Abstract
Introduction
C-X-C chemokine receptor type 4/stromal-derived factor-1 (CXCR4/SDF-1) axis dynamically mediates hematopoietic stem cell trafficking in the bone marrow (BM). Granulocyte colony-stimulating factor (G-CSF) as the most effective mobilizing agent induces SDF-1 secretion from BM stromal cells into circulation that recruit CXCR4+ cells such as hematopoietic stem cells (HSCs) into circulation. However, the direct effect of G-CSF on CXCR4 expression of HSC remains unknown. The nervous system regulates HSC migration with effecting on adrenergic receptors. On the other hand, interaction of G-CSF and catecholamines has been demonstrated; hence, we examined the direct effect of G-CSF and catecholamine on CXCR4 expression.
Material and methods
After enrichment of CD34+ HSCs from the cord blood with magnetic-activated cell sorting (MACS), these cells were exposed to G-CSF (100 ng/ml), epinephrine (10 µM), isoproterenol (10 µM), and propranolol (1 µM) separately and together.
Results
Our results showed that G-CSF have no direct effect on CXCR4 expression on human CD34+ cells in vitro and treating HSCs with epinephrine leads to significantly increased CXCR4 in 1, 3, and 5 hours. Epinephrine and G-CSF-induced up-regulation of CXCR4 mRNA is dependent on beta receptors, so incubation of HSCs with propranolol led to inhibition of such increased expression. In addition, isoproterenol and agonist of beta receptors would significantly increase the expression of CXCR4 approximately 4- and 12-fold after 1-hour incubation, respectively.
Discussion
Co-stimulation of enriched HSCs with G-CSF and isoproterenol resulted to a further enhanced CXCR4 levels. In general, G-CSF-induced CXCR4 expression is the indirect mechanism and is specifically regulated through beta-adrenergic receptors.
Introduction
Under normal homeostatic conditions, the majority of hematopoietic stem cells (HSCs) are detectable in the bone marrow (BM) surrounded by a highly organized micro-environment.Citation1 In humans, a small number of HSCs are present in the blood stream during steady-state situation.Citation2 The prototypical agent used in the stem cell transplant, granulocyte colony-stimulating factor (G-CSF), chemotherapeutic agents such as cyclophosphamide, and chemokines can induce HSCs’ egression of BM.Citation3 Mobilized peripheral blood HSCs have become the principal cellular source to reconstitute the hematopoietic system following myeloablative therapy.Citation4 Currently, G-CSF is the most effective agent to induce HSC mobilization.Citation5 However, the mechanisms governing G-CSF-induced HSC's mobilization have not been defined.
Accumulating evidence has emerged that the interaction of stromal-derived factor-1 (SDF-1) with its cognate receptor, C-X-C chemokine receptor type 4 (CXCR4), is essential for the maintenance of HSCs within their niches and may play an important role in regulating G-CSF-induced HSC mobilization.Citation6 SDF-1 and CXCR4 are highly expressed by stromal cells including endothelium, reticular cells, and osteoblasts.Citation7,Citation8 Moreover, CXCR4 receptors are expressed by most hematopoietic cells.Citation8 Studies of SDF-1- or CXCR4-deficient mice have established that these molecules are necessary in vivo homing and re-population potential in transplanted nonobese diabetic, severe combined immunodeficient (NOD/SCID) mice by the more matured CD34+/CD38+ cells.Citation9,Citation10 Moreover, it is stated that the administration of AMD3100, a specific antagonist of CXCR4, is resulted in the loss of HSCs’ retention in BM, and induces rapid and robust HSC mobilization in both human and mice.Citation11 Finally, it has been shown that SDF-1 protein expression in the BM is significantly decreased following G-CSF treatment.Citation12 However, a specific role of SDF-1/CXCR4 interactions as a key step in G-CSF-induced mobilization of HSCs has not yet been elucidated.
The sympathetic nervous system via catecholamines signaling recently emerged as a novel mediator of stem and progenitor cell mobilization in steady state, as well as the following G-CSF administration by the suppression of osteoblast function and the supportive stromal micro-environment.Citation13,Citation14 Additional evidence for a mutual functional relevance between the sympathetic innervation and HSCs’ mobilization has been demonstrated by studying on catecholamines, expression of their receptors on human HSCs, and the ability of neural fibers for controlling BM micro-environment like secretion of proteolytic enzymes.Citation11,Citation14 In , the effect of catecholamines has been shown on most factors involved in HSC mobilization.
Table 1. Effect catecholamines on genes and cytokines-related HSCs’ mobilization
Therefore, the aim of this study was to further characterize the role of G-CSF and catecholamines in the CXCR4 regulation of HSCs. We found that the effect of G-CSF on CXCR4 is indirect and requires adrenergic signals. Our study indicated a main function for catecholamines in G-CSF-induced CXCR4 expression.
Material and methods
Purification of cord blood HSCs
Human cord blood cells were obtained from full-term deliveries after informed consent. Accumulated samples were diluted 1:1 in phosphate buffered saline (PBS). MNCs were separated over a Ficoll-Hypaque gradient and washed in PBS. CD34+ HSCs’ enrichment was done with the magnetic-activated cell sorting (MACS) cell isolation kit and was immunomagnetically selected in accordance with the manufacturer's instructions. The purity of the isolated CD34+ HSCs population was evaluated by flow cytometry after labeling with PE-HPCA2 anti-CD34 monoclonal antibody (Miltenyi Biotec, Bergisch Gladbach, Germany, http://www.miltenyibiotec.com).
Treatment with drugs
After isolation, HSCs (2 × 105 cells/ml) cultured in StemSpan medium supplemented with thrombopoietin (TPO) 50 ng/ml, stem cell factor (SCF) 50 ng/ml, and fms-like tyrosine kinase 3 ligand (FLT3L) 50 ng/ml at 37°C for 5 hours and exposed with previously described doses of epinephrine 10 µM (Sigma, China), isoproterenol 10 µM (Sigma), propranolol 1 µM (Sigma), and G-CSF 100 ng/ml.Citation24,Citation25
RNA isolation and quantitative RT-PCR
After treatment of HSCs with described dose of G-CSF, epinephrine, propranolol, and isoproterenol, the total RNA was extracted using the acidic phenol extraction protocol followed by TRIsol RNeasy Mini Kit purification according to the manufacturer's recommendations (Invitrogen Corp., USA). cDNA was reverse-transcribed from 5 µg of the total RNA obtained from purified CD34+ HSCs using oligo-dT primers (Invitrogen Corp.). Quantitative PCR analysis was performed using the LightCycler system (Roche Diagnostics Corp.) with SYBR green staining of DNA double strands. Cycling conditions were as follows: 5 minutes at 95°C, 30 seconds at 94°C, 20 seconds at 58°C, and 20 seconds at 72°C for 40 cycles. The following primer pairs were used: CXCR4 forward, GGTCCATGGTTACCAGAAGA; CXCR4 reverse, CCTGGAAGCTGTTGTTCCATG. Primers of housekeeping (glyceraldehyde-3-phosphate dehydrogenase (GAPDH)) included sense, 5-CCTGGCGTCGTCATTAGTAGTG-3, and anti-sense, 5-TCAGTCCTGTCCATAATTAGTCC-3. The ABI PCR analysis was performed using the SYBR green master mix, according to the manufacturer's recommendations (ABI, USA). Specificity of product was ensured by melting point analysis and agarose gel electrophoresis.
Statistical analysis
Results were expressed as mean ± standard deviation (SD). Statistical analysis was performed using SPSS 19. The paired t-test and ANOVA followed by Tukey's test were used to analyze the expression levels of CXCR4 expression between control and cells exposed with agents. The values of P < 0.05 were considered as a significant level.
Results
G-CSF directly has no effect on the expression of CXCR4 mRNA
Preliminary results have shown that CXCR4 expression would up-regulate on BM human HSCs in mice treated with G-CSF.Citation12 Thus, we incubated human cord blood HSCs with or without G-CSF (100 ng/mL). By using quantitative PCR, we found that levels of CXCR4 mRNA were no change (data not shown). Therefore, these data showed that the regulation of CXCR4 mRNA expression in the BM following the injection of G-CSF is probably an indirect mechanism.
Epinephrine induces the expression of CXCR4 mRNA
In recent reports, we found that the catecholamines emerged as a novel regulator of stem egress from the BM in steady-state situation, as well as following G-CSF administration.Citation25 To investigate this hypothesis that G-CSF possibly regulates CXCR4 gene expression via catecholamines, we used exposed HSCs with epinephrine as an agonist of adrenergic receptors. Using this system, CXCR4 expression was analyzed in the presence of (10 µM) epinephrine. CXCR4 was detected at high levels in CD34+ HSCs during the initial 1 hour of incubation, but levels were decreased after 3–5 hours of culture (). However, each of the 3 hours was significant compared to control. These data demonstrated catecholamine-induced CXRD4 of HSCs and the direct role of the nervous system in regulating the CXR4/SDF-1 axis.
G-CSF up-regulated CXCR4 requires adrenergic receptors stimulation
We examined the effects of G-CSF on CXCR4 mRNA levels in the presence of 10 µM epinephrine. Our results showed that G-CSF could significantly increase levels of CXCR4 in CD34+ HSCs only in the presence of epinephrine, beginning 1 hour after addition to culture. Although increased CXCR4 persists at the subsequent time points (3 and 5 hours), in comparison with 1 hour, decreased CXCR4 expression was found out (A). Therefore, we demonstrated that G-CSF-induced CXCR4 expression requires cooperation of stimulation of neural receptors. Further, the direct study indicated that G-CSF plus epinephrine-elevated expression of CXCR4 was significant compared with alone-added epinephrine to HSCs’ medium culture (B).
Figure 2. Effect of G-CSF and epinephrine on CXCR4 expression level; CXCR4 mRNA was measured by quantitative PCR. (A) Cord blood HSCs were cultured for 1–5 hours in the presence of G-CSF (100 ng/ml) plus epinephrine (10 µM). (B) Comparing effect of alone epinephrine (10 µM) with epinephrine (10 µM) plus G-CSF (100 ng/ml) on CXCR4 expression of HSCs. The results reflect the means of triplicate determinations; the error bars represent SD of the mean. *P < 0.05, **P < 0.01.
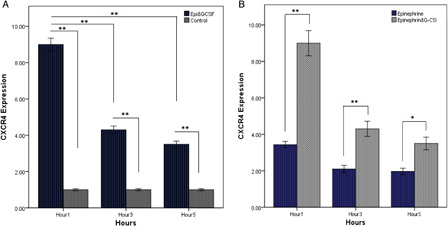
Activation of beta-adrenergic receptors has a major role in G-CSF-induced CXCR4
Epinephrine is an agonist for alpha- and beta-adrenergic receptors.Citation26 Data have shown that beta is one of the important receptors on HSC.Citation27 To determine whether epinephrine and G-CSF-induced up-regulation of CXCR4 mRNA is dependent on the beta receptor, we, therefore, measured the rate of CXCR4 in the presence of the antagonist of beta receptor (propranolol 1 µM). By quantitative PCR (A), the increased expression noted after stimulation of CD34+ HSCs with G-CSF and epinephrine was ablated when beta-adrenergic receptors were inhibited by the addition of conditioned medium with propranolol. Indeed, the results indicated that epinephrine-induced CXCR4 mRNA was through beta-adrenergic receptors. To further examine the potential contribution of catecholamine and G-CSF to the increase of CXCR4 mRNA expression, we measured the effect of isoproterenol as a beta agonist on CXCR4. The data showed that isoproterenol would significantly increase the expression of CXCR4 mRNA approximately four-fold after 1-hour incubation at 37°C (B). We found levels of CXCR4 at subsequent hours (3 and 5 hours) to be similarly reduced in cells exposed to epinephrine (B) and assert the main role of beta receptors in catecholamine-stimulated CXCR4. Finally, co-stimulation of enriched HSCs with G-CSF (100 ng/ml) and isoproterenol (10 µM) was resulted to a further enhanced CXCR4 levels (C). As expected, CXCR4 expression was highest after 1 hour and then steadily reduced after 3- and 5-hour treatment (C). Furthermore, CXCR4 expression in 1 hour was substantial further that other both hours were illustrating immediate activity of catecholamine and G-CSF in the expression of the CXCR4/SDF-1 axis. Direct comparison of isoproterenol with a combination of agents of G-CSF and isoproterenol on CXCR4 expression showed a substantial difference between two groups (D).
Figure 3. The direct role of beta-adrenergic receptors on expression of CXCR4. (A) Quantitative RT-PCR analysis of CXCR4 mRNA in HSCs for 5 hours of incubation with G-CSF (100 ng/ml) and epinephrine (10 µM) plus propranolol (1 µM). (B) Cord blood HSCs were incubated with isoproterenol (10 µM) for 5 hours. The results are expressed as mean (±SD) of triplicate cultures. (C) Levels of CXCR4 mRNA were measured by quantitative PCR. G-CSF (100 ng/mL) plus isoproterenol (10 µM) increases the expression of CXCR4 in CD34+ HSCs. (D) Studying the effect of alone isoproterenol (10 µM) with isoproterenol (10 µM) plus G-CSF (100 ng/ml) on CXCR4 expression of HSCs. The results reflect the mean fold (±SD) mRNA change relative to cells cultured as a control. *P < 0.05, **P < 0.01.
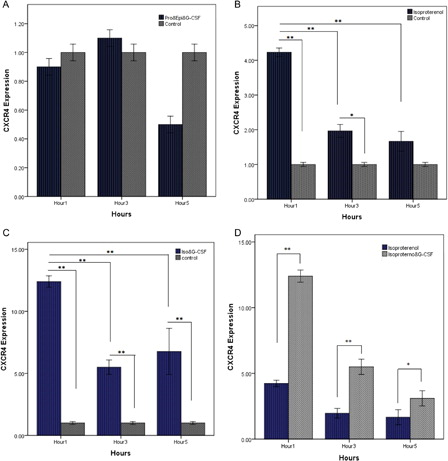
Discussion
Today, circulating HSCs associated with G-CSF injection is used as the main cells source for BM transplant in various diseases such as leukemia. Sometimes, despite appropriated doses of G-CSF injection, insufficient HSCs would release into circulation known poor mobilization that have an effect on outcome and therapeutic process patients. Hence, to optimize HSCs’ collection, the identification of mechanisms and strategies involved in HSCs’ egression from BM is considered important. There are fundamental evidences for a crucial role of the SDF-1/CXCR4 axis in the maintenance of HSC in the BM.Citation28 Studies have indicated that disruption of the SDF-1/CXCR4 axis induced by AMD3100 leads to mobilization of CD34+ HSCs from the BM to the peripheral circulation, but the mechanisms of this destruction are partially known.Citation11
In this study, we investigated the role of epinephrine, beta-adrenergic agonist (isoproterenol), and G-CSF in the expression of CXCR4 of HSCs. Consistent with previous observation, we found that G-CSF itself could not have any direct effect on CXCR4 expression on human CD34+ HSCs in vitro.Citation12 G-CSF administration to mice up-regulates CXCR4 on HSCs; however, it seems such cytokine need to an indirect pathway such as neutrophil elastase, degrading adhesion molecules, or even catecholamines. Different studies have demonstrated that mice-lacking proteolytic enzymes have sufficient HSCs’ migration. Furthermore, denervated neural endings of BM tissue lead to significantly decreased HSCs’ mobilization. So, the role of the nervous system in BM micro-environment was highlighted and suggested that G-CSF indirect mechanism to up-regulate HSCs CXCR4 regulates via catecholamines. Catecholamines are present in substantial amounts in BM and synthesis in both from nerve endings and BM cells such as macrophages and lymphocytes.Citation29,Citation30 Moreover, several studies have suggested that G-CSF could selectively stimulate neural fibers to secrete catecholamines into BM micro-environments.Citation13,Citation19 Here, we reported that epinephrine could significantly increase the expression of CXCR4. Such increase was transient in which the highest CXCR4 levels were seen in the early hours. Epinephrine influences both adrenergic receptors. The adrenergic receptors constitute a family of closely related proteins, which is found on many cells, including BM hematopoietic cells.Citation14,Citation31 In this study, we analyzed type receptors involved in stimulation of HSCs mobilization via this catecholamine. Blocking beta-adrenergic receptors with propranolol abrogated the role of epinephrine on CXCR4 expression. Moreover, we observed a four-fold increased expression of CXCR4 after treating HSCs with an agonist of beta adrenergic. The question was still remaining, regarding the co-stimulation of G-CSF and catecholamines on CXCR4 expression. To address this question, we examined that adding simultaneously epinephrine or isoproterenol with G-CSF into HSCs medium led to substantial CXCR4 expression. Previous studies have shown that G-CSF up-regulates adrenergic receptors on immature human CD34+ cells.Citation14 The second question was how HSC with increased CXCR4 expression could egress from BM and what mechanism is there behind such mobilization? G-CSF injection into mice would induce a reduction of SDF-1 in BM, whereas it increases their secretion into the blood circulation.Citation12,Citation32 Further, the role of catecholamines in suppressed SDF-1 in BM has been determined.Citation11,Citation33 Indeed, SDF-1 gradient in circulation would recruit HSCs with increased CXCR4. Studies have indicated that injection of G-CSF/AMD3100 contributes to HSCs’ migration to SDF-1 gradients.Citation6,Citation34 We determined repetitive CXCR4 stimulation with catecholamine or catecholamine associated with G-CSF reduced its expression compared to 1 hour. Such down-regulation of CXCR4 expression of HSCs may contribute to reduce the tendency of these cells to homing to BM.
According to the proposed model, releasing catecholamines may play an important role in temporary up-regulation of CXCR4 of HSCs induced by G-CSF in the BM that would regulate trafficking of HSCs between BM and the circulation (). On the basis of our results, we propose that myeloid cytokines such as G-CSF have indirect functions in the regulation of human CD34+ cells. These cytokines affect neural endings in BM, increasing catecholamine secretion into BM compartments. On the other hand, G-CSF induces up-regulation of adrenergic receptors on HSCs, thus augmenting their response to neurotransmitters, leading to enhanced mobilization of HSC to the circulation.Citation35 Hence, catecholamine would influence on various molecular of BM compartments such as CXCR4 and SDF-1 expression that finally provide situation to egress HSCs to the circulation. Our study has broadened the understanding of the function of the sympathetic nervous system in HSCs’ mobilization; however, the signaling pathway that mediates the effects of catecholamines on the CXCR4 expression of CD34+ HSCs is unknown.
Figure 4. Mobilization of hematopoietic stem cell induced by G-CSF and catecholamines (1) G-CSF-induced up-regulation of catecholamines from the sympathetic neuron. (2) Catecholamines effect on CXCR4 and SDF-1 expression. (3) Expression of SDF-1 is reduced in BM environment and is secreted into circulation, and CXCR4 expression in hematopoietic is increased inducing egression of HSC from BM. SDF-1, stromal-derived factor-1; CXCR4, CXC motif, receptor 4; HSC, hematopoietic stem cell; G-CSF, granulocyte colony-stimulating factor.
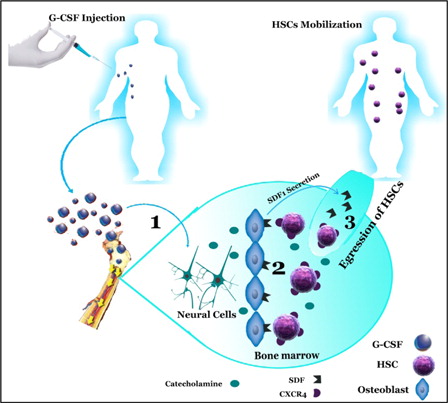
Acknowledgement
We wish to thank all our colleagues in Tarbiat Modares University.
Disclaimer statements
Contributors MS provided laboratory instruments, conceived the manuscript, and revised it; FS performed the technical laboratory tests and wrote the manuscript; SK, SA, and NS recommend important points for conducting the laboratory test. NS, FS, and SB (contributors) helped writing the final version of manuscript.
Funding Our work has been supported by training grants from Tarbiat Modares University.
Conflicts of interest None.
Ethics approval None.
References
- Kaplan RN, Psaila B, Lyden D. Niche-to-niche migration of bone-marrow-derived cells. Trends Mol Med. 2007;13(2):72–81.
- Hoggatt J, Pelus LM. Many mechanisms mediating mobilization: an alliterative review. Curr Opin Hematol. 2011;18(4):231.
- To LB, Levesque J, Herbert KE. How I treat patients who mobilize hematopoietic stem cells poorly. Blood 2011;118(17):4530–40.
- Körbling M, Anderlini P. Peripheral blood stem cell versus bone marrow allotransplantation: does the source of hematopoietic stem cells matter? Blood 2001;98(10):2900–08.
- Winkler I, Pettit A, Raggatt L, Jacobsen R, Forristal C, Barbier V, et al. Hematopoietic stem cell mobilizing agents G-CSF, cyclophosphamide or AMD3100 have distinct mechanisms of action on bone marrow HSC niches and bone formation. Leukemia 2012;26(7):1594–601.
- Hattori K, Heissig B, Rafii S. The regulation of hematopoietic stem cell progenitor mobilization by chemokines SDF-1. Leuk Lymphoma 2003;44:575–82.
- Kucia M, Jankowski K, Reca R, Wysoczynski M, Bandura L, Allendorf DJ, et al. CXCR4–SDF-1 signalling, locomotion, chemotaxis and adhesion. J Mol Histol. 2004;35(3):233–45.
- Lataillade JJ, Domenech J, Le Bousse-Kerdiles MC. Stromal cell-derived factor-1 (SDF-1)/CXCR4 couple plays multiple roles on haematopoietic progenitors at the border between the old cytokine and new chemokine worlds: survival, cell cycling and trafficking. Eur Cytokine Netw. 2004;15:177–88.
- Foudi A, Jarrier P, Zhang Y, Wittner M, Geay J-F, Lecluse Y, et al. Reduced retention of radioprotective hematopoietic cells within the bone marrow microenvironment in CXCR4–/–chimeric mice. Blood 2006;107(6):2243–51.
- Greenbaum A, Hsu Y-MS, Day RB, Schuettpelz LG, Christopher MJ, Borgerding JN, et al. CXCL12 in early mesenchymal progenitors is required for haematopoietic stem-cell maintenance. Nature 2013;495(7440):227–30.
- Dar A, Schajnovitz A, Lapid K, Kalinkovich A, Itkin T, Ludin A, et al. Rapid mobilization of hematopoietic progenitors by AMD3100 and catecholamines is mediated by CXCR4-dependent SDF-1 release from bone marrow stromal cells. Leukemia 2011;25(8):1286–96.
- Petit I, Szyper-Kravitz M, Nagler A, Lahav M, Peled A, Habler L, et al. G-CSF induces stem cell mobilization by decreasing bone marrow SDF-1 and up-regulating CXCR4. Nat Immunol. 2002;3(7):687–94.
- Katayama Y, Battista M, Kao WM, Hidalgo A, Peired AJ, Thomas SA, et al. Signals from the sympathetic nervous system regulate hematopoietic stem cell egress from bone marrow. Cell 2006;124(2):407–21.
- Spiegel A, Shivtiel S, Kalinkovich A, Ludin A. Catecholaminergic neurotransmitters regulate migration and repopulation of immature human CD34+ cells through Wnt signaling. Nat Immunol. 2008;10:1123–31.
- Hajifathali A, Saba F, Atashi A, Soleimani M, Mortaz E, Rasekhi M. The role of catecholamines in mesenchymal stem cell fate. Cell Tissue Res. 2014;358(3):651–65.
- Carlson SL, Beiting DJ, Kiani CA, Abell KM, McGillis JP. Catecholamines decrease lymphocyte adhesion to cytokine-activated endothelial cells. Brain Behav Immun. 1996;10(1):55–67.
- Yang EV, Sood AK, Chen M, Li Y, Eubank TD, Marsh CB, et al. Norepinephrine up-regulates the expression of vascular endothelial growth factor, matrix metalloproteinase (MMP)-2, and MMP-9 in nasopharyngeal carcinoma tumor cells. Cancer Res. 2006;66(21):10357–64.
- Yang EV, Sood AK, Chen M, Li Y, Eubank TD, Marsh CB, et al. Norepinephrine up-regulates the expression of vascular endothelial growth factor, matrix metalloproteinase (MMP)-2, and MMP-9 in nasopharyngeal carcinoma tumor cells. Cancer Res. 2006;66(21):10357–64.
- Schneider A, Krüger C, Steigleder T, Weber D, Pitzer C, Laage R, et al. The hematopoietic factor G-CSF is a neuronal ligand that counteracts programmed cell death and drives neurogenesis. J Clin Investig. 2005;115(8):2083–98.
- Borger P, Hoekstra Y, Esselink MT, Postma DS, Zaagsma J, Vellenga E, et al. β-Adrenoceptor-mediated inhibition of IFN-γ, IL-3, and GM-CSF mRNA accumulation in activated human T lymphocytes is solely mediated by the β2-adrenoceptor subtype. Am J Respir Cell Mol Biol. 1998;19(3):400–7.
- Kavelaars A, van de Pol M, Zijlstra J, Heijnen CJ. β2-Adrenergic activation enhances interleukin-8 production by human monocytes. J Neuroimmunol. 1997;77(2):211–6.
- Sun X, Ng YC. Effects of norepinephrine on expression of IGF-1/IGF-1R and SERCA2 in rat heart. Cardiovasc Res. 1998;37(1):202–9.
- Robertson D, Krantz SB, Biaggioni I. The anemia of microgravity and recumbency: role of sympathetic neural control of erythropoietin production. Acta Astronaut. 1994;33(0):137–41.
- Sierra MDLL, Gasperini P, McCormick PJ, Zhu J, Tosato G. Transcription factor Gfi-1 induced by G-CSF is a negative regulator of CXCR4 in myeloid cells. Blood 2007;110(7):2276–85.
- Méndez-Ferrer S, Lucas D, Battista M, Frenette PS. Haematopoietic stem cell release is regulated by circadian oscillations. Nature 2008;452:442–7.
- Mignini F, Streccioni V, Amenta F. Autonomic innervation of immune organs and neuroimmune modulation. Auton Autacoid Pharmacol. 2003;23(1):1–25.
- Méndez-Ferrer S, Battista M, Frenette PS. Cooperation of β2- and β3-adrenergic receptors in hematopoietic progenitor cell mobilization. Skelet Biol Med. 2010;1192:139–44.
- Lapid K, Vagima Y, Kollet O, Lapidot T. Egress and mobilization of hematopoietic stem and progenitor cells. In: StemBook. Cambridge (MA): Harvard Stem Cell Institute; 2009.
- Nance DM, Sanders VM. Autonomic innervation and regulation of the immune system (1987–2007). Brain Behav Immun. 2007;21(6):736–45.
- Nadri S, Soleimani M, Mobarra Z, Amini S. Expression of dopamine-associated genes on conjunctiva stromal-derived human mesenchymal stem cells. Biochem Biophys Res Commun. 2008;377(2):423–31.
- Kalinkovich A, Spiegel A, Shivtiel S, Kollet O, Jordaney N, Piacibello W, et al.. Blood-forming stem cells are nervous: direct and indirect regulation of immature human CD34+ cells by the nervous system. Brain Behav Immun. 2009;23(8):1059–65.
- Semerad CL, Christopher MJ, Liu F, Short B, Simmons PJ. G-CSF potently inhibits osteoblast activity and CXCL12 mRNA expression in the bone marrow. Blood 2005;106(9):3020–27.
- Saba F, Soleimani M, Atashi A, Mortaz E, Shahjahani M, Roshandel E, et al.. The role of the nervous system in hematopoietic stem cell mobilization. Lab Hematol. 2013;19(3):8–16.
- Abraham M, Biyder K, Begin M, Wald H, Weiss ID, Galun E, et al.. Enhanced unique pattern of hematopoietic cell mobilization induced by the CXCR4 antagonist 4F-benzoyl-TN14003. Stem Cells 2007;25(9):2158–66.
- Can A. Haematopoietic stem cells niches: interrelations between structure and function. Transfus Apher Sci. 2008;38(3):261–8.