Abstract
Anticoagulants are pivotal for the treatment of debilitating thromboembolic and associated disorders. Current anticoagulants such as heparin and warfarin are non-specific and have a narrow therapeutic window. These limitations have provided the impetus to develop new anticoagulant therapies/strategies that target specific factors in the blood coagulation cascade, ideally those located upstream in the clotting process. Factor VIIa (FVIIa) presents an attractive target as it, in complex with tissue factor (TF), acts as the prima ballerina for the formation of blood clot. A comprehensive review delineating the structure–activity relationship of protein/peptide anticoagulants targeting FVIIa or TF–FVIIa complex is absent in the literature. In this article, we have addressed this deficit by appraising the peptide/protein anticoagulants that target FVIIa/TF–FVIIa complex. Further, the current status of these anticoagulants, with regard to their performance in different clinical trials has also been presented. Lastly, the unexplored domains of these unique proteins have also been highlighted, which will facilitate further translational research in this paradigm, to improve strategies to counter and treat thromboembolic disorders.
Blood coagulation is an innate response to vascular injury that results from a series of amplified reactions in which zymogens of serine protease circulating in the plasma are sequentially activated by limited proteolysis leading to the formation of blood clot thereby preventing the loss of blood. It is initiated through the extrinsic pathway.Citation1 Membrane-bound tissue factor (TF), exposed during vascular injury, interacts with factor VIIa (FVIIa), which pre-exists in the plasma (at 1–2% of total factor VII),Citation2 and forms the extrinsic tenase complex. This complex activates factor X (FX) to factor Xa (FXa). TF–FVIIa also activates factor IX (FIX) to factor IXa (FIXa). Traces of activated FXa are sufficient to produce a small amount of thrombin empowering the cascade to inflate. The two main amplification complexes within this cascade are intrinsic tenase, composed of activated factor VIII (FVIIIa) (in the plasma milieu, FVIII circulates in a stable non-covalent complex with von Willebrand factor. Upon activation by thrombin, it dissociates from the complex) associated with FIXa, and prothrombinase, composed of activated factor V (FVa) (activated by thrombin) associated with FXa. The former activates FX into FXa, the latter, prothrombin into thrombin. Thrombin cleaves fibrinogen to fibrin, promoting the formation of a fibrin clot, and activates platelets for inclusion in the clot. The TF–FVIIa complex can also activate factor IX (FIX) to factor IXa (FIXa), thus helping in the propagation of the coagulation cascade through the intrinsic pathway. The coagulation cascade is under tight regulation. Any imbalance in its regulation could lead to either un-clottable blood, resulting in excessive bleeding during injuries, or unwanted clot formation, resulting in death and debilitation due to vascular occlusion with the consequence of myocardial infarction, stroke, pulmonary embolism, or venous thrombosis. Therefore, there is an urgent need for the prophylaxis and treatment of thromboembolic disorders.
Anticoagulants are crucial for the prevention and treatment of thromboembolic disorders and associated disorders.Citation3 About 0.7% of the western population receives oral anticoagulant treatment. Heparin and coumarins are the most widely used anticoagulants. However, the non-specific mode of action of these anticoagulants accounts for their therapeutic limitations in maintaining a balance between thrombosis and hemostasis. This limitation has provided the impetus for the design of new anticoagulants that target specific coagulation enzymes or steps in the coagulation pathway. These anticoagulants have been developed from proteins isolated and characterized from hematophagous organisms, using recombinant DNA technology, or by structure-based drug design. A state-of-the-art review of the protein anticoagulants targeting TF–FVIIa complex is provided in this article with plausible future investigations that can be carried out in this paradigm.
Appropriateness of TF–FVIIa as a therapeutic target to treat cardiovascular disorders
TF individually has been implicated in the pathogenesis of a plethora of cardiovascular disorders. Its involvement in cardiovascular risk factors namely hypertension,Citation4,Citation5 diabetes,Citation6,Citation7 dyslipidemia,Citation8 and smokingCitation9 as well as its role in atherosclerosisCitation10 and acute coronary syndromesCitation11 are well known and have been investigated extensively. Further TF–FVIIa complex has also been studied elaborately as a likeable molecular target for various thrombosis-related disorders, including unstable angina, acute myocardial infarction, myocardial ischemia-reperfusion injury, venous thrombosis, sepsis, and glomerulonephritis.Citation12–Citation17 Therefore, anticoagulants targeting the complex in addition to mediating a cardio-protective function will also arbitrate secondary beneficial effects as is evident from the above studies.
A wide variety of strategic approaches have been availed for the design and synthesis of inhibitors targeting TF–FVIIa complex, as this complex presents an attractive target for the design/synthesis of anticoagulants, since the concentration of FVIIa in the plasma milieu is ∼10 nM. Thus, as a result, low dose of an anticoagulant will be required to inhibit the coagulation cascade.Citation2,Citation18,Citation19 In addition, TFCitation19 and FVIIa participate in the cascade at an upstream position which will minimize adverse drug-related effects associated with an anticoagulant therapy. The above statement can be levied on to the fact that an anticoagulant inhibiting TF–FVIIa complex may have speculative recompenses over the inhibition of downstream coagulation proteases such as factor Xa (FXa) or thrombin based on the several thousand-fold augmentation that occurs after initiation by TF–FVIIa complex. This may minimize adverse drug-related effects, as the downstream proteases apart from their role in clot formation; also mediate pivotal cell signaling responses. Case in point, thrombin regulates platelet aggregation, endothelial cell activation, and other vital responses in vascular biology through protease-activated receptor signaling.Citation20
Tissue factor pathway inhibitor
Tissue factor pathway inhibitor (TFPI) is a 42 kDa naturally occurring endogenous plasma glycoprotein having 276 amino acid residues. It is a Kunitz-type protease inhibitor consisting of three tandem Kunitz inhibitor domains, the first and second of which inhibit TF–FVIIa complex and FXa, respectively.Citation21,Citation22 The third domain has no inhibitory activity and contains the heparin-binding sites.Citation23 Similar heparin-binding site is also localized in the COOH-terminal basic region of the molecule. Interaction of these TFPI regions with heparin enhances its anticoagulant potency.Citation23 The complete structure of TFPI has not been solved, but the structures of the individual second and third Kunitz domains are available.Citation24,Citation25
The anticoagulant action of TFPI is a two-stage process. The second Kunitz domain first binds to a molecule of FXa and deactivates it. The first domain then rapidly binds to an adjacent TF–FVIIa complex, preventing further activation of FX.Citation26,Citation27 Interestingly, the first kunitz domain of TFPI itself inhibits TF–FVIIa with a Ki of 250 nM.Citation28 A chimeric protein has been designed by linking the first Kunitz domain of TFPI to the COOH-terminal of the light chain of FX. The chimera potently inhibits TF–FVIIa in the absence of FX/FXa with 14 times more potency than TFPI.Citation29
Recombinant TFPI (tifacogin) has been compared with placebo in phases 230 and 331 clinical trials. The promising results of the phase 2 trial were not confirmed in the phase 3. Thus, further development of TFPI for anticoagulant therapy has been halted. A highly homologous protein termed TFPI-2 has also been characterized,Citation32 though its use as an antithrombotic is still to be tested. A truncated version of TFPI containing only the first and second Kunitz domains has also demonstrated antithrombotic effects in animal models.Citation33
Nematode anticoagulant protein c2
Nematode anticoagulant protein c2 (NAPc2) is an 8 kDa polypeptide originally isolated from the canine hookworm, Ancylostomacaninum.Citation34 The recombinant protein, rNAPc2, is expressed in yeast. The protein contains two antiparallel β-sheets and a short helix ().Citation35 Both natural and recombinant NAPc2 potently inhibit TF–FVIIa complex by a unique mechanism, which requires the initial anchoring of the protein to FX or FXa prior to the formation of a final quaternary inhibitory complex with TF–FVIIa (TF-VIIa-Xa-NAPc2) (). The use of FXa as an inhibitory scaffold for the inhibition of TF–FVIIa complex by NAPc2 appears similar to the mechanism of TF–FVIIa inhibition by TFPI (discussed above). However, unlike TFPI, which specifically requires an un-occupied active site on FXa to allow binding, NAPc2 interacts with FX or FXa via an extended binding site or exo-site. In addition, the unique interaction of NAPc2 with FXa allows the catalytic site of the FXa to remain unobstructed and thus enzymatically active in the quaternary inhibitory complex. However, FXa bound to NAPc2 can cleave only peptidyl substrates but not prothrombin.Citation36
Figure 1. (A) Ribbon diagrams of the second and third Kuniz domain of TFPI. (B) Mechanism of action of TFPI: inhibitory mechanisms of the initial reaction of blood coagulation by TFPI on cell surface. TFPI binds to FVIIa and Xa via K1 and K2 domains and to proteoglycans via K3 and C-terminal domains (K denotes Kunitz domain) (diagram kindly provided by Dr Jun Mizuguchi). (C) Ribbon diagram of the minimized mean structures of NAPc2 (kindly provided by Dr Peter E Wright). The molecules on the right have been rotated about the vertical axis by 90°. Beta-Strands are shown in cyan and helices in red and yellow. The cysteine side chains are shown in gold. (D) Mechanism of action of rNAPc2. In step 1 of the scheme, rNAPc2 binds with high affinity to zymogen FX in solution (binding to FXa as an inhibitory scaffold is not shown). The resulting stable bimolecular complex between rNAPc2–FX serves as an inhibitory scaffold that facilitates docking to the membrane-bound TF–FVIIa. In this way, the inhibitory scaffold uses both a similar docking mechanism as the natural substrate (FX) and subsequent presentation to the active site of TF–FVIIa. In the last step of the mechanistic scheme, the canonical reactive loop of rNAPc2 docks into the active site of FVIIa/TF, allowing for the formation of tightly bound quaternary inhibitory complex.
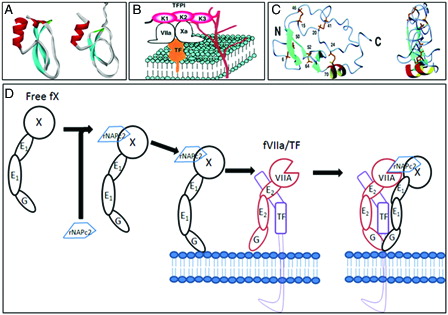
NAPc2 has a half-life of approximately 50 hours after subcutaneous injection. Its anticoagulant activity was evaluated in an open-label phase 2 dose-finding study of 293 patients undergoing elective knee arthroplasty and showed promising results. The safety of NAPc2 has also been assessed in a randomized, double-blind, placebo-controlled dose-escalation study of 154 subjects undergoing elective coronary angioplasty in combination with aspirin, clopidogrel, and heparin.Citation37 Additionally, the ANTHEM-TIMI-32 trial showed that the protein was well tolerated in patients with non-ST-segment elevation acute coronary syndrome.Citation38
Ixolaris
This anticoagulant has been isolated from hard tick and Lyme disease vector, Ixodesscapularis. It has been shown to inhibit TF–FVIIa-induced FX activation with an inhibitory concentration of 50% (IC50) in the picomolar range. At high concentration, Ixolaris attenuates the amidolytic activity of TF–FVIIa; however, in the presence of DEGR-FX or DEGR-FXa (but not des-Gla-DEGR-FXa), Ixolaris becomes a tight inhibitor of TF–FVIIa as assessed by recombinant FIX activation assays. This indicates that FX and FXa are scaffolds for Ixolaris in the inhibition of TF–FVIIa and implies that the Gla domain is necessary for TF–FVIIa–Ixolaris-FX(a) complex formation.Citation39 Although, the protein has been extensively investigated recently in its ability to attenuate tumor growth,Citation40 it is still to be appraised in clinical trials.
Active site inhibitors
Certain proteins inhibit FVIIa by directly binding to the active site. These are Kunitz-type inhibitors, containing about 58 amino acid residues and three conserved disulfide bonds. They are slow tight-binding, reversible inhibitors of serine proteases that bind to the active site and inhibit according to the standard mechanism.Citation41 Kunitz domain inhibitors of TF–FVIIa has also been selected from Alzheimer's amyloid beta-protein precursor inhibitor (APPI) and basic pancreatic trypsin inhibitor Kunitz domain libraries displayed on phage. An overall consensus inhibitor designated as TF7I-C, differing from APPI in four residues inhibited TF–FVIIa with an apparent Ki of 1.9 nM. But it also inhibited FXIa, plasma kallikrein, and plasmin.Citation42 Later, the Kunitz domain of TF7I-C was utilized to develop a bi-functional inhibitor by covalently linking it to the TF mutant TFAA (TF with amino acid alanine at positions 165 and 166). The bivalent fusion protein inhibited TF–FVIIa activity specifically with 200-fold more potency than the individual components.Citation43
A bovine pancreatic trypsin inhibitor (BPTI) variant designated as 5LI5, selected from phage display libraries and differing in eight residues from BPTI-inhibited TF–FVIIa with a Ki of 0.4 nM.Citation44 The crystal structure of the complex of 5LI5 with FVIIa has been solved, shading important lights on the mechanism of action.Citation45 Also, 5LI5 has been studied in platelet-rich venous thrombosis models in hamsters yielding promising results.Citation46 However, this class of inhibitors have not been evaluated in clinical trials, the reason for which may be the non-specific mode of inhibition as observed in the case of TF7I-C.
Active site-inhibited FVIIa (factorVIIai)
FactorVIIai (FVIIai) is inactivated FVIIa where either dansyl-l-Glu-l-Gly-l-Arg or d-Phe-l-Phe-l-Argchloromethyl ketone have been used to alkylate the active site.Citation47 It behaves completely as a non-competitive inhibitor of FX activation; where the inhibition reaction proceeds through a non-productive ternary TF–FVIIai–FX complex.Citation48 Interestingly, the affinity of TF is five-fold higher for FVIIai than FVIIa itself.Citation49
Based on promising results in animal models of thrombosis,Citation50 treatment with FVIIai, in doses ranging from 50 to 400 µg/kg with or without adjunctive heparin, was compared with treatment with heparin alone in patients undergoing elective percutaneous coronary interventions. The rates of major bleeding were similar with therapy with FVIIai plus heparin and heparin alone. Because of these disappointing results, FVIIai has not been developed further.
Modified TF
Lysine residues at positions 165 and 166 of TF are important for substrate recognition by the TF–FVIIa complex.Citation51,Citation52 A soluble TF mutant (TFAA; alanine at both these positions), containing alanines at the above positions was constructed for use as a specific anticoagulant. TFAA binds to FVIIa and the resultant complex lacks FX activation activity. Moreover, in the plasma TFAA forms complex with FVII and this phenomenon further attenuates its anticoagulant function as evident from in vitro studies. Studies conducted in arterial thrombosis models in rabbit with TFAA have shown anti-thrombotic activity with reduced bleeding in comparison to heparin. Further, amino acid substitutions were made in TFAA, increasing its affinity for FVIIa by 20-fold.
Antibody to FVII/FVIIa (Corsevin M™)
A monoclonal antibody to FVII/FVIIa (Corsevin M™, licensed from Corvas International (San Diego, CA, USA), is being studied by Johnson & Johnson in clinical trials. The antibody binds to the protease domain of both FVII and FVIIa in the presence or absence of TF with high affinity and shows mixed type of inhibition for FX activation. A Fab fragment of the antibody reduced circulating FVII and FVIIa levels.Citation53 In a phase I study, Corsevin M™ showed immediate and dose-related anticoagulation without adverse effects.Citation54
Antibody to TF
A plethora of antibody preparations directed against the TF have been investigated in vivo. They can be grouped into two different classes: (A) The first type interferes with the binding of TF to FVIIa,Citation55,Citation56 whereas the second type interferes with substrate docking without affecting the TF binding to FVIIa.Citation57
In a rabbit carotid artery thrombosis model, a monoclonal anti-TF Ab attenuated the formation of thrombus and decreased tissue plasminogen activator lysis time.Citation58 In the line with the above administration of a monoclonal anti-TF Ab reduced infarct size in a rabbit coronary artery ligation model, as result of inhibition of chemokine expression and leukocyte migration to the site of infarct. A polyclonal antibody to TF has been appraised in humans and has been found to reduce thrombogenicity of disrupted atherosclerotic plaques.Citation59 Sunol-cH36, a chimeric monoclonal antibody to TF has been evaluated in humans. It exhibited dose-dependent anticoagulant effects. However, mucosal bleeding was observed as one of the adverse events.Citation60
Inhibitor of TF synthesis
A hairpin ribozyme has been designed that destroys TF mRNA, abrogating the induction of TF protein expression and activity in vascular smooth muscle cells.Citation61 A strategy involving the use of antisense oligonucleotides, hybridizing to their complementary target mRNA and thereby preventing translation of the protein, has been employed in reducing the synthesis of TF in monocytes.Citation62 However, the above strategies because of involving plausible adverse side effects such as unintentional mutagenesis have not been evaluated in clinical trials.
Peptide inhibitors
Exosite inhibitors
Two different classes of peptide inhibitors (A-series and E-series peptides) of FVIIa inhibitors have recently been discovered using phage display libraries.Citation63–Citation65 A- and E-series of peptides have no sequence identity. Both classes of peptides bound to the protease domain of FVIIa, to two distinct exosites to inhibit extrinsic tenase activity.
The A-series represented by the 15-mer cyclic peptide A-183 (EEWEVLCWTWETCER) is a mixed-type inhibitor (Ki ∼200 pM) of FX activation with an IC50 of 1.6 nM. Structural studies showed that the binding of A-183 causes a conformational change in FVII, which disrupts the potential substrate-binding sites.Citation65
The E-series of peptides represented by 18 residue peptide E-76 (Ac-ALCDDPRVDRWYCQFVEG-NH2), has a kd of 8.5 nM for FVIIa and TF–FVIIa complex (Dennis et al., 2000) and inhibits both enzymatic and amidolytic activity of TF–FVIIa (IC50 of ∼1 nM). Structural studies with E-76 complexed with FVIIa showed the mechanism of inhibition to be a conformational change in the activation loops (the 140 seconds loop) of FVIIa. It has also been postulated that E-76 also disrupts the hydrogen bond conformation at the oxyanion hole, which is important for stabilization of the transition state of the substrate during catalysis.
In another anticoagulant design strategy, the A- and E-series peptides were covalently linked together to make fusion peptides.Citation64 The A-linker-E bifunctional peptides are potent anticoagulants that specifically inhibit of TF–FVIIa-catalyzed activation of FX.
Cyclic peptide inhibitor
The cyclic pentapeptide Cys-Gln-Gln-Tyr-Cys inhibitor was derived from a tripeptide motif within the second epidermal growth factor (EGF) domain of FVII.Citation66 This peptide is a specific and non-competitive inhibitor of TF–FVIIa complex, although in comparison with the exosite binding peptides its inhibitory potency is low.
Hemextin AB complex
Hemextin AB complex is a potent inhibitor of coagulation FVIIa isolated and characterized from the venom of Hemachatus haemachatus (African Ringhals cobra). The complex consists of two synergistically acting anticoagulant three-finger proteins, hemextin A and hemextin B. Hemextin A but not hemextin B exhibits mild anticoagulant activity. However, hemextin B interacts with hemextin A and forms a complex (hemextin AB complex), and synergistically enhances its anticoagulant potency. Prothrombin time assay showed that these two proteins form a 1:1 complex. Using a ‘a dissection approach’, it was found that hemextins A and AB complex prolong clotting by inhibiting extrinsic tenase activity. Further studies showed that hemextin AB complex potently inhibits the proteolytic activity of FVIIa and its complexes.Citation67–Citation69 Kinetic studies showed that hemextin AB complex is a non-competitive inhibitor of FVIIa-soluble TF proteolytic activity with a K(i) of 25 nM. Hemextin AB complex is the first reported natural inhibitor of FVIIa that does not require either TF or factor Xa scaffold to mediate its inhibitory activity. Molecular interactions of hemextin AB complex with FVIIa/TF–FVIIa may provide a new paradigm in the search for anticoagulants inhibiting the initiation of blood coagulation.
Conclusions and future directions
This review identified 11 well-characterized protein anticoagulants that target FVIIa, the prima ballerina in the sequential activation of prothrombin to thrombin. Out of the depicted protein anticoagulants targeting factor VIIa, NAPc2 has shown great promise in the clinical trials but a number of other protein anticoagulants mentioned have not yet been investigated in great depth. Case in point, the ribozyme technology based on which the anti-thrombotic strategy was conceived had limitations with regard to the retroviral gene delivery vector. Further as delineated in the article by Cavusoglu et al., replication of target cells is pivotal for the pro-viral integration.Citation70 Therefore, it is questionable as to whether stable high-level gene expression in-vivo can be attained with retrovirally transduced cells. Additionally, the long-term safety of retroviral vectors is still to be investigated in detail as integration into chromosomal DNA could result in disregulation of genes or mutagenesis.Citation71 However, the ribozyme technology to design an anticoagulant is important as this technology can be successfully employed before injury associated with balloon dilatation or other pre-cutaneous techniques. Addressing the above limitations will therefore facilitate in the development of an anticoagulant that can be used successfully for various invasive techniques.
Our review article illustrates two naturally occurring protein anti-coagulants that through specific and well-characterized mechanisms target factor VIIa: NAPc2 and hemextin AB complex. Of this NAPc2 has been successfully tested and wetted in clinical trials. This indicates that animal venoms and saliva of hematophagus organisms provides a researcher with an unopened treasure chest of novel therapeutic leads, in this particular scenario, protein anti-coagulants targeting FVIIa. Transcriptomic and proteomic approaches, therefore, can be availed to screen novel anticoagulants from natural sources specifically saliva of hematophagus organisms and animal venom. In this regard, currently our laboratory is investigating the venom of a less studied saw-scaled viper belonging to the Echis species – Echis omanensis to screen for novel anticoagulants including those targeting FVIIa. Further, novel proteins can be used as templates to generate chimeric proteins with increased anticoagulant potency specifically the A and E peptides identified by phage display binding to FVIIa exosite, where these proteins can be conjugated with inhibitors binding to the active site of the enzyme. A similar strategy was used in the design of chimeric protein with both pro/anticoagulant activities. This protein christened the sTF-annV consists of amino acid residues 1 to 218 of TF (soluble-TF), a linker region, and the entire coding sequence for annexin V (a human phosphatidylserine-binding protein). Specific anticoagulants depicted above have not been evaluated in clinical trials albeit initial studies have shown promise. These apart from being promising therapeutic leads may direct the design of successful FVIIa targeting anticoagulant agents.
References
- Davie EW, Fujikawa K, Kisiel W. The coagulation cascade: initiation, maintenance, and regulation. Biochemistry. 1991;30(43):10363–70.
- Edgington TS, Dickinson CD, Ruf W. The structural basis of function of the TF. VIIa complex in the cellular initiation of coagulation. Thromb Haemost. 1997;78(1):401–5.
- Al-Amoudi SM. Anticoagulant induced leukoagglutination. Saudi Med J. 2008;29(8):1192–3.
- Felmeden DC, Spencer CG, Chung NA, Belgore FM, Blann AD, Beevers DG, et al. Relation of thrombogenesis in systemic hypertension to angiogenesis and endothelial damage/dysfunction (a substudy of the Anglo-Scandinavian Cardiac Outcomes Trial [ASCOT]). Am J Cardiol. 2003;92(4):400–5.
- Napoleone E, Di SA, Camera M, Tremoli E, Lorenzet R. Angiotensin-converting enzyme inhibitors downregulate tissue factor synthesis in monocytes. Circ Res. 2000;86(2):139–43.
- Boeri D, Almus FE, Maiello M, Cagliero E, Rao LV, Lorenzi M. Modification of tissue-factor mRNA and protein response to thrombin and interleukin 1 by high glucose in cultured human endothelial cells. Diabetes. 1989;38(2):212–8.
- Aljada A, Ghanim H, Mohanty P, Kapur N, Dandona P. Insulin inhibits the pro-inflammatory transcription factor early growth response gene-1 (Egr)-1 expression in mononuclear cells (MNC) and reduces plasma tissue factor (TF) and plasminogen activator inhibitor-1 (PAI-1) concentrations. J Clin Endocrinol Metab. 2002;87(3):1419–22.
- Wada H, Kaneko T, Wakita Y, Minamikawa K, Nagaya S, Tamaki S, et al. Effect of lipoproteins on tissue factor activity and PAI-II antigen in human monocytes and macrophages. Int J Cardiol. 1994;47 (Suppl 1):S21–5.
- Matetzky S, Tani S, Kangavari S, Dimayuga P, Yano J, Xu H, et al. Smoking increases tissue factor expression in atherosclerotic plaques: implications for plaque thrombogenicity. Circulation. 2000;102(6):602–4.
- Hansson GK. Inflammation, atherosclerosis, and coronary artery disease. N Engl J Med. 2005;352(16):1685–95.
- Annex BH, Denning SM, Channon KM, Sketch MH, Stack RS, Morrissey JH, et al. Differential expression of tissue factor protein in directional atherectomy specimens from patients with stable and unstable coronary syndromes. Circulation. 1995;91(3):619–22.
- Wilcox JN, Smith KM, Schwartz SM, Gordon D. Localization of tissue factor in the normal vessel wall and in the atherosclerotic plaque. Proc Natl Acad Sci USA. 1989;86(8):2839–43.
- Kaikita K, Ogawa H, Yasue H, Takeya M, Takahashi K, Saito T, et al. Tissue factor expression on macrophages in coronary plaques in patients with unstable angina. Arterioscler Thromb Vasc Biol. 1997;17(10):2232–7.
- Erlich JH, Holdsworth SR, Tipping PG. Tissue factor initiates glomerular fibrin deposition and promotes major histocompatibility complex class II expression in crescentic glomerulonephritis. Am J Pathol. 1997;150(3):873–80.
- Erlich JH, Boyle EM, Labriola J, Kovacich JC, Santucci RA, Fearns C, et al. Inhibition of the tissue factor-thrombin pathway limits infarct size after myocardial ischemia-reperfusion injury by reducing inflammation. Am J Pathol. 2000;157(6):1849–62.
- Himber J, Wohlgensinger C, Roux S, Damico LA, Fallon JT, Kirchhofer D, et al. Inhibition of tissue factor limits the growth of venous thrombus in the rabbit. J Thromb Haemost. 2003;1(5):889–95.
- Taylor FB, Chang A, Ruf W, Morrissey JH, Hinshaw L, Catlett R, et al. Lethal E. coli septic shock is prevented by blocking tissue factor with monoclonal antibody. Circ Shock. 1991;33(3):127–34.
- Al-Nozha MM, Abdel-Gader AG, Arafah MR, Al-Maatouq MA, Al-Shahid MS, Al-Harthi SS, et al. Tissue factor pathway inhibitor, natural coagulation inhibitors and hemostatic activation markers in patients with acute coronary syndromes. Saudi Med J. 2005;26(6):937–42.
- Lwaleed BA. Tissue factor: biological function and clinical significance. Saudi Med J. 2002;23(2):135–43.
- Coughlin SR. Thrombin signalling and protease-activated receptors. Nature. 2000;407(6801):258–64.
- Ndonwi M, Tuley EA, Broze GJ The Kunitz-3 domain of TFPI-alpha is required for protein S-dependent enhancement of factor Xa inhibition. Blood. 2010;116(8):1344–51.
- Bajaj MS, Birktoft JJ, Steer SA, Bajaj SP. Structure and biology of tissue factor pathway inhibitor. Thromb Haemost. 2001;86(4):959–72.
- Hamamoto T, Yamamoto M, Nordfang O, Petersen JG, Foster DC, Kisiel W. Inhibitory properties of full-length and truncated recombinant tissue factor pathway inhibitor (TFPI). Evidence that the third Kunitz-type domain of TFPI is not essential for the inhibition of factor VIIa-tissue factor complexes on cell surfaces. J Biol Chem. 1993;268(12):8704–10.
- Burgering MJ, Orbons LP, van der Doelen A, Mulders J, Theunissen HJ, Grootenhuis PD, et al. The second Kunitz domain of human tissue factor pathway inhibitor: cloning, structure determination and interaction with factor Xa. J Mol Biol. 1997;269(3):395–407.
- Mine S, Yamazaki T, Miyata T, Hara S, Kato H. Structural mechanism for heparin-binding of the third Kunitz domain of human tissue factor pathway inhibitor. Biochemistry. 2002;41(1):78–85.
- Baugh RJ, Broze GJ, Krishnaswamy S. Regulation of extrinsic pathway factor Xa formation by tissue factor pathway inhibitor. J Biol Chem. 1998;273(8):4378–86.
- Panteleev MA, Zarnitsina VI, Ataullakhanov FI. Tissue factor pathway inhibitor: a possible mechanism of action. Eur J Biochem. 2002;269(8):2016–31.
- Petersen LC, Bjorn SE, Olsen OH, Nordfang O, Norris F, Norris K. Inhibitory properties of separate recombinant Kunitz-type-protease-inhibitor domains from tissue-factor-pathway inhibitor. Eur J Biochem. 1996;235(1–2):310–6.
- Girard TJ, MacPhail LA, Likert KM, Novotny WF, Miletich JP, Broze GJ. Inhibition of factor VIIa-tissue factor coagulation activity by a hybrid protein. Science. 1990;248(4961):1421–4.
- Abraham E, Reinhart K, Svoboda P, Seibert A, Olthoff D, Dal NA, et al. Assessment of the safety of recombinant tissue factor pathway inhibitor in patients with severe sepsis: a multicenter, randomized, placebo-controlled, single-blind, dose escalation study. Crit Care Med. 2001;29(11):2081–9.
- Abraham E, Reinhart K, Opal S, Demeyer I, Doig C, Rodriguez AL, et al. Efficacy and safety of tifacogin (recombinant tissue factor pathway inhibitor) in severe sepsis: a randomized controlled trial. JAMA. 2003;290(2):238–47.
- Petersen LC, Sprecher CA, Foster DC, Blumberg H, Hamamoto T, Kisiel W. Inhibitory properties of a novel human Kunitz-type protease inhibitor homologous to tissue factor pathway inhibitor. Biochemistry. 1996;35(1):266–72.
- Holst J, Lindblad B, Bergqvist D, Nordfang O, Ostergaard PB, Petersen JG, et al. Antithrombotic effect of recombinant truncated tissue factor pathway inhibitor (TFPI1–161) in experimental venous thrombosis – a comparison with low molecular weight heparin. Thromb Haemost. 1994;71(2):214–9.
- Cappello M, Vlasuk GP, Bergum PW, Huang S, Hotez PJ. Ancylostoma caninum anticoagulant peptide: a hookworm-derived inhibitor of human coagulation factor Xa. Proc Natl Acad Sci USA. 1995;92(13):6152–6.
- Duggan BM, Dyson HJ, Wright PE. Inherent flexibility in a potent inhibitor of blood coagulation, recombinant nematode anticoagulant protein c2. Eur J Biochem. 1999;265(2):539–48.
- Lee AY, Vlasuk GP. Recombinant nematode anticoagulant protein c2 and other inhibitors targeting blood coagulation factor VIIa/tissue factor. J Intern Med. 2003;254(4):313–21.
- Lee A, Agnelli G, Buller H, Ginsberg J, Heit J, Rote W, et al. Dose-response study of recombinant factor VIIa/tissue factor inhibitor recombinant nematode anticoagulant protein c2 in prevention of postoperative venous thromboembolism in patients undergoing total knee replacement. Circulation. 2001;104(1):74–8.
- Giugliano RP, Wiviott SD, Stone PH, Simon DI, Schweiger MJ, Bouchard A, et al. Recombinant nematode anticoagulant protein c2 in patients with non-ST-segment elevation acute coronary syndrome: the ANTHEM-TIMI-32 trial. J Am Coll Cardiol. 2007;49(25):2398–407.
- Monteiro RQ, Rezaie AR, Ribeiro JM, Francischetti IM. Ixolaris: a factor Xa heparin-binding exosite inhibitor. Biochem J. 2005;387(Pt 3):871–7.
- Carneiro-Lobo TC, Konig S, Machado DE, Nasciutti LE, Forni MF, Francischetti IM, et al. Ixolaris, a tissue factor inhibitor, blocks primary tumor growth and angiogenesis in a glioblastoma model. J Thromb Haemost. 2009;7(11):1855–64.
- Bode W, Huber R. Natural protein proteinase inhibitors and their interaction with proteinases. Eur J Biochem. 1992;204(2):433–51.
- Dennis MS, Lazarus RA. Kunitz domain inhibitors of tissue factor-factor VIIa. I. Potent inhibitors selected from libraries by phage display. J Biol Chem. 1994;269(35):22129–36.
- Lee GF, Lazarus RA, Kelley RF. Potent bifunctional anticoagulants: Kunitz domain-tissue factor fusion proteins. Biochemistry. 1997;36(19):5607–11.
- Stassen JM, Lambeir AM, Matthyssens G, Ripka WC, Nystrom A, Sixma JJ, et al. Characterisation of a novel series of aprotinin-derived anticoagulants. I. In vitro and pharmacological properties. Thromb Haemost. 1995;74(2):646–54.
- Zhang E, St CR, Tulinsky A. Structure of extracellular tissue factor complexed with factor VIIa inhibited with a BPTI mutant. J Mol Biol. 1999;285(5):2089–104.
- Stassen JM, Lambeir AM, Vreys I, Deckmyn H, Matthyssens G, Nystrom A, et al. Characterisation of a novel series of aprotinin-derived anticoagulants. II. Comparative antithrombotic effects on primary thrombus formation in vivo. Thromb Haemost. 1995;74(2):655–9.
- Taylor FB Role of tissue factor and factor VIIa in the coagulant and inflammatory response to LD100 Escherichia coli in the baboon. Haemostasis. 1996;26 (Suppl 1):83–91.
- Nemerson Y, Gentry R. An ordered addition, essential activation model of the tissue factor pathway of coagulation: evidence for a conformational cage. Biochemistry. 1986;25(14):4020–33.
- Sorensen BB, Persson E, Freskgard PO, Kjalke M, Ezban M, Williams T, et al. Incorporation of an active site inhibitor in factor VIIa alters the affinity for tissue factor. J Biol Chem. 1997;272(18):11863–8.
- Jang Y, Guzman LA, Lincoff AM, Gottsauner-Wolf M, Forudi F, Hart CE, et al. Influence of blockade at specific levels of the coagulation cascade on restenosis in a rabbit atherosclerotic femoral artery injury model. Circulation. 1995;92(10):3041–50.
- Roy S, Hass PE, Bourell JH, Henzel WJ, Vehar GA. Lysine residues 165 and 166 are essential for the cofactor function of tissue factor. J Biol Chem. 1991;266(32):22063–6.
- Ruf W, Miles DJ, Rehemtulla A, Edgington TS. Cofactor residues lysine 165 and 166 are critical for protein substrate recognition by the tissue factor-factor VIIa protease complex. J Biol Chem. 1992;267(9):6375–81.
- Dickinson CD, Shobe J, Ruf W. Influence of cofactor binding and active site occupancy on the conformation of the macromolecular substrate exosite of factor VIIa. J Mol Biol. 1998;277(4):959–71.
- Biemond BJ, Levi M, ten CH, Soule HR, Morris LD, Foster DL, et al. Complete inhibition of endotoxin-induced coagulation activation in chimpanzees with a monoclonal Fab fragment against factor VII/VIIa. Thromb Haemost. 1995;73(2):223–30.
- Pawashe AB, Golino P, Ambrosio G, Migliaccio F, Ragni M, Pascucci I, et al. A monoclonal antibody against rabbit tissue factor inhibits thrombus formation in stenotic injured rabbit carotid arteries. Circ Res. 1994;74(1):56–63.
- Golino P, Ragni M, Cirillo P, Avvedimento VE, Feliciello A, Esposito N, et al. Effects of tissue factor induced by oxygen free radicals on coronary flow during reperfusion. Nat Med. 1996;2(1):35–40.
- Huang M, Syed R, Stura EA, Stone MJ, Stefanko RS, Ruf W, et al. The mechanism of an inhibitory antibody on TF-initiated blood coagulation revealed by the crystal structures of human tissue factor, Fab 5G9 and TF.G9 complex. J Mol Biol. 1998;275(5):873–94.
- Ragni M, Cirillo P, Pascucci I, Scognamiglio A, D'Andrea D, Eramo N, et al. Monoclonal antibody against tissue factor shortens tissue plasminogen activator lysis time and prevents reocclusion in a rabbit model of carotid artery thrombosis. Circulation. 1996;93(10):1913–8.
- Badimon JJ, Lettino M, Toschi V, Fuster V, Berrozpe M, Chesebro JH, et al. Local inhibition of tissue factor reduces the thrombogenicity of disrupted human atherosclerotic plaques: effects of tissue factor pathway inhibitor on plaque thrombogenicity under flow conditions. Circulation. 1999;99(14):1780–7.
- Morrow DA, Murphy SA, McCabe CH, Mackman N, Wong HC, Antman EM. Potent inhibition of thrombin with a monoclonal antibody against tissue factor (Sunol-cH36): results of the PROXIMATE-TIMI 27 trial. Eur Heart J. 2005;26(7):682–8.
- Cavusoglu E, Chen I, Rappaport J, Marmur JD. Inhibition of tissue factor gene induction and activity using a hairpin ribozyme. Circulation. 2002;105(19):2282–7.
- Stephens AC, Rivers RP. Suppression of human monocyte tissue factor synthesis by antisense oligodeoxynucleotide. Thromb Res. 1997;85(5):387–98.
- Dennis MS, Eigenbrot C, Skelton NJ, Ultsch MH, Santell L, Dwyer MA, et al. Peptide exosite inhibitors of factor VIIa as anticoagulants. Nature. 2000;404(6777):465–70.
- Roberge M, Santell L, Dennis MS, Eigenbrot C, Dwyer MA, Lazarus RA. A novel exosite on coagulation factor VIIa and its molecular interactions with a new class of peptide inhibitors. Biochemistry. 2001;40(32):9522–31.
- Eigenbrot C, Kirchhofer D, Dennis MS, Santell L, Lazarus RA, Stamos J, et al. The factor VII zymogen structure reveals reregistration of beta strands during activation. Structure. 2001;9(7):627–36.
- Orning L, Fischer PM, Hu CK, Agner E, Engebretsen M, Husbyn M, et al. A cyclic pentapeptide derived from the second EGF-like domain of Factor VII is an inhibitor of tissue factor dependent coagulation and thrombus formation. Thromb Haemost. 2002;87(1):13–21.
- Banerjee Y, Mizuguchi J, Iwanaga S, Kini RM. Hemextin AB complex, a unique anticoagulant protein complex from Hemachatus haemachatus (African Ringhals cobra) venom that inhibits clot initiation and factor VIIa activity. J Biol Chem. 2005;280(52):42601–11.
- Banerjee Y, Mizuguchi J, Iwanaga S, Kini RM. Hemextin AB complex – a snake venom anticoagulant protein complex that inhibits factor VIIa activity. Pathophysiol Haemost Thromb. 2005;34(4–5):184–7.
- Banerjee Y, Lakshminarayanan R, Vivekanandan S, Anand GS, Valiyaveettil S, Kini RM. Biophysical characterization of anticoagulant hemextin AB complex from the venom of snake Hemachatus haemachatus. Biophys J. 2007;93(11):3963–76.
- Miller DG, Adam MA, Miller AD. Gene transfer by retrovirus vectors occurs only in cells that are actively replicating at the time of infection. Mol Cell Biol. 1990;10(8):4239–42.
- Cone RD, Mulligan RC. High-efficiency gene transfer into mammalian cells: generation of helper-free recombinant retrovirus with broad mammalian host range. Proc Natl Acad Sci USA. 1984;81(20):6349–53.