCR4 detection, 10 μl of anti-CXCR4-APC were used. A control sample was incubated with the appropriate isotype control antibodies. After incubation, cells were washed once and analyzed within 2 hours on a FACS Calibur (Becton–Dickinson). Flow cytometry data were analyzed by the FlowJo software (version 2.7.4; Tree Star, Ashland, OR, USA).
Statistical analysis
All statistical analyses were performed by the SPSS 17.0 System. Multiple comparisons were made by a one-way analysis of variance test. The significance between before-treatment group and after-treatment group was determined by Student’s t-test for paired samples. Spearman correlation analysis was used for ranking correlation tests. P<0.05 was considered statistically significant.
Results
Expression of p57kip2 in MDS, AML, MDS–AML and control group
The results of qPCR are detailed in . As shown in A, the expression level of p57kip2 was significantly reduced in low grade MDS [0.55±0.58, P<0.001 (n = 46)], high grade MDS [0.48±0.53, P<0.001 (n = 21)], and AML and MDS–AML group [0.46±0.56, P<0.001 (n = 19)] compared with that in control group. There was no significant difference among low grade MDS, high grade MDS and AML and MDS–AML group. In MDS, according to WHO subtypes, there was also no significant difference among them.
Figure 1. Expression of p57kip2 mRNA in MDS cases. *P<0.05; **P<0.01; NS, no significant statistical correlation. (A) The median value of p57kip2 expression is clearly lower in patients of low grade MDS (n = 46), high grade MDS (n = 21), and AML and MDS–AML (n = 19), compared with that in control group (n = 18). (B) Normal versus abnormal karyotype. The median value of p57kip2 expression is clearly lower in patients with poor karyotype compared with that in normal group. (C) The change in p57kip2 before and after treatment. In seven MDS patients who achieved remission, p57kip2 expression increased significantly after treatment (C1, P = 0.009, n = 7). However, in ineffective therapy group, the change in p57kip2 expression was irregularly (C2, P = 0.87, n = 7). (D) There is a negative correlation between p57kip2 and CD34+ cell percentage in MDS patients (r = −0.458, P<0.001, n = 67).
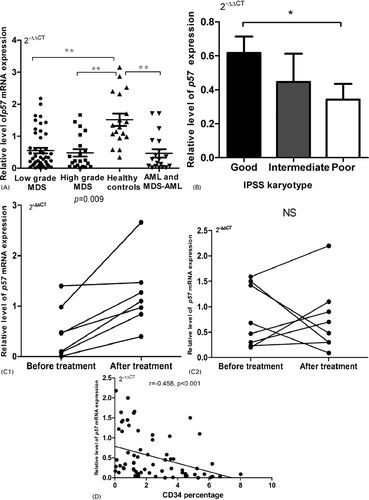
Table 2. The main clinical and laboratory features of the patients
Regarding to karyotype, patients with poor karyotype (according to IPSS) showed lower expression of p57kip2, compared with patients with normal karyotype (B, P = 0.046).
The changes in p57kip2 expression before and after DAC treatment were investigated. As shown in in Appendix, of 22 patients who received DAC, three patients (13.6%) achieved CR, one patient (4.5%) achieved PR and three patients (13.6%) achieved mCR. After treatment, p57kip2 expression was significantly increased in patients who achieved response (C1, before treatment 0.50±0.52 vs after treatment 1.24±0.71, P = 0.009, n = 7), but not in ineffective group ().
Besides, we found a negative correlation between p57kip2 expression and CD34+ cell percentage in MDS cases (D, r = −0.458, P<0.001, n = 67).
Expression of CXCR4 in MDS cases
Furthermore, we also measured CXCR4 levels in BMNCs from patients and healthy controls by qPCR. However, there were no significant differences among the three groups, as shown in A. Surprisingly, we found a positive correlation between CXCR4 and p57kip2 (B, r = 0.568, P<0.001 (n = 67)).
SDF-1 promoted p57kip2 expression dose dependently in normal BMNCs, but not in MDS patients
As shown in , p57kip2 expression in BMNCs of normal control increased significantly when co-cultured with SDF-1 in vitro, which could be blocked by AMD3100. With the dosage increasing, the expression of p57kip2 was increased. SDF-1 could have its maximum impact at the concentration of 50 ng/ml. However, when BMNCs of MDS patients were treated with SDF-1, the increase in p57kip2 expression was weakly.
Figure 3. SDF-1/CXCR4 signal regulated p57kip2 expression. Four MDS cases (two low grade MDS and two high grade MDS) and four healthy volunteers were included. (A) BMMCs from every healthy volunteer were cultured with different concentrations of SDF-1. With the increasing of SDF-1 concentrations, the expression of p57kip2 increased. (B) When BMMCs of MDS patients were treated with SDF-1, p57kip2 was almost not changed. (C) The function of SDF-1 promoted p57kip2 expression would be blocked by AMD3100. (D) The change of p57kip2 expression was quantified by qPCR. In vitro SDF-1 significantly elevated the p57kip2 in BMMCs from the control group but not in MDS patients. (E) CXCR4 protein expression on BMMCs was measured by flow cytometry. There was no significant difference between MDS (n = 4) and healthy controls (n = 4).
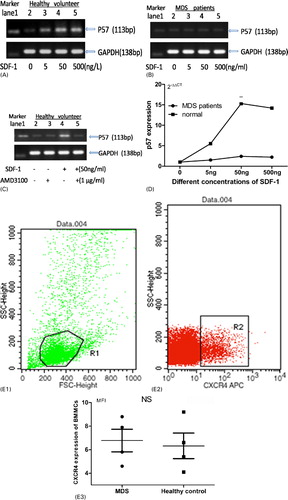
The changes of p57kip2 expression in the presence of SDF-1 were also detected by qPCR. As shown in D, in vitro, SDF-1 treatment increased the p57kip2 level dose dependently in healthy BMNCs. The mean level of p57kip2 expression increased 5.5-fold, 15.3-fold, and 14.2-fold in the presence of 5, 50 and 500 ng/ml of SDF-1, respectively. Whereas, SDF-1 induced a mild increase in p57kip2 expression in MDS compared with normal controls. In the presence of 50 ng/ml of SDF-1, p57kip2 expression only increased 1-fold in MDS.
Moreover, we measured CXCR4 protein expression on BMNCs by flow cytometry. There was no significant difference between MDS and healthy controls. In short, reduced response to SDF-1 was found in the BMNCs of MDS.
Discussion
Myelodysplastic syndromes may originate from a primitive hematopoietic stem cell.Citation21 Altered cell proliferation and apoptosis participate in MDS progression. Abnormality of cell apoptosis in MDS is intensively investigated, but little is known about the changes of cell cycle. The negative regulators of the cell cycle can be grouped in two families: the INK4 family (p15INK4b, p16INK4a, p18INK4c, and p19INK4d) and the Cip/Kip familyCitation22 (p57kip2, p21Cip1, and p27Kip1). In the last few years, many studies had focused on abnormal methylation of cell cycle regulators, such as p15, which is methylated quite often in MDS patients.Citation23 However, the studies on molecules, at the mRNA or protein level, which might influence cell cycle kinetics in MDS, are scarce.
We measured the expression of p57kip2 in de novo myelodysplastic syndromes and tried to clarify the role of p57kip2 in MDS pathogenesis. Our data showed that p57kip2 expression in MDS (including low and grade risk) was significantly lower than that in control group, as well as in AML and MDS-AML group (A). Patients with poor karyotype showed lower p57kip2 than that in the normal group (B). After treatment with DAC, p57kip2 increased in patients who got response (C). A potential explanation of the low p57kip2 expression in MDS comes from its function. P57kip2 null mice were observed hyperproliferation and differentiation delay.Citation24 Low expression of p57kip2 could promote cell cycle progression and cell proliferation. Indeed, increased proliferation and blocked differentiation are two steps associated with the pathogenesis of MDS.Citation25,Citation26 Lower p57kip2 in patients with poor karyotype suggest a proliferative advantage of these cases. It may be related to poor prognosis. These results suggested that low expression of p57kip2, which is benefit to clone proliferation, may be a malignant feature of MDS. In addition, p57kip2 increased in cases who responsed to decitabine treatment. Although the molecular mechanism underlying their clinical activity is unknown, it has been suggested that the drug could selectively suppress malignant cells in the bone marrow and thereby facilitate the expansion of normal bone marrow progenitor cells.Citation27 Therefore, it is possible that effective treatments blocked the growth of MDS clone and increased P57kip2 expression.
CD34+ cells were found to be increased in high grade MDS.Citation28–Citation31 P57kip2 and p27 null HSCs were more proliferative and had reduced capacity to engraft in transplantation.Citation32 We found a negative correlation between p57kip2 and CD34+ cell percentage (D). This finding implied that low expression of p57kip2 is associated with immature cell proliferation. In the research cohort, the expression of P57kip2 in three cases, who had transformed into AML was extremely low. We inferred that p57kip2 may be a candidate tumor suppressor gene in MDS.
We further analyzed the reasons for the low expression of p57kip2 in MDS. Two groups reported that p57kip2 methylation in MDS was infrequent (0/67, 2/52),Citation33,Citation34 so methylation may not play a key role in MDS. In hematologic system, data showed that SDF-1/CXCR4 signaling could induce P57kip2 expression, which maintained HSC in G0/G1.Citation7,Citation35 Most of BMNCs express CXCR4. We measured CXCR4 mRNA expression in MDS cases and healthy volunteers. There was no significant difference between them (A). However, we found a positive correlation between p57kip2 and CXCR4 in MDS patients (B). Therefore, we deduced that SDF-1/CXCR4 signaling may regulate p57kip2 expression in BMNCs.
In vitro, SDF-1 treatment significantly elevated the p57kip2 expression in BMNCs from healthy volunteers but not in MDS cases (A, 3B, and 3D), whereas CXCR4 protein expression on BMNCs of the two groups has no difference (E). Moreover, SDF-1-induced p57kip2 expression could be blocked by AMD3100 (C), a distinctive antagonist of CXCR4. These processes demonstrated that SDF-1/CXCR4 signal could regulate p57kip2 expression in BMNCs. This effect is reduced in MDS patients. It may be ascribed to the impaired SDF-1/CXCR4 signal in MDS. Indeed, many studies have shown that SDF-1/CXCR4 signal is impaired in MDS. Matsuda et al.Citation36 reported that CXCR4 expressed on CD34+ progenitor cells from MDS patients did not respond to SDF-1 effectively. However, the mechanisms were unknown. Fuhler et al.Citation37 found that impaired activation of the PI3K and Rac pathways and decreased F-actin polymerization contribute to the impaired motility of MDS CD34+ cells toward SDF-1. Fuhler et al.Citation38 also found phosphorylation of protein kinase B and extracellular signal-regulated kinase, which are the downstream of SDF-1/CXCR4 signal, is decreased in neutrophils from MDS patients.
In conclusion, our studies have shown that p57kip2 expression in MDS is decreased, especially in poor karyotype group. This may be ascribed to the reduced response to SDF-1/CXCR4 signal. Further research is needed to elucidate the role and the regulation mechanisms of p57kip2 in MDS pathogenesis.
Acknowledgements
This study was supported in part by the National Nature science foundation of China (Grant No. NNSFC81170463).
References
- Tefferi A, Vardiman JW. Myelodysplastic syndromes. N Engl J Med. 2009;361:1872–85.
- Pateras IS, Apostolopoulou K, Niforou K, Kotsinas A, Gorgoulis VG. p57kip2KIP2: ‘Kip’ing the cell under control. Mol Cancer Res. 2009;7:1902–19.
- Economopoulou C, Pappa V, Papageorgiou S, Kontsioti F, Economopoulou P, Charitidou E, et al.. Cell cycle and apoptosis regulatory gene expression in the bone marrow of patients with de novo myelodysplastic syndromes (MDS). Ann Hematol.2010;89:349–58.
- Besson A, Dowdy SF, Roberts JM. CDK inhibitors: cell cycle regulators and beyond. Dev Cell. 2008;14:159–69.
- Kavanagh E, Joseph B. The hallmarks of CDKN1C (p57kip2, KIP2) in cancer. Biochim Biophys Acta. 2011;1816:50–6.
- Guo H, Tian T, Nan K, Wang W. p57kip2: A multifunctional protein in cancer. Int J Oncol. 2010;36:1321–9.
- Nie YC, Han YC, Zou YR. CXCR4 is required for the quiescence of primitive hematopoietic cells. J Exp Med. 2008;205:777–83.
- Teicher BA, Fricker SP. CXCL12 (SDF-1)/CXCR4 pathway in cancer. Clin Cancer Res. 2010;16:2927–31.
- Cashman J, Clark-Lewis I, Eaves A, Eaves C. Stromal-derived factor 1 inhibits the cycling of very primitive human hematopoietic cells in vitro and in NOD/SCID mice. Blood. 2002;99:792–9.
- Cashman J, Dykstra B, Clark-Lewis I, Eaves A, Eaves C. Changes in the proliferative activity of human he-matopoietic stem cells in NOD/SCID mice and enhancement of their transplantibility after in vivo treatment with cell cycle inhibitors. J Exp Med. 1996;196:1141–9.
- Fuhler GM, Drayer AL, Olthof SG, Schuringa JJ, Coffer PJ, Vellenga E. Reduced activation of protein kinase B, Rac, and F-actin polymerization contributes to an impairment of stromal cell derived factor-1 induced migration of CD34+ cells from patients with myelodysplasia. Blood. 2008;111:359–68.
- Kotsianidis I, Bouchliou I, Nakou E, Spanoudakis E, Margaritis D, Christophoridou AV, et al.. Kinetics, function and bone marrow trafficking of CD4+CD25+FOXP3+ regulatory T cells in myelodysplastic syndromes (MDS). Leukemia. 2009;23:510–8.
- Chunkang C, Rui Y, Feng X, Juan G, Xi Z, Lingyun W, et al.. The roles of SDF-1/CXCR4 axis and its relationship with apoptosis in the myelodysplastic syndromes. Med Oncol. 2011;28:S494–500.
- Valent P, Horny HP, Bennett JM, Fonatsch C, Germing U, Greenberg P, et al.. Definitions and standards in the diagnosis and treatment of the myelodysplastic syndromes: consensus statements and report from a working conference. Leuk Res. 2007;31:727–36.
- Vardiman JW, Harris NL, Brunning RD. The World Health Organization (WHO) classification of the myeloid neoplasms. Blood. 2002;100:2292–302.
- Greenberg P, Cox C, LeBeau MM, Fenaux P, Morel P, Sanz G, et al.. International scoring systemfor evaluating prognosis in myelodysplastic syndromes. Blood. 1997;89:2079–88.
- Bennett JM, Catovsky D, Daniel MT. Proposals for the classification of the acute leukaemias: French-American-British co-operative group. Br J Haematol. 1976;33:451–8.
- Greenberg PL, Attar E, Bennett JM, Bloomfield CD, Castro DeCM, Deeg HJ, et al.. NCCN Clinical Practice Guidelines in Oncology: myelodysplastic syndromes. J Natl Compr Canc Netw. 2011;9:30–56.
- Cheson BD, Greenberg PL, Bennett JM, Lowenberg B, Wijermans PW, Nimer SD, et al.. Clinical application and proposal for modification of the International Working Group (IWG) response criteria in myelodysplasia. Blood. 2006;108:419–25.
- Guo J, Cai J, Yu L, Tang H, Chen C, Wang Z. EZH2 regulates expression of p57 and contributes to progression of ovarian cancer in vitro and in vivo. Cancer Sci. 2011;102:530–9.
- Wong AK, Fang B, Zhang L, Guo X, Lee S, Schreck R. Loss of the Y chromosome: an age-related or clonal phenomenon in acute myelogenous leukemia/myelodysplastic syndrome? Arch Pathol Lab Med. 2008;132:1329–32.
- Park MT, Lee SJ. Cell cycle and cancer. J Biochem Mol Biol. 2003;36:60–5.
- Quesnel B, Guillerm G, Vereecque R, Wattel E, Preudhomme C, Bauters F, et al.. Methylation of the p15 (INK4b) gene in myelodysplastic syndromes is frequent and acquired during disease progression. Blood. 1998;91:2985–90.
- Zhang P, Liégeois NJ, Wong C, Finegold M, Hou H, Thompson JC, et al.. Altered cell differentiation and proliferation in mice lacking p57KIP2 indicates a role in Beckwith-Wiedemann syndrome. Nature. 1997;387:151–8.
- Agool A, Schot BW, Jager PL, Vellenga E. 18F-FLT PET in hematologic disorders: a novel technique to analyze the bone marrow compartment. J Nucl Med. 2006;47:1592.
- Bejar R, Levine R, Ebert BL. Unraveling the molecular pathophysiology of myelodysplastic syndromes. J Clin Oncol. 2011;29:504–15.
- Daskalakis M, Nguyen TT, Nguyen C, Guldberg P, Köhler G, Wijermans P, et al.. Demethylation of a hypermethylated P15/INK4B gene in patients with myelodysplastic syndrome by 5-Aza-2'-deoxycytidine (decitabine)treatment. Blood. 2002;100:2957–64.
- Guyotat D, Campos L, Thomas X, Vila L, Shi ZH, Charrin C, et al.. Myelodysplastic syndromes: a study of surface markers and in vitro growth patterns. Am J Hematol. 1990;34:26–31.
- Oertel J, Huhn D. CD34 immunophenotyping of blasts in myelodysplasia. Leuk Lymphoma. 1994;15:65–9.
- Oertel J, Kleiner S, Huhn D. Immunotyping of blasts in refractory anemia with excess of blasts. Br J Haematol. 1993;84:305–9.
- Oertel J, Oertel B, Beyer J, Huhn D. CD34 immunotyping of blasts in myelodysplasia. Ann Hematol. 1994;68:77–80.
- Zou P, Yoshihara H, Hosokawa K, Tai I, Shinmyozu K, Tsukahara F, et al.. p57 (Kip2) and p27 (Kip1) cooperate to maintain hematopoietic stem cell quiescence through interactions with Hsc70. Cell Stem Cell. 2011;9:247–61.
- Brakensiek K, Länger F, Kreipe H, Lehmann U. Absence of p21 (CIP 1), p27 (KIP 1) and p 57(KIP 2) methylation in MDS and AML. Leuk Res. 2005;29:1357–60.
- Hatzimichael E, Dasoula A, Benetatos L. The absence of CDKN1C (p57KIP2) promoter methylation in myeloid malignancies also characterizes plasma cell neoplasms. Br J Haematol. 2008;141:557–8.
- Umemoto T, Yamato M, Nishida K, Yang J, Tano Y, Okano T. p57kip2Kip2 is expressed in quiescent mouse bone marrow side population cells. Biochem Biophys Res Commun. 2005;337:14–21.
- Matsuda M, Morita Y, Hanamoto H, Tatsumi Y, Maeda Y, Kanamaru A. CD34+ Progenitors from MDS patients are unresponsive to SDF-1, despite high levels of SDF-1 in bone marrow plasma. Leukemia. 2004;18:1038–40.
- Fuhler GM, Drayer AL, Olthof SG, Schuringa JJ, Coffer PJ, Vellenga E. Reduced activation of protein kinase B, Rac, and F-actin polymerization contributes to an impairment of stromal cell-derived factor-1 induced migration of CD34+ cells from patients with myelodysplasia. Blood. 2008;111:359–68.
- Fuhler GM, Drayer AL, Vellenga E. Decreased phosphorylation of protein kinase B and extracellular signal-regulated kinase in neutrophils from patients with myelodysplasia. Blood. 2003;101:1172–80.
Appendix
Table 3. The changes of p57 expression before and after DAC treatment