Abstract
Background
Frequent diagnostic radiology procedures in allogeneic stem cell transplantation (SCT) recipients raise concern about the potential harm from incidental radiation.
Objectives
To determine the cumulative radiation dose from diagnostic studies in allogeneic SCT and its impact on clinical outcome.
Patients and methods
This retrospective cohort study was conducted to determine the cumulative radiation dose from diagnostic studies following SCT. Sixty-four consecutive patients with hematological malignancies in a single tertiary care institution underwent total body irradiation (TBI)-based myeloablative conditioning followed by six of six human leukocyte antigen (HLA)-identical sibling allogeneic SCT. The median follow-up was 3 years. The cumulative effective dose in mSv from diagnostic radiological studies in the peri-transplant period from day −30 to day +200 was calculated for each patient and its impact on overall survival and non-relapse mortality was determined.
Results
The median cumulative radiation exposure from diagnostic radiological procedures was 92 mSv (range 1.2–300), representing about 30× the normal annual background radiation for the population and 10% of the 1200 cGy TBI dose used in conditioning. Sixty-five percent of the cumulative radiation exposure was delivered between day +1 and day 100 and computed tomography scans contributed 88%. In multivariate analysis, diagnostic procedures did not significantly impact clinical outcomes.
Conclusions
While radiation exposure from diagnostic procedures did not impact clinical outcomes the risk of secondary cancers in long-term survivors is likely to be increased. Our results indicate that patients who are acutely ill for prolonged periods can receive clinically significant radiation doses during their hospital care. Our findings should prompt attempts to limit radiation exposure from diagnostic procedures in post-SCT recipients
Keywords:
Introduction
Outcomes in allogeneic hematopoietic stem cell transplantation (HSCT) are compromised by high complication rates attributable both to the underlying disease as well as to the treatment. Transplant recipients undergo rigorous evaluation prior to transplant to determine transplant eligibility. Clinical evaluation frequently takes the form of diagnostic radiology procedures such as computed tomography (CT) scans, X-rays, nuclear medicine (NM) studies, and fluoroscopy. Following transplant, many patients experience prolonged hospitalization and repeated diagnostic radiological procedures and may incur a significant cumulative radiation exposure. The health impact of incidental radiation exposure from diagnostic studies, particularly delayed cancer risk is becoming more widely appreciated. Since allogeneic SCT conditioning regimens including total body irradiation (TBI) incur an increased risk of secondary cancers,Citation1 it is important to determine the contribution that radiological diagnostic procedures add to the total radiation dose received.
Frequent medical imaging is commonplace in oncology and recent studies have begun to raise concerns about radiation risks.Citation2,Citation3 Late malignancies are already a documented problem in allogeneic stem cell transplant survivors, with the magnitude of risk of secondary malignancies after SCT ranging from 4- to 11-fold that of the general population.Citation4 The frequency of utilization of diagnostic radiology procedures in allogeneic HSCT has not been well characterized and the attendant risk of radiation exposure is completely unknown. We conducted a single-institute retrospective study of radiation exposure in the peri-transplant period extending from day –30 to day 200 to define this risk.
Patients and methods
Patients
Sixty-four consecutive patients (age 13–68 years) underwent myeloablative six of six HLA-matched sibling allogeneic HSCT for hematological malignancies at our center between October 2006 and September 2011. All patients gave written informed consent for National Institutes of Health protocols, approved by the National Heart, Lung, and Blood Institute Institutional Review Board (protocol 06-H-0248 (NCT 00378534, FDA IDE #13058) and protocol 07-H-0136 (NCT00467961, FDA IND #13305)). 06-H-0248 and 07-H0136 are contemporaneous protocols with comparable clinical outcomes for overall survival (OS), relapse, and non-relapse mortality (NRM).Citation5
Transplant conditions
Recipients received conditioning with fludarabine 125 mg/m2, cyclophosphamide 120 mg/kg and TBI of either 12 cGy (age <55 years) or 4 cGy (age ≥55 years).
Granulocyte colony stimulating factor (G-CSF)-mobilized peripheral blood grafts underwent CD34+ selection by the Miltenyi CliniMacs system, with infusion of a target CD34+ dose of 6 × 106/kg (range 3–10 × 106/kg) and a fixed CD3+ dose of 5 × 104/kg.
Twenty-four patients (PD cohort) received 5 × 106 CD3+/kg donor lymphocytes selectively depleted of alloreactivity by ex vivo photo depletion (Kiadis),Citation6 while the remaining patients (TCD cohort) received a prophylactic unmanipulated donor lymphocyte infusion of 5 × 106 CD3+/kg on day 90 in the absence of graft versus host disease (GVHD).
Low-dose cyclosporine (100–200 ng/ml) till day 21 was the sole GVHD prophylaxis.
Radiation exposure
All 66 patients were evaluated for diagnostic radiology exposure. Diagnostic radiology procedures (X-rays, CT Scans, fluoroscopy, and NM) were extracted from the electronic medical record and documented for each individual in three periods (day −30 to day 0, day +1 to day100, and day +101 to day 200). Pre-transplant studies in the 30 days prior to transplant were included to capture diagnostic radiology for restaging and eligibility determination, which includes a routine CT scan of the chest, abdomen, and pelvis at our center. Radiation exposure was calculated using the ‘effective dose’ (ED) in mSv. The ED is a means of converting a non-uniform (or localized) radiation dose to a dose that would be equivalent to a whole-body exposure in a reference patient. Given the considerable variations in ED based on machine characteristics, the majority of common diagnostic studies had their ED computed by direct measurements conducted by NIH Radiation Safety. For infrequent studies, values were assigned from literature review. Since no standards were found for interventional radiology procedures, they were assigned an ED equivalent to 5 minutes of fluoroscopic exposure (Supplementary ). ED is defined as total body absorbed dose (whole-body equivalent) required to achieve an adequate diagnostic image. The biological effects vary by the type and energy of the radiation and the organism and tissues involved. The unit of ED is the Sievert (1 Sievert (Sv) = 1 joule/kilogram (J/kg)).
Table
All patients received therapeutic TBI in their conditioning regimen. The unit of the absorbed dose of radiation in therapeutic radiation is the gray (1 Gy = 1 J/kg). Forty-eight patients who were <55 years old received 12 Gy (divided in eight fractions with lung shielding to 600 cGy) and 16 patients who were ≥55 years old received 4 Gy (divided in eight fractions without lung shielding).
The relationship between the dose equivalent and the absorbed dose is given by: dose equivalent (Sieverts) is the product of dose (grays) and the quality factor (Q). The Q for X-ray, gamma, or beta radiation (covering all forms of radiation exposure from diagnostic radiation) is 1.Citation7 For comparison purposes in this study, 1 Sv (radiation equivalent) = 1 Gy (radiation absorbed) = 1 J/kg.
Statistical analysis
Nine patients failed to survive >200 days and were excluded from study of the impact of diagnostic radiation exposure on clinical outcomes. OS and NRM were evaluated in the remaining 55 subjects who survived >200 days in univariate analysis (Kaplan–Meier estimate) Citation8 and multivariable cox proportionate hazard models.Citation9 In order to compensate for the confounding association between diagnostic radiology procedures and patient morbidity, serum albumin and serum creatinine (as alternative surrogates for patient complexity) were introduced into the multivariable models. All variables were tested for the proportionate hazards model. The variables for the cumulative and the periodic ED were forced into the multivariable models at all steps of model building regardless of statistical significance. A backward and forward stepwise model building approach was used. Statistical analysis was performed using SPSS (IBM, PASW 18, version 18.0.3). Variables that attained a P ≤ 0.05 were held in the final multivariate models.
Results
Patient characteristics and transplant outcomes
Pre-transplant patient and donor characteristics are summarized in . The median time to an absolute neutrophil count >500/μl was 12 days and there were two episodes of secondary graft failure. The incidence of acute GVHD grades II–IV and III–IV were 18.8 and 4.7%, respectively. Limited and extensive chronic GVHD occurred in 29.1 and 27.2% of transplant recipients. Fifty-five subjects survived beyond the 200-day landmark and were evaluable for survival analysis. At a median follow-up of 36 months, the Kaplan–Meier estimates for the cumulative incidence of relapse/progression, non-relapse mortality, and OS were 19.4, 34.3, and 56.2%.
Diagnostic radiology procedures
Between day −30 and day 200, patients received a median of 11 diagnostic X-rays, 10 CT scans, 1 fluoroscopic, and 0 NM studies. The median cumulative radiation exposure from diagnostic radiology procedures was 91.6 mSv (range 1.2–300.1).
In terms of time frame, 29% of the cumulative ED was delivered between day −30 and day 0, 65% between day +1 and day 100, and only 6% between day +101 and day 200. In terms of diagnostic modalities, the relative contributions from CT scans, X-rays, fluoroscopy, and NM were 88, 6, 5, and <1%, respectively ().
Cumulative diagnostic radiation and clinical outcomes
A greater than median cumulative dose of incidental radiation from day −30 to day +100 was significantly associated with NRM and OS (P < 0.03) in univariate analysis. In multivariable analysis, cumulative doses of radiation lost significance and were displaced by serum creatinine and serum albumin in the final models for OS, and by albumin, acute GVHD, and photodepletion for NRM (, ).
Figure 2. Univariate survival analysis for the cumulative effective dose of diagnostic radiation. Univariate Kaplan–Meier survival analyses show inferior outcomes for cumulative ED greater than the median for (A) OS (day −30 to day +200), (B) OS (day −3 to day +100), and (C) non-relapse mortality (day −30 to day +100).
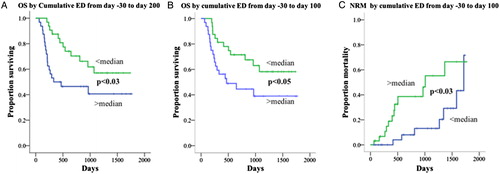
Table
Discussion
Most estimates of harmful effects of low-dose radiation derive from studies of atomic bomb survivors in Japan. Radiation doses ranging from 5 to 150 mSv with a mean of about 40 mSv produced a definite increase in cancer risk.Citation10,Citation11
Radiation impact from diagnostic procedures has been difficult to quantify epidemiologically, because of debate regarding the linearity of the dose–response curves at low doses of radiation, recall bias, long latency till cancer development, non-uniform exposure, and marked differences in X-ray energies produced by diagnostic machines.Citation12,Citation13 However, a large cohort study involving over 400 000 nuclear radiation workers showed a 1–2% dose-related increase in cancer deaths from chronic exposure to low levels of radiation, even at cumulative doses as low as 5–50 mSv.Citation14 This data are consistent with the conclusion of Biological Effects of Ionizing Radiation VII, which showed ‘a linear, no-threshold dose–response relationship between exposure to ionizing radiation and the development of cancer in humans’.Citation13 Recurrent CT scans have been singled out as a major concern and children are particularly vulnerable.Citation15–Citation17 In one risk estimate, de Gonzalez, et al.Citation18 estimate that approximately 29 000 (95% UL, 15 000–45 000) future cancers could be related to CT scans performed in the USA in 2007. In response, the FDA has developed a collaborative initiative to reduce unnecessary radiation exposure from medical imaging.Citation19 The initiative focuses on the three types of procedures that are the greatest contributors to the US population's total radiation exposure from medical imaging: CT, fluoroscopy, and NM studies.
Our patients received a median of 92 mSv. This is 30× greater than the average annual radiation exposure from all sources in the USA of about 3.0 mSv and double the accepted limit of 50 mSv per year for healthcare workers.Citation20 Furthermore, some patients received radiation doses up to 100-fold greater than the annual radiation exposure. Between day +1 and day 100 post-SCT, CT scans represented approximately 90% of the radiation exposure and peak exposure (65%). While excessive for a healthy individual, the median total radiation exposure from diagnostic radiation (92 mSv) represented around 10% of the myeloablative conditioning radiation dose given at our center. Consistent with this, cumulative dose of incidental radiation exposure did not affect NRM and OS multivariable analysis. Nevertheless, such excess radiation from diagnostic procedures would be more significant after reduced intensity regimens, popular for patients over the age of 55 who may receive conditioning regimens with no or very low-dose TBI.
Our study is limited by the lack of organ-specific measurements of radiation dose, which was not available for all the diagnostic radiology procedures performed. Secondly, follow-up was too short to examine impact on secondary cancers which are a concern in long-term SCT survivors.Citation21 Nevertheless, as discussed above, this possibly avoidable diagnostic radiation has the potential to adversely impact long-term outcome after SCT since the risk of secondary malignancies is directly related to cumulative radiation dose received.
While our study involved a largely adult SCT population, it should be noted that children are the most vulnerable population for adverse events from radiation exposure. The EDs of radiation in medical imaging are considerably greater in pediatric recipients for reasons that are not well understood Citation22,Citation23 and children's hospitals are leading the way in reducing diagnostic radiation exposure. Growing awareness of the hazards of cumulative radiation exposure has stimulated efforts to reduce diagnostic radiation exposure by limiting clinically unnecessary restaging and duplicate studies (e.g. PET/CTs), eliminating multiphase studies (e.g. liver imaging), and diminishing image detail without reducing diagnostic accuracy.
In conclusion, although diagnostic radiology procedures can be expected to only modestly increase the overall radiation exposure of SCT, any unnecessary radiation exposure is worth avoiding. Our findings should stimulate greater awareness of the large doses of radiation accumulated in post-transplant recipients, who can remain acutely sick for months and prompt efforts to minimize such exposure.
Authorship
M.B. and J.B. designed the study; F.F., D.D., J.K.K., N.A.J., K.C., Z.M., P.A.P., and S.I. contributed to collect data; M.B., J.B., and F.F. analyzed and interpreted statistical data and wrote the manuscript. All authors reviewed and approved the manuscript.
yhem_a_11692202_sm0001.pdf
Download PDF (118.1 KB)Acknowledgments
This research was supported by the Intramural Research Program of the National Heart, Lung, and Blood Institute, National Institutes of Health.
References
- Curtis RE, Rowlings PA, Deeg HJ, Shriner DA, Socie G, Travis LB, et al. Solid cancers after bone marrow transplantation. N Engl J Med. 1997;336:897–904
- Murano T, Tateishi U, Iinuma T, Shamin N, Daisaki H, Terauchi T, et al. Evaluation of the risk of radiation exposure from new 18FDG PET/CT plans versus conventional X-ray plans in patients with pediatric cancers. Ann Nucl Med. 2010;24:261–7.
- Marti JL, Dauer LT, Stempel M, Patil S, Kaplan JB, Montgomery LL. Cumulative imaging radiation exposure following breast-conservation therapy. Ann Surg Oncol. 2011;18:104–8.
- Majhail NS. Old and new cancers after hematopoietic-cell transplantation. Hematology Am Soc Hematol Educ Program 2008:142–9.
- Battiwalla M, McIver Z, Klotz J, Draper D, Haggerty J, Chawla K, et al. Outcomes after CD34+ selection with or without the addition of photo-allodepleted T lymphocytes in myeloablative HLA-matched sibling transplantation. Biol Blood Marrow Transplant. 2012;18:S260.
- Mielke S, McIver ZA, Shenoy A, Fellowes V, Khuu H, Stroncek D, et al. Selectively T cell-depleted allografts from HLA-matched sibling donors followed by low-dose posttransplantation immunosuppression to improve transplantation outcome in patients with hematologic malignancies. Biol blood Marrow Transplant. 2011;17:1855–61.
- NRC Regulations: Title 10, Code of Federal Regulations PART 20 – STANDARDS FOR PROTECTION AGAINST RADIATION. § 201004 Units of radiation dose. USA. http://www.nrc.gov/reading-rm/doc-collections/cfr/part020/part020-1004.html
- Kaplan EL, Meier P. Nonparametric estimation from incomplete observations. J Am Statist Assn. 1958;53:457–81.
- Cox DR. Regression models and life-tables. J R Stat Soc B Methodol. 1972;34:187–220.
- Preston DL, Ron E, Tokuoka S, Funamoto S, Nishi N, Soda M, et al. Solid cancer incidence in atomic bomb survivors: 1958–1998. Radiat Res. 2007;168:1–64.
- Ron E, Preston DL, Mabuchi K. More about cancer incidence in atomic bomb survivors: solid tumors, 1958–1987. Radiat Res. 1995;141:126–7.
- Brenner DJ, Doll R, Goodhead DT, et al. Cancer risks attributable to low doses of ionizing radiation: assessing what we really know. Proc Natl Acad Sci USA 2003;100:13761–6.
- Committee to Assess Health Risks from Exposure to Low Levels of Ionizing R, National Research C. Health Risks from Exposure to Low Levels of Ionizing Radiation: BEIR VII Phase 2. The National Academies Press; 2006.
- Cardis E, Vrijheid M, Blettner M, et al. Risk of cancer after low doses of ionising radiation: retrospective cohort study in 15 countries. BMJ 2005;331:77.
- Sodickson A, Baeyens PF, Andriole KP, et al. Recurrent CT, cumulative radiation exposure, and associated radiation-induced cancer risks from CT of adults. Radiology 2009;251:175–84.
- Brenner DJ, Hall EJ. Computed tomography – an increasing source of radiation exposure. N Engl J Med. 2007;357:2277–84.
- Risk of ionizing radiation exposure to children: a subject review. American Academy of Pediatrics. Committee on Environmental Health. Pediatrics 1998;101:717–9.
- de Gonzalez BA, Mahesh M, Kim KP, Bhargavan M, Lewis R, Mettler F, et al. Projected cancer risks from computed tomographic scans performed in the United States in 2007. Arch Intern Med. 2009;169:2071–7.
- FDA. FDA Unveils Initiative to Reduce Unnecessary Radiation Exposure from Medical Imaging. 2010.
- ACGIH. Threshold limit values for chemical substances and physical agents & biological exposure. The American Conference of Governmental Industrial Hygienists (ACGIH). 2012.
- Rizzo JD, Curtis RE, Socie G, Gilbert E, Landgren O, Travis WD, et al. Solid cancers after allogeneic hematopoietic cell transplantation. Blood. 2009;113:1175–83.
- Frush D. CT dose and risk estimates in children. Pediatr Radiol. 2011;41:483–7.
- Blake GM, Naeem M, Boutros M. Comparison of effective dose to children and adults from dual X-ray absorptiometry examinations. Bone 2006;38:935–42.