Abstract
The present work critically reviews the scientific and patent literature on low melting bismuth based oxide glass frits in materials for electronics, sensors and related applications such as sealing glasses, solar cells, architectural and automotive glass, the main motivation being to replace lead based materials by environmentally more benign ones. Due to similar glass forming properties of Bi and Pb, Bi based glasses are the closest ‘drop-in’ alternative for lead bearing formulations, and are therefore actually replacing them in many applications, helped also by previous experience with Bi containing materials in thick film technology and component metallisations. The outstanding issues are discussed, e.g. matching the lowest processing temperatures achieved by the classical lead based glasses without sacrificing durability and stability, as well as stability versus chemical reduction. Finally, consideration is also given to special ‘heavy’ glasses (often containing Bi and Pb together) that are useful in fields such as optics, superconductors and nuclear technology, as well as to specific Bi2O3 containing crystalline compounds.
Introduction
Low melting glasses in electronics and other applications
As for ceramics, inorganic glasses, glass–ceramic and glaze materials have long gone beyond their traditional uses to address a wide array of modern technological challenges,Citation2–Citation43 in fields such as electrical engineering/electronics/sensors,Citation9–Citation32 solar energy,Citation42,Citation43 protective and decorative coatings,Citation20,Citation27–Citation35 optics/optical telecommunications,Citation36,Citation37 structural mechanics,Citation8 medical,Citation38 nuclear technology,Citation6,Citation7 superconductorsCitation39 and microfluidics.Citation40,Citation41
Owing to performance and cost criteria, most standard glasses have relatively high softening points. However, there are many technological applications where a low softening temperature is required, in order to lower energy expenditure, avoid damaging devices in contact with the glass during processing or ensure compatibility with other materials:
i. | hermetic sealing of packages, lamps, electrical feedthroughs and semiconductor devicesCitation13,Citation14,Citation16,Citation17,Citation19,Citation44,Citation45 | ||||
ii. | hermetic sealing and mechanical attachment of sensorsCitation23,Citation27 () | ||||
iii. | encapsulation of semiconductor devicesCitation29,Citation30 | ||||
iv. | overglazing of automotive, packaging and architectural glassCitation33,Citation34,Citation46–Citation48 | ||||
v. | photovoltaic (PV) solar cell technology – conductors and contactsCitation42,Citation43,Citation49–Citation53 | ||||
vi. | enamelling of aluminium in architecture and home appliancesCitation35,Citation54–Citation58 | ||||
vii. | thick film (TF) electronics and other devicesCitation21,Citation22,Citation24,Citation25,Citation27,Citation59 on various substrates:Citation60 glasses for TF resistor (TFR),Citation61,Citation62 conductor,Citation63,Citation64 overglaze, dielectricCitation65 and sealingCitation15–Citation19 materials (, the section on ‘PbO in low melting frits and TF technology’); especially, special low firing compositions for fabrication of circuits and sensors on glass or metals.Citation1,Citation28,Citation66–Citation72 |
Figure 1. Example TF circuit, piezoresistive pressure sensorCitation27, showing typical involved materials: reddish tint added to sealing glass to enhance visibility; ‘conductive glass’ seal = low firing TFR composition
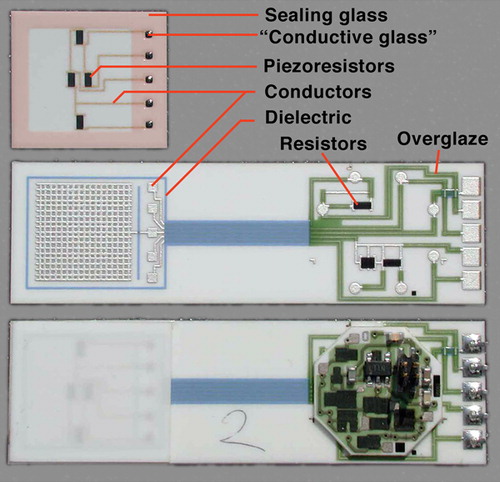
For these applications, glasses are often formulated as frits (e.g. finely divided powder), which may be applied, dispersed in a suitable medium, onto a substrate by various methods such as slip casting, screen printing, roller/curtain coating, spraying, dispensing and electrophoresis, or as preforms for sealing. Classically, the aforementioned applications have to a great extent used lead based glasses, which have a rather unique combination of desirable properties,Citation10–Citation12 as will be discussed hereafter in the section on ‘PbO in low melting frits and TF technology’. compiles the compositions and melting points/processing temperatures of selected classical low melting lead based glasses.
Table 1. Representative compositions (cation-%) of low melting lead based glasses [Temperatures = melting points (eutectics) or processing temperatures (others)]
shows a TF integrated pressure sensorCitation27 that illustrates many of the aforementioned applications: hermetic sealing of the sensing membrane combined with mechanical attachment and electrical contact, encapsulation through a hermetic dielectric of a wetted surface, conductors, resistors and overglazes.
While most low melting glasses are used on a substrate or for sealing, there are several significant ‘bulk’ applications of low melting or relatively low melting glasses:
i. | lead ‘crystal’ glassCitation89 | ||||
ii. | glasses for nuclear waste immobilisationCitation6,Citation95–Citation98 | ||||
iii. | leaded CRT tube glassCitation89 | ||||
iv. | superconductor synthesis, bulk or film, via the glass–ceramic routeCitation39,Citation99–Citation105 | ||||
v. | heavy metal oxide (HMO) glasses with high refraction indices and far infrared (IR) transmission for optical devices and communicationsCitation36,Citation106–Citation128 | ||||
vi. | HMO glasses for gamma radiation shieldingCitation129–Citation135 |
Main performance criteria
The performance criteria in selecting a low melting glass depend on the application. A short overview is given in (layers and sealing) and (bulk) for the aforementioned applications. In contrast to most ‘film’ applications, most of the ‘bulk’ examples listed above depend specifically on the presence in the glass of HMOs, rather than just require a reliable low melting glass. In optics, heavy, polarisable cations such as Pb2+ and Bi3+ impart to the glass a high diffraction index, a high dispersion, strong nonlinear effects and better IR transmission,Citation12,Citation36,Citation112,whereas CRT and gamma radiation shielding benefits from the strong absorption of ionising radiation by heavy elements.Citation11,Citation129,Citation130
Table 2. Requirements for (relatively) low melting glasses for layers and seals (‘−’ = normally not important; ‘+’ = significant; ‘++’ = critical)
Table 3. Requirements for low melting ‘bulk’ glasses (‘−’ = normally not important; ‘+’ = significant; ‘++’ = critical)
PbO in low melting frits and TF technology
Thick film electronics makes wide use of glassy compounds, used as main components of overglazes, permanent binders for dielectrics and resistors, and also as frits/adhesion promoters for conductors.Citation21,Citation22,Citation24,Citation59,Citation61–Citation65 Note that the technology and materials are very similar for other applications such as architectural/automotive/solar cell overglazes and conductors.
For conductors, resistors and overglazes (and relatively old dielectrics), most classical low melting frits are based on the PbO–B2O3 (lead borate) system, with mainly SiO2, ZnO and Al2O3 additions. Several phase diagrams and property maps exist for these systems.Citation21,Citation,91–Citation93,Citation136–Citation144
gives several representative ‘traditional’ glass compositions, compared with that of traditional leaded ‘crystal’ glassCitation89 and some representative eutectic compositions in the phase diagrams. Throughout this work, compositions are given on a cation basis unless specified otherwise, as by Dumbaugh and Lapp;Citation36 this convention facilitates comparison with Bi2O3 based glasses when PbO is replaced by approximately equimolar amounts of ‘BiO1·5’.
By altering the composition, the properties, especially the processing temperature and the tendency to crystallise, can be easily and reliably tuned. Low melting glasses in this system, which have a composition relatively close to the PbO–B2O3 binary eutectic, allow a reduction in binder glass amount in TF conductors, and/or a decrease of processing temperatures down to ∼400°C for low temperature TF conductors, dielectrics, overglazes and resistors,Citation67,Citation72,Citation85,Citation86 for glass sealing (‘solder glasses’) of cathode ray tubes (CRTs) and flat panel displays (FPDs), or for glass encapsulation of semiconductor devices.Citation15,Citation16,Citation73,Citation74
Low melting glasses in the lead zinc borosilicate system can be formulated as essentially ‘stable’, e.g. with little or no crystallisation during firing or sealing, or devitrifying, e.g. forming significant amounts of crystalline phase and thus conserving dimensional stability upon later reheating. These latter crystallising glasses are referred to as glass–ceramics, vitroceramics, or ‘cements’ in glass sealing parlance. In these compositions, devitrification is usually favoured by high amounts of ZnO, whereas B2O3, SiO2 and Al2O3 tend to stabilise the glassy state (). To achieve even lower processing temperatures and/or promote wetting, compounds such as CuO, Fe2O3, Bi2O3, V2O5, WO3, MoO3 and fluorine (batched as CaF2, PbF2, ZnF2, BiF3, etc.) can be added to the glass formulation.Citation19,75,145Citation75,Citation145–Citation152 Interestingly, fluorine, which is effective in lowering the processing temperature, was found to have better compatibility with glasses where a sizeable amount of PbO was replaced by Bi2O3.Citation149 Very low processing temperatures may be reached by glasses largely based on PbO–TeO2,Citation45 PbO–V2O5Citation145,Citation147,Citation148 and especially SnO–SnF2–PbO–PbF2–P2O5.Citation153
Glass frits are often used in conjunction with other materials that act as fillers (): insulating powders for dielectrics/overglazes/encapsulation/sealing glasses,Citation18,Citation67,Citation145,Citation147,Citation149–Citation152,Citation154 conductive oxides for resistors,Citation61,Citation62,Citation155–Citation157 metal powders and adhesion promoters for conductors,Citation63,Citation64,Citation88,Citation158–Citation160 pigments, etc. Even for applications such as sealing, encapsulation or TF overglazes, where they are not intrinsically required, fillers are often found necessary or advantageous in practice, mainly to adjust the coefficient of thermal expansion (CTE) of the deposited material to that of the substrate(s) (see Donald’s reviewCitation20 for an extensive list of filler CTEs). The filler can also be used as a nucleating agent to better control the crystallisation process of a devitrifying glass. Alternatively or additionally, chemical and mechanical stabilisation of a glass can be obtained by reaction with the filler; an example is the reaction of lead bearing glass with TiO2 and MoO3,Citation161,Citation162 yielding both an increase of the filler volume (by formation of PbTiO3/PbMoO4) and of the glass softening point (by the resulting depletion of glass PbO content).
Table 4. Representative materials/fillers used in conjunction with glass frits
Correctly formulated, both stable and devitrifying lead based glasses achieve an excellent combination of very consistent and reliable properties, relatively large processing windows, acceptable corrosion resistance and low processing temperatures, all this without requiring, in their composition, alkaline oxides, which are detrimental for insulating properties (due to the mobility of alkaline ions under electric fields) and chemical durability, and impart a high CTE that is deleterious in most cases (except for substrates with CTE greater than ∼10 ppm K−1). Owing to these advantages, which have been recognised for a long time (see the section on ‘Lead and bismuth in glass: a historical perspective’ in Supplementary Material 1 http://dx.doi.org/10.1179/1743280412Y.0000000010.S1), these lead based glasses have achieved widespread use, and have been the object of extensive studies and reviews.Citation10–Citation12
An overview of the current status of commercial TF compositions is given in ; modern multilayer dielectric compositions such as ESL 4913 are commonly lead free,Citation163 and recently introduced (relatively) low melting overglaze materials use Bi2O3 instead of PbO. Surprisingly, even an old composition such as Ag/Pd conductor DP 9473 uses a Bi2O3 based glass.Citation164
Table 5. Qualitative composition (+++ = high, ++ = medium, + = low, ? = very low or absent) of commercial TF inks (Tf = firing temperature): dielectricsCitation167 (compared with LTCC),Citation168–Citation170 conductorCitation164 and resistor.Citation85 Boron most likely present in all these compositions, but not always detectable by the analysis methods – mentioned where explicitly formulated/detected
The trend away from lead
In recent times, there is a trend towards removing lead from electronic materials due to its toxicity (see the section on ‘Toxicity of elements in glasses’ in Supplementary Material 1 http://dx.doi.org/10.1179/1743280412Y.0000000010.S1), a move spurred by the enactment of the European Union RoHS (Restriction of Hazardous Substances) directive.Citation171 This has already largely taken place in the field of metallic solders, where the Sn–Ag–Cu alloy (‘SAC’) has become the standard to replace the classical Sn–Pb–(Ag) eutectic.Citation172 Although glasses in electronics are mentioned under the list of exemptions,Citation173 the directive requires this list to be periodically reviewed in the future, and further restrictions on the use of lead bearing glasses are therefore likely in the medium term. Moreover, cadmium, also a popular addition to low melting glass frits, must be abandoned.
In contrast to the case of metallic lead and its simple, relatively soluble compounds such as litharge and massicot (PbO), minium (Pb3O4) and ceruse (2PbCO3.Pb(OH)2), lead in well prepared glasses and glazes was traditionally considered to be stably bound, and therefore not a health concern, provided the composition was well formulated, and, for glazes, sufficiently fired.Citation174 This perception drastically changed after the widely publicised 1991 work of Graziano and Blum,Citation175 who demonstrated that important amounts of lead could be leached out of ‘crystal’ glass over time by (acidic) wines and spirits. This alarming report was later somewhat contradicted by more realistic tests,Citation176 while other researchCitation177 showed that water dredged from Pb contaminated sediments remained well within the official limits, and, more to our point, that lead bearing low temperature co-fired ceramic (LTCC) compositions could even exhibit good biocompatibility.Citation178,Citation179 However, although human exposure to lead and corresponding blood levels have drastically dropped in recent times, the ongoing controversy over the effects of low lead levels, especially for children,Citation89 will likely generate additional regulatory pressure on its uses (see the section on ‘Toxicity of elements in glasses’ in Supplementary Material 1 http://dx.doi.org/10.1179/1743280412Y.0000000010.S1). In the case of ‘crystal’ glass, this has led to research activity towards lead free substitutes,Citation90 which showed that most of the properties of original ‘crystal’ could be largely duplicated (although the working range was somewhat smaller), while guaranteeing minimal leaching of potentially dangerous substances.
The situation of glasses in electronics might seem less critical, due to the smaller volumes involved and to the fact that contact with foodstuffs or beverages is (usually) not specified. However, electronic glasses often require lower processing temperatures and only little or no alkali ions are tolerable when good insulating properties are required. Therefore, they can contain much higher amounts of lead than ‘crystal’ (⩾24 mass-%): ∼65% for classical 850°C firing TFRsCitation62 and up to ∼85 mass-% for sealing glassesCitation12,Citation15,Citation16,Citation73,Citation74,Citation150 and low firing TFRsCitation85,Citation86 (). This results in much lower stability against dissolution in acids,Citation140,Citation141 which again raises the issue of contamination of groundwater from disposed electronics waste. Moreover, very low temperature electronic encapsulant and sealing glasses may contain even more dangerous metals such as Cd and Tl.Citation165 Therefore, especially for these applications, alternative materials are needed. Finally, even if the final product may be considered stable, occupational exposure during processing is always a concern.
In TF electronics, removal of lead started in the 1980s with multilayer dielectrics, where traditional ceramic filled glass formulations have given way to crystallisable types, which can be formulated lead free.Citation163 More recently, there has been an effort to remove lead from frits in conductors,Citation180 overglazesCitation181 and sealing glasses,Citation182 and commercial lead free compositions have become widely available (see ). However, resistors (and to some extent sealing glasses and overglazes) have lagged behind in this trend, due to the exceptionally easy processing of lead based glasses and the considerable development work required for entirely new TFR series. Lead free glasses were widely used in the 1970s to 1980s, including for resistors, due to the then considerable development of nitrogen firing TF systems.Citation183–Citation189 However, these materials have largely fallen into disfavour, due mainly to performance and reliability problems, especially in ensuring proper organic vehicle burnout.Citation190
While ‘lead free’ is an important aspect in the present review, glasses containing both Bi2O3 and PbO are also included, as they are relevant for specialised applications.
Low melting frits: alternative systems
After the ‘classical’ lead based glasses (see previous sections), a short discussion of the potential oxide glass substitutes based on elements other than bismuth is given in this section. The reader is referred to other reviews for halide, chalcogenide (non-oxide) and chalcohalide glasses.Citation37,Citation191–Citation194
Borate/borosilicate/silicate glasses
Several glass systems have been proposed to replace lead bearing frits. In the case of multilayer dielectrics for ‘standard’ (firing at 850–900°C) TF technology, crystallising glasses containing mainly CaO–Al2O3–B2O3–SiO2, forming phases such as anorthite or celsian, have largely displaced lead bearing types in both screen printed 850°C firing multilayer dielectricsCitation163 and LTCC,Citation9,Citation168,Citation169 with mostly improved performance, and therefore provide a satisfactory solution.
A complete lead free cofireable TFR+LTCC system for processing at 900°C has been implemented by Bosch in its production of car engine control units (ECUs).Citation169 This system is based on two glasses: an anorthite crystallising CaO–Al2O3–B2O3–SiO2 glass (as in the case of dielectrics),Citation163 and a lower melting Na2O–B2O3–SiO2 one, which probably acts as a binder. In spite of its good properties, its extension to a general purpose TFR system would be unlikely, as the complicated reactions involved in its processing require a very rigid and tightly controlled manufacturing process: as the resistor has a higher CTE than the substrate, it has to be co-fired with its overglaze, which imparts a protective compressive stress. Such very standardised processes may probably also be used by the chip resistor manufacturers to produce lead free components.
There have been some attempts at making general purpose TFRs based on similar glasses, with RuO2Citation195–Citation198 and ruthenate perovskites,Citation197,Citation199–Citation202 or pyrochloresCitation82,Citation203 as conducting phases, which have partly resulted in promising properties, albeit with problems of high process sensitivity and the requirement of a large amount of, expensive, conducting RuO2.
The high encountered process sensitivity is expected, as these glasses tend to be not so ‘well behaved’Citation29,Citation198,Citation204–Citation207 as lead based ones, which may be formulated to be virtually non-crystallising;Citation45,Citation73 the glass forming range and stability of the lead free glasses is in general more limited, and the processing range is restricted to relatively higher temperature applications than for lead bearing ones, or other properties such as CTE matching and durability are compromised. Therefore, the abovementioned lead free glasses are not applicable to compositions requiring very low processing temperatures in applications such as low firing TFR overglazes and sealing glasses in flat screens.Citation206,Citation207
Nevertheless, silicate, borosilicate or borophosphate glasses have found large scale low temperature applications such as the overglazing of architectural and automobile glass,Citation34,Citation46,Citation47 and enamelling of aluminium.Citation35,Citation47,Citation54–Citation57,Citation208 In these applications, the processing window between sufficient melting of the glass and degradation of the substrate is narrow, and firing schedules are tightly controlled, so a very wide stability range against crystallisation is not necessary. Moreover, significant amounts of alkali oxides, which are detrimental for insulator dielectric applications, are tolerated within the limits set by their detrimental effect on corrosion resistance; in enamels for aluminium, they impart a desirable high CTE to the glass.
Phosphate glasses
Phosphate glassesCitation209,Citation210 are an interesting alternative, as they usually have low working temperatures. On the other hand, high CTE and water absorption are potential issues. An example low melting system is Na2O–Cu2O–CuO–P2O5,Citation211 but chemical durability is only passable and it contains a high alkali content, limiting its use in electronics.
Many promising phosphate glasses are based on/derived from the ZnO P2O5 system, with additives such as B2O3, SiO2, MgO, CaO, Al2O3, Fe2O3, V2O5 and Nb2O5.Citation97,Citation212–Citation216 For instance, Nb2O5 additions were claimed to allow sealing glasses with processing temperatures as low as 500°C, while retaining good durability and moderate CTE values.Citation216 Explorative TFRs have also been formulated with such glasses, yielding, however, compatibility problems with Ag terminations.Citation214,Citation216 As in borosilicates, a good combination of low processing temperature, stability and durability is imparted by PbO, and corresponding lead iron phosphate glasses have drawn interest for vitrification of high level radioactive waste; avoidance of PbO is possible for this application if somewhat higher processing temperatures can be accepted.Citation6,Citation95,Citation97,Citation98
Glasses based on divalent tin
A major breakthrough towards low melting phosphate frits was achieved with the SnO–ZnO–P2O5 system.Citation182,Citation217 SnO, with Sn in the unusual +2 oxidation state, seems to behave in a similar manner as PbO, without the toxicity problems. In fact, comparing simple binary SnO, PbO and ZnO phosphate glasses, SnO gives the lowest glass transition temperatures, in the order SnO<PbO<ZnO.Citation218,Citation219 Thus, SnO–ZnO–P2O5 glasses (with more SnO than ZnO) can achieve flow characteristics similar to those of traditional lead based frits,Citation182 while remaining lead and alkali free and having acceptable chemical durability. A recent reviewCitation219 of SnO based glasses shows that low melting properties are also found in tin(II) borate and silicate glasses, and, like PbO, SnO allows very wide glass forming ranges with the glass forming oxides, because it can partly behave as a glass former at high concentrations. Substituting part of the O2− anions by F− or Cl−,Citation220,Citation221 can further reduce processing temperatures (usually at the expense of durability, greatly improved by additions of none other than Pb),Citation153 while posing less migration problems than the alkali ions often present in other low melting glass compositions.
Although these glasses seem very promising, there are issues about their rather large thermal expansion,Citation182 mediocre adhesion to silicates such as float glassCitation222 and mechanical properties.Citation150 Moreover, the +2 valence state of Sn, which is not stable in ambient air, raises two important processing issues. First, processing in air is preferable (cost and burnout of the organic vehicle), but can oxidise Sn2+ to Sn4+, leading to devitrification and halting densification. This issue can be solved by replacing some of the SnO with low valence oxides of transition metals such as Mn, Co and Fe, which would protect Sn2+ by acting as buffers that stabilise the oxygen activity in the glass to low values while being preferentially oxidised, as has been patented for Mn.Citation223 This, however, raises the second issue: such glasses, once they achieve densification, have a reducing character for anything they encapsulate, as evidenced by the tendency of Cu ions to be reduced to metal.Citation219 Although this opens up interesting applications such as base metal TFs, compatibility with some applications such as existing RuO2/ruthenate based TFRs will be problematic, due to likely reduction of the Ru compounds to metal (2SnO+RuO2→2SnO2+Ru). Finally, the presence of metals in several coexisting valence states can degrade the insulating characteristics of dielectrics based on these glasses.Citation224–Citation228 One interesting open point relevant for this work is the possible substitution of Pb by Bi as an additive to achieve water durable ultra low melting tin fluorophosphate glasses,Citation153,Citation220 i.e. whether Bi oxifluoride in glass is first at all thermodynamically compatible with Sn2+ (not reduced to metal) and, if this is the case, yields similar improvements in durability as Pb while maintaining a low processing temperature.
Other systems
Finally, other more ‘exotic’ systems must be mentioned, such as glasses containing important amounts of TeO2, V2O5, Nb2O5, Ta2O5, MoO3 and WO3, with TeO2, V2O5 and MoO3 giving especially low melting compositions.Citation45,Citation73,Citation113,Citation120,Citation123,Citation126,Citation229–Citation241 Although toxicity of V2O5 is a cause of concern (the section on ‘Toxicity of elements in glasses’ in Supplementary Material 1 http://dx.doi.org/10.1179/1743280412Y.0000000010.S1), these oxides are useful as additives in small amounts, to improve adhesion, wetting and durability, suppress crystallisation in glasses and reduce working temperatures.Citation35,Citation56–Citation58,Citation146,Citation198,Citation207,Citation216,Citation222,Citation242
Discussion
From the above considerations, one can conclude that replacement of lead based frits by the abovementioned systems, of which several examples are summarised in , may be achieved for applications not requiring a too demanding combination of good insulating properties (e.g. alkali free), wide processing window, high durability and low processing temperatures; enamelling/overglazing aluminium and glass for protective, functional and decorative purposes, as well as TF dielectric and LTCC compositions, are good examples of successful large scale replacement of lead bearing glasses by borosilicate/silicate compositions. However, durability is often problematic if low processing temperatures are specified.Citation33
Table 6. Some low melting lead free glass systems (without Bi), with typical glass transition temperature Tg [R2O = (Li,Na,K)2O; RO = (Ca,Sr,Ba,Zn)O]
In electronics, mass produced chip resistors and co-fired LTCC devices including resistors may also be manufactured lead free using similar glasses. However, it would be difficult to achieve a general purpose TF system with a comfortable processing window using these materials.
Phosphate and SnO based glasses, especially those derived from the SnO–ZnO–P2O5 system modified with transition metal oxides, are very promising, and their flow characteristics can resemble those of lead based frits, but they represent a very radical departure from the heretofore applied chemistry, especially due to their intrinsically reducing character. This may lead, through the likely resulting presence of mixed valence transition metal oxides, to degradation of the insulating properties of dielectrics. Also, TFRs, currently based on (most likely incompatible) RuO2 would have to be formulated anew, using compatible conductive phases based on compounds such as reduced/doped SnO2, Fe3O4, MoO2 and WO2. Finally, the high water affinity of phosphate glassesCitation209 is an issue which cannot be ignored if well defined, high reliability electronic materials are to be manufactured.
Bismuth glasses
Introduction
In contrast to the abovementioned lead free glasses, Bi2O3 appears a quite promising ‘drop in’ replacement for PbO, as also evidenced by comparing the commercial lead free and lead based TF overglazes (). The intentional use of bismuth in glasses is by far not as old as that of lead (the section on ‘Lead and bismuth in glass: a historical perspective’ in Supplementary Material 1 http://dx.doi.org/10.1179/1743280412Y.0000000010.S1), but the similarity of Bi2O3 and PbO was immediately noticed in the early studies;Citation129,Citation244–Citation247 akin to PbO, Bi2O3 belongs to the class of ‘conditional glass formers’: while it does not by itself readily form a glass, it can be incorporated in very large quantities in the classical glass forming oxides SiO2, B2O3 and P2O5 and GeO2,Citation2,Citation110,Citation245,Citation246,Citation248 where it acts as a glass modifier at low concentrations, but partly as a glass former at higher ones. These glasses may in turn incorporate, under standard glassmaking conditions, large amounts of alkaline earth (especially SrO and BaO) and transition metal oxides (e.g. ZnO, Fe2O3, CuOy, MnOy, CoOy), as well as PbO, with small additions of enhancing vitrification.Citation73,Citation122,Citation129,Citation246,Citation248–Citation272 Other possible additives are alkaliesCitation247,Citation248 and rare earths.Citation273–Citation278 Vitrification in different systems is detailed more fully in the following section. Representative compositions are given in , and a system property reference index of studied systems is given in for borates, for silicates, germanates and phosphates, for other systems and for binary systems without network formers; systems with several network formers are attributed on a following priority basis: B2O3, SiO2, GeO2, TeO2, V2O5 and MoO3.
Table 7. Bismuth glass compositions, in cation mole percentage
Table 8. System property index: borates
Table 9. System property index: silicates, germanates and phosphates
Table 10. System property index: tellurites, vanadates, molybdates and other
Table 11. Glasses without standard network formers: binary systems
One fortunate difference with lead is the much lower toxicity of bismuth, which compares well in this respect with other potential substitutes, as discussed in the section on ‘Toxicity of elements in glasses’ in Supplementary Material 1 http://dx.doi.org/10.1179/1743280412Y.0000000010.S1. A less fortunate aspect, however, is the somewhat lower fluxing ability, as can be inferred from the higher overall bonding of Bi3+ vs. Pb2+: simple substitution of PbO with ‘BiO1·5’ leads to higher processing temperatures, as illustrated by the stable liquidus () and glass transition temperatures (Tg, ). This may be seen as well on the ternary PbO–Bi2O3–B2O3 phase diagram,Citation279 where the ternary eutectic composition lies very close to the PbO–B2O3 join, at about 45Pb+4Bi+51B on a cation basis. Therefore, most studies and developed low melting glasses are based on the Bi2O3–B2O3 binary, which combines a wide vitrification range with relatively low processing temperatures, with ZnO, SiO2 and Al2O3 being the most common additions. One must, however, note that comparison on the basis of equilibrium diagrams should be made with caution, given the slow equilibration in many Bi2O3 containing systems, attributed to mesomorphism in the meltCitation280 and illustrated in corresponding metastable phase diagrams.Citation280–Citation282
Figure 2. Liquidus temperatures of binary systems, redrawn from phase diagrams Bi2O3–SiO2,Citation281 Bi2O3–GeO2 (PDC-2359), Bi2O3–B2O3 (PDC-323) and PbO–B2O3 (PDC-282)
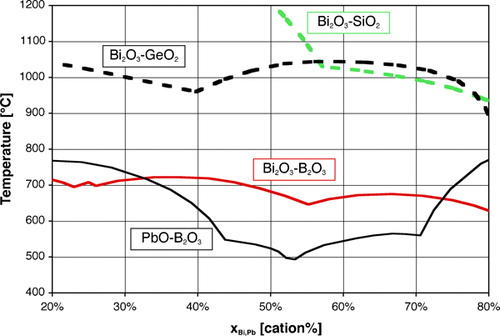
Figure 3. Glass transition temperatures Tg of binary systems according to George et al.Citation248 (heavy lines), compared with other works (×: Ref. 317; Δ: Ref. 318; +: Ref. 141; *: Ref. 319)
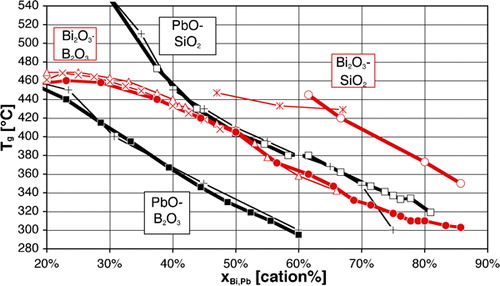
Scientific work has been matched by technical use, the first patent dating from as early as 1945.Citation283 In the early patents,Citation49,Citation87,Citation146,Citation283–Citation288 Bi2O3 was introduced in component/ceramic metallisations for its fluxing and wetting properties. The glass frits usually contained PbO and/or CdO, their elimination was at the time not an issue, and the patents gave conflicting information about how Bi2O3 should best be added to obtain maximal adhesion: included in the glass frit, ‘presintered’ with it, added separately to the paste, or even be present both in the glass and as a separate addition. Ensuring good adhesion to alumina without any alkali oxides, CdO and PbO was reported to be problematic, but possible by replacing some SiO2 by GeO2.Citation288 Starting from 1980, a string of early Soviet patents,Citation289–Citation293 from what is now the Belarusian State Technological University, disclose a family of low melting or intermediate glass frits based on the ZnO–Bi2O3–B2O3–SiO2 system, with optional Li2O, Na2O, MgO, BaO, CuO and CdO, Al2O3 additions, and claiming a better chemical resistance and a lower CTE than analogous lead borosilicate frits. These glasses (: B80/B82/B83/B89), featuring moderate to high Bi content, processing temperatures down to ∼500°C, and designed specifically for application in electronics, overglazing and sealing,Citation289–Citation294 can truly be considered as the base for the ‘modern’ Bi based frits. More recent patents disclose usually similar compositions for glazes and enamels,Citation295–Citation302 TF conductors,Citation250,Citation303–Citation305 resistorsCitation251 and overglazes,Citation306,Citation307 plasma display panel (PDP) dielectrics,Citation308 conductorsCitation305,Citation309,Citation310 and low melting sealing glasses.Citation311–Citation314
The closeness of PbO and Bi2O3 may be seen by comparing, on a cation basis, some glasses taken from (standard and low fire resistor and non-crystallising sealing compositions) with corresponding Bi based analogues (). Both types belong to the so called ‘fragile glasses’, i.e. with a strong dependence of properties on temperature around Tg.Citation253,Citation315
Bi based oxide glasses are already making strong inroads in commercial architecturalCitation33 and automotive overglazes,Citation48 as well as TF compositions (). Besides these lead free substitutes, Bi2O3 based HMO glasses have found potential applications, partly together with PbO, in nuclear physics (scintillators, γ-ray shielding windows),Citation129–Citation135 optics,Citation36,Citation106–Citation109,Citation111–Citation128 magnetic materialsCitation316 and glass–ceramic+ semi/superconductors.Citation99–Citation102 However, in spite of their significance, compositions based on Bi2O3 have drawn only scant attention, if mentioned at all, in classical ‘mainstream’ reviews of glasses.Citation2–Citation6,Citation20
Glass formation
A comparison of the vitrification ranges of Bi2O3 and PbO (and a few SnO examples) with common and uncommon glass formers, as found by various authors, is given in for nominally binary systems, as a function of the estimated rate of cooling from the melt. This rate, indicated as a subscript for each limiting composition, is expressed in this work as a ‘quenching index’ Q, equal to the base 10 logarithm of the estimated cooling rate (K s−1):The reader is reminded that the indicated cooling rates are approximate at best, educated guesses at the worst; the method for estimating/determining Q is discussed in the section on ‘Estimation of cooling rates’ in Supplementary Material 1 http://dx.doi.org/10.1179/1743280412Y.0000000010.S1. Please also refer to the section on ‘Sealing and glass stability during reflow’ for stability upon reheating, and to more extensive work on PbO basedCitation10–Citation13,Citation16,Citation19 and SnO basedCitation219 glasses.
Origins of discrepancies in indicated data
As seen in , some values are clearly in conflict, as exemplified by studies on air cooled gram size samplesCitation245 yielding a larger vitrification range than others on quenched ones.Citation244 Partial volatilisation of some components, especially PbO, Bi2O3, B2O3 and P2O5, can account for some of these discrepancies, especially for quenching studies, which tend to involve small, open melts. Also, the large apparent discrepancy involving the extensive early work of Janakirama–RaoCitation246 is tentatively attributed to the graphical representation; if Bi2O3 is taken as ‘BiO1·5’ (to make it comparable to the other oxides), a convention sometimes seen in the literatureCitation36,Citation112 and used in the present review, their results become closer to that of other work.
This said, the by far most common cause of extended reported vitrification ranges can be traced to small but significant amounts of SiO2, Al2O3 and other impurities (in porcelain, fireclay, etc.) leached from crucibles,Citation118,Citation318 so some of the examined compositions are most likely not strictly binary. Therefore, the borate systems are marked in by a crucible specific suffix (where specified) after the quenching index.
Binary systems
The binary Bi2O3–B2O3 system has been studied most extensively, and vitrifies easily at low cooling rates.Citation317 A minimal amount of Bi2O3 is seen to be necessary due to the miscibility gap in the phase diagramCitation110 (which also exists with PbO–Bi2O3Citation136 and many other borates), setting a practical limitation for technical purposes to above ∼19%Bi2O3, the end of the gap. It is nevertheless possible to achieve apparently homogeneous vitrification throughout this range if quenching sufficiently fast from above the gap.Citation110
For the ‘strict’ Bi2O3–B2O3 binary, the extensive and well controlled work of BeckerCitation317 (very large melts, controlled cooling, noble metal crucibles, 20–43%Bi2O3) is deemed the most reliable for slow cooling. At intermediate cooling rates, the maximum Bi content is ∼60%,Citation118,Citation244,Citation318 with 66% achievable for splat quenching.Citation318 Going to twin roller quenching increases the vitrification range further, to 0–88%Bi.Citation110,Citation248
The Bi2O3 rich ends of the glass forming ranges with B2O3 and SiO2 are often reported to be quite different from each other, and also from the values for PbO. However, as noticed by Dumbaugh and Lapp,Citation36 this is due to the arbitrary selection of the ‘molecules’ PbO, Bi2O3, B2O3 and SiO2; on a cation basis, these limits (Pb versus Bi and B versus Si) become more similar, as illustrated by the results of fast quenching experiments by Stehle, George et al.,Citation110,Citation248 where the four systems were examined in the same conditions; for Bi2O3–SiO2, a maximum of ∼85% Bi is obtained.
To summarise the data on binary systems with the common glass formers (B2O3, SiO2, P2O5, GeO2), the vitrification ranges (in cation-%) of Bi2O3 and PbO appear similar, although a direct comparison is difficult due to the spread in experimental data and the paucity of experiments under the same conditions. The maximum Bi2O3 content is smaller with GeO2, and even more with P2O5; the Bi2O3–P2O5 system has therefore attracted limited attention. The reverse is seen for SnO, where vitrification is especially favourable with P2O5, resulting in glasses based on the SnO–P2O5 system being another promising substitute to lead based ones (see the section on ‘Glasses based on divalent tin’).
With the unconventional network formers, vitrification is more difficult in general, and more severe quenching must be applied; comparing with PbO, vitrification appears to be more difficult for Bi2O3 with TeO2 and V2O5, while the reverse is true with MoO3. Under fast to very fast quenching, binary glasses may be obtained with Li2O, BaO, PbO, CuOy, MnOy and Ga2O3.
Complex systems with traditional network formers
As mentioned earlier, even small amounts of Al2O3 and especially SiO2 leached from the crucible considerably facilitate vitrification in the Bi2O3–B2O3 system. This synergistic vitrification is confirmed by experiments with B≈Si, where 74% Bi may be achieved by conventional melting and casting,Citation361 i.e. much more than in either the Bi2O3–B2O3 or Bi2O3–SiO2 binary system. This is also true for low SiO2 and Al2O3 additions: a 65BiO1·5+33BO1·5+2SiO2 composition vitrifies easily when casting large plates,Citation112 as does 70BiO1·5+25BO1·5+3SiO2+3AlO1·5 under moderate quenching.Citation324 Such modifications are therefore very useful for low melting glasses rich in Bi2O3, as they stabilise the glass with only limited impact on processing temperature.Citation314
Synergistic vitrification also occurs, for a given network former, when replacing on a cation basis part of the Bi2O3 with other alkaline earth and transition metal oxides. This occurs in spite of a generally poorer vitrification with these oxides (except PbO) taken individually than with Bi2O3, and is seen for borates with SrO,Citation246,Citation258,Citation346 BaO,Citation246,Citation258,Citation270,Citation349 PbO,Citation130,Citation246,Citation279 ZnOCitation246,Citation354,Citation355,Citation392 and CuOy.Citation249 , redrawn on a cation basis from the original studies,Citation249,Citation354 illustrates the case of the Bi2O3–B2O3 binary extended with ZnO and CuOy: there is clearly an optimum in substitution that minimises the required amount of network former. This composition ‘hump’ roughly agrees with low melting zones in the corresponding ternary equilibrium diagrams.Citation354,Citation356 In commercial low melting glasses based on ZnO–Bi2O3–B2O3 (, Hg–Nch–Usu), the Zn/Bi ratio, in practice also influenced by other considerations such as acid resistance, is commonly 0·2–0·7. In most cases, Zn is preferred over Cu due to the easy occurrence of mixed valence in the latter, leading to semiconductivity in Cu rich glassesCitation249, a drawback mainly for insulators. This said, excluding PbO and alkalis, Zn and Cu apparently give the lowest melting points in the ternary phase diagrams, with the deepest eutectics somewhat below 600°C; the BaO–Bi2O3–B2O3 system, for instance,Citation347,Citation348 apparently is fully solid at 600°C. Going one step further in complexity, mixing both network formers and modifier, allows a further decrease in the required amount of the former, as seen for PbO–ZnO–Bi2O3–B2O3–SiO2 glasses,Citation256 in line with the general trend towards more difficult crystallisation of multicomponent glasses.
Figure 4. Vitrification range in Bi2O3–B2O3–ZnOCitation354/CuOyCitation249 systems (cation basis)
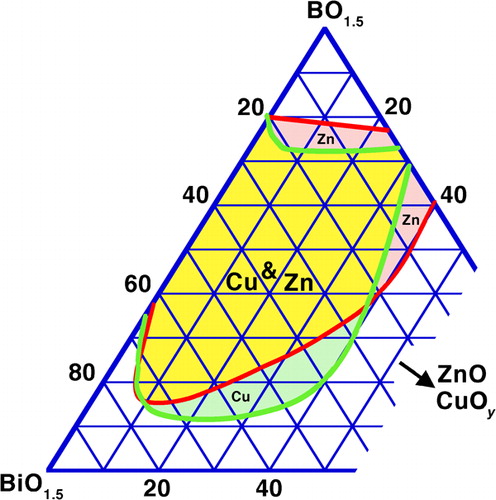
In contrast to these oxides, an extension of the glass forming range to lower B2O3 contents is not seen with the light alkali elements Li, Na and K ();Citation247 the effect for small substitutions of BiO1·5 by alkalis is neutral up to ∼25% LiO0·5, then the minimum B2O3 required for vitrification rises sharply; this threshold is much lower (∼3%) for NaO0·5 and practically zero for KO0·5. Under fast cooling in the more complex R2O–ZnO–Bi2O3–B2O3 (R = Li, Na, K) system, a deleterious effect on vitrification was also observed, with a similar, but less pronounced trend of the size of the vitrification domain (Li>Na>K).Citation392 Qualitatively, this agrees with the relatively stringent limits set on alkali content in the patents of Hasegawa et al.,Citation313,Citation314 as discussed in the section on ‘Sealing and glass stability during reflow’.
Figure 5. Effect of R2O on min. B2O3 in R2O–Bi2O3–B2O3 (R = Li, Na, K) systemsCitation247
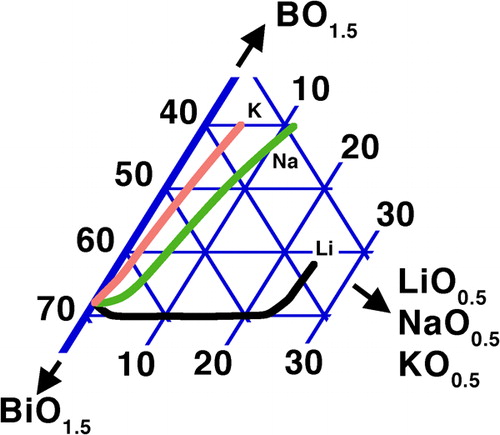
While small amounts of rare earth additions are common for luminescence studies, the effect of larger quantities on vitrification Bi2O3–B2O3 have been less studied; especially, experiments where Bi2O3 is systematically replaced by Ln2O3 (Ln = rare earth and Y, except Ce) are lacking. Experiments by Pascuta et al.,Citation276–Citation278,Citation357 while extensive, were carried out on relatively unstable glasses with a low amount of B2O3 (20–33%BO1·5), requiring fast quenching; they could replace ∼25 to 35% of the whole glass, on a cation basis, by GdO1·5 or EuO1·5 (they achieving similar results with GeO2 as a network formerCitation374). Stabilisation of the glass was also observed with 5%Fe2O3.Citation255 Compositions reported by this group for this and other systemsCitation255,Citation274,Citation276–Citation278,Citation357,Citation374,Citation384,Citation393,Citation394 must be taken with caution, however, as they used very high melting temperatures (1100°C and above) in Al2O3 crucibles and their reported glass forming ranges, >90% BiO1·5 for moderate quenching, seem too high (see ); their compositions are therefore likely to be shifted by reaction or volatilisation.
For more stable glasses (Bi2O3.2B2O3), vitrification is easy and therefore not affected by small Ln2O3 additions; however, strong suppression of crystallisation upon reheating was observed for 5%La2O3 and 3%Er2O3.Citation275 In the patent literature (complex glasses based on ZnO–Bi2O3–B2O3), maximal rare earth content is reported to be at ∼4 to 8%, lower melting glasses being more sensitive.Citation314
For the less studied silicates and germanates, a wide maximum in synergistic vitrification range is achieved when mixing Bi2O3 with PbOCitation129,Citation367,Citation369 or PbO and some BaOCitation107 and, while systematic studies are lacking, successful incorporation of large amounts of alkaline earth/transition metal oxides with low network former content is reported;Citation246,Citation250,Citation251 for instance Janakirama–Rao reports facile vitrification of 31RO+62BiO1·5+7SiO2, where R = Sr, Ba, Pb or Zn, i.e with a much lower amount of SiO2 than needed for vitrification in the binary systems, and similar results when adding two oxides (CdO and WO3, PbO and MnOy, PbO and CuOy) to the Bi2O3–SiO2 binary.Citation246 In comparison, the PbO–SiO2 system is also quite tolerant for substitution of PbO by NiO, ZnO, MnOy and FeOy,Citation395 though a decrease of required SiO2 is not observed for small substitutions, in contrast to the Bi2O3–B2O3 system.
Data on alkali additions is not as complete as with borates. At <10 cation-%Si, R2O–Bi2O3–SiO2 is reported not to vitrify for R = Li, Na or K (as with borates), but to vitrify easily with R = Rb or Cs, even with a very large (>50%) degree of substitution of Bi by R.Citation246
Extensions of the glass forming range by other oxides also occur with vanadates (Fe2O3Citation234), molybdates (PbOCitation115, Fe2O3Citation234) and even gallates (PbO and CdOCitation36). In the case of phosphates, vitrification with ZnO occurs over a wider range than with Bi2O3, but a synergistic effect is achieved nonetheless, albeit in this case with less Bi2O3 than ZnO.Citation262
Glasses without network formers
Besides providing new insights in glass formation, glasses without traditional network formers are of interest for optical applications (the section on ‘Optics’), provided other light element oxides with strong oxygen bonding (especially Al2O3) are absent.
Although early attempts to make glasses of Bi2O3 without at least a very small amount of true network formers were unsuccessful,Citation244,Citation246 several such binary systems were later successfully vitrified under twin roller quenching (), and melts with Li2O and Ga2O3 were observed to actually vitrify under relatively moderate quenching.
Adding more components facilitates glass formation, of which several examples are given in . Extension of the Li2O compositions to systems such as Li2O–BaO/PbO–Bi2O3 significantly facilitates vitrification.Citation381,Citation382 KhalilovCitation107 systematically modified glasses based on Bi2O3–SiO2 with binary or more complex combinations of PbO, BaO, CdO, ZnO and MgO, under moderate quenching (cast in metallic moulds and covered with plates), and SiO2 free Bi2O3–PbO–BaO–CdO–ZnO (+optional MgO) glasses were obtained; as the mixtures were melted in Pt crucibles, contamination by SiO2, Al2O3 or B2O3 can safely be excluded. Other similar systems are SrO–PbO–Bi2O3Citation117 and CaO–SrO–PbO–Bi2O3–CuOyCitation99 (useful for processing superconductors via the glass–ceramic route [see the section on ‘Bi2O3 in (glass-)ceramics and crystals’)], and PbO–CdO–Bi2O3–Fe2O3.Citation36 Ga2O3 was found to be particularly useful to promote glass formation, with the relatively simple PbO–Bi2O3–Ga2O3 system exhibiting easy vitrification over a wide composition range and even allowing casting of large objects.Citation36,Citation106,Citation386
Table 13. Glasses without standard network formers: complex systems
Conclusions
The following remarks may be derived from the data on glass formation:
1. | Bi3+, akin to Pb2+, is a large, polarisable ion; both, while not vitrifying alone, behave as conditional network formers, requiring ‘abnormally’ low amounts of true network formers for successful vitrification at moderate cooling rates. | ||||
2. | The Bi2O3–B2O3 system appears to be the most promising basis for the fabrication of stable lead free Bi2O3 glasses with low processing temperatures, but does not allow by itself processing temperatures as low as those of the corresponding PbO–B2O3 system. | ||||
3. | In the Bi2O3–B2O3 system, adding low amounts of SiO2 and other oxides such as ZnO, Al2O3 and Fe2O3 also stabilises the glass without largely increasing processing temperatures. Further studies, however, are needed to better define glass stability as a function of composition, especially upon reheating, which is critical for TF processes (see the section on ‘Sealing and glass stability during reflow’ for some information in this regard). | ||||
4. | Mixing several oxides of large, polarisable cations, such as Bi2O3, PbO and BaO tends to stabilise the glasses and/or lower the required amount of true network formers; adding limited amounts of other transition metal or rare earth oxides furthers this trend. | ||||
5. | Using rapid cooling, glasses containing Bi2O3 and PbO may be formed in the complete absence of true network formers. The PbO–Bi2O3–Ga2O3 system provides an especially favourable base for such HMO glasses. |
Glass structure
General considerations
Since the ‘anomalous’ ease of vitrification of Bi2O3 with standard network formers, similar to that of PbO, was noticed in early work,Citation244–Citation246 numerous studies have been devoted to elucidating the structure of Bi2O3 based glasses, using methods such as X-ray diffraction (XRD), Fourier transform infrared, Raman, electron paramagnetic/spin resonance, Mössbauer, X-ray absorption and magic angle spinning nuclear magnetic resonance (MAS-NMR) spectroscopy. A good knowledge of structural features is required to efficiently correlate glass properties with chemical data;Citation327,Citation329,Citation399–Citation401 this is especially important for borate glasses, given the different possible forms and structures assumed by borate anions.Citation402
Bonding in crystalline compounds
To provide additional insight into the structural features of Bi2O3 based glasses, a survey of a range of relevant and related crystalline oxides, where atomic positions may be precisely determined, was carried out (the section on ‘Coordination of bismuth in crystalline oxides’ in Supplementary Material 1 http://dx.doi.org/10.1179/1743280412Y.0000000010.S1). Even in crystalline oxides, Bi3+ adopts a wide variety of asymmetric, disordered and often ill defined coordination polyhedra, presumably due to its high polarisabilityCitation400 and stereochemically active ‘lone pair’ electrons, and bonding with oxygen is fairly covalent. An overview of idealised typical oxygen coordination shells observed around Bi3+ cations, in the crystalline oxides examined in the supplement, is given in , with the corresponding descriptions in . The lone pair may strongly deform the oxygen coordination shell (6-Oct33), and often replaces an oxygen anion to ‘fill’ the corresponding vacancy (3-PyM, 4-BPy/4-PyM, 5-Py14). Recently, the lone pair concept has been revisited in the light of diffraction data and spectroscopic studies of band structure, coupled with detailed computational modellingCitation403 (see other references in the section on ‘Coordination of bismuth in crystalline oxides’ in Supplementary Material 1 http://dx.doi.org/10.1179/1743280412Y.0000000010.S1); the lone pair is found to stem from interaction of both metal valence s and p orbitals, mediated by oxygen 2p ones.
Figure 6. Some oxygen coordination shells around Bi observed in crystalline oxides (see section on ‘Coordination of bismuth in crystalline oxides’ in Supplementary Material 1 http://dx.doi.org/10.1179/1743280412Y.0000000010.S1 and ): E = Bi3+ lone pair electrons
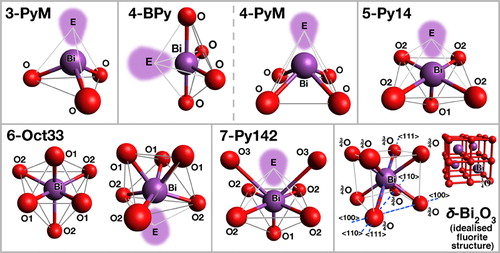
Table 14. Description of oxygen coordination shells around Bi observed in crystalline oxides (see section on ‘Coordination of bismuth in crystalline oxides’ in Supplementary Material 1 http://dx.doi.org/10.1179/1743280412Y.0000000010.S1 and ) (E stands for Bi3+ lone pair electrons)
In compounds, Bi3+ tends to have coordination number (CN) values of typically 5–7, but with very varying bond lengths and presumably strengths, with only a slight tendency to reduction to typically 5 at high Bi3+ concentrations. This reduction has little effect in practice as it only eliminates very long, weak bonds. CN = 3 is found only exceptionally, such as for a minority of Bi3+ cations in the defective sillenite γ-Bi2O3.
Pb2+ and Sn2+ are fundamentally similar to Bi3+, also being lone pair cations. However, Sn2+ has relatively well defined coordination shells and tends to low CN values, ∼3. Pb2+, being larger and more polarisable, behaves in a more similar way to Bi3+ in compounds; CN is similar to that of Bi3+ at low concentrations, but drops to 3 or 4 in Pb rich compounds. Bi3+ has more asymmetric bonding, having fairly high strongest bond valences in the range 0·8–1·3, compared to 0·6–0·7 for Pb2+ (Table S13 in Supplementary Material 1 http://dx.doi.org/10.1179/1743280412Y.0000000010.S1).
Borate glasses
The binary xBi2O3+(1−x)B2O3 system has been studied most extensively; there is general agreement on several features.Citation248,Citation317,Citation318,Citation350,Citation355,Citation394,Citation402
With addition of Bi2O3 in B2O3, the original B2O3 network, constituted of [B3O6] boroxol rings and [BO3] triangles (written BΔ, i.e. CN = 3), is initially strengthened, as in other borate glasses, by conversion of part of the BΔ groups to tetrahedral [BO4] ones (BT, CN = 4), as shown in . Boroxol rings persist only in compositions with very low Bi2O3 content, and disappear for x>25%. This initial increase in the degree of bonding results, as in other borate glasses , in an increase of Tg (, ) and network compacityCitation248,Citation317 up to x≈20–25%.
Figure 7. [BO4] tetrahedra fraction N4 in boron coordination polyhedra ([BO3] and [BO4]), for Bi2O3–B2O3 glass (○: Ref. 318; +: Ref. 247; □: Ref. 327) and crystallised glass (•: Ref. 318), (ZnO.Bi2O3)–B2O3 glass (Δ: Ref. 355) and PbO–B2O3 glass (×: Ref. 397)
![Figure 7. [BO4] tetrahedra fraction N4 in boron coordination polyhedra ([BO3] and [BO4]), for Bi2O3–B2O3 glass (○: Ref. 318; +: Ref. 247; □: Ref. 327) and crystallised glass (•: Ref. 318), (ZnO.Bi2O3)–B2O3 glass (Δ: Ref. 355) and PbO–B2O3 glass (×: Ref. 397)](/cms/asset/a4acd27c-48fc-4807-aaa2-2d3c4de5e495/yimr_a_11743737_f0007_b.jpg)
Table 15. Tg and N4 peaks in binary x(BiO1·5,SbO1·5,PbO,SnO)–(1−x)BO1·5 glasses
At low x, an ‘ideal’ modifier cation Mn+ with valence n, incorporated as its oxide MOn/2, is fully incorporated by creation of tetrahedral unitswhere Ø is the usual convenient symbolCitation350,Citation352,Citation404 for an oxygen ion making a bridging link between two network former cations (and therefore corresponding to half an oxygen atom in formulae, sometimes written ‘O½’Citation398). Ideally, the fraction N4 of [BØ4]− tetrahedra is nx/(1−x), a relation obeyed at nx smaller than ∼25% by alkaliCitation405 and large alkaline earth (Sr, Ba)Citation404 oxide additives. In contrast, N4 is much lower than expected for Bi2O3, hinting at very different behaviour from that of an ideal modifier, presumably stemming from stronger preference for retaining direct covalent Bi–O bonds over more ‘diffuse’ ones with [BØ4]− tetrahedra. This behaviour also matches that of SnO, and of other intermediate oxides such as CdO and ZnO.Citation398 In contrast, with PbO (), N4 behaves closer to ideal at low x.
For x greater than ∼25%, N4 further rises, but borate units are progressively depolymerised, as B–O–B linkages are replaced by weaker B–O–Bi ones, resulting in a drop of Tg. This behaviour is very different from simple alkali and alkaline earth borate glasses,Citation404,Citation405 where the peaks of Tg and N4 roughly coincide. PbO and SnO borate glasses also exhibit the same anomalies (), but they are much less pronounced; the exceptional structural feature in binary Bi2O3–B2O3 glasses is a very broad peak of N4≈45%, which essentially extends throughout the ‘useful’ composition range. It is therefore assumed that Bi3+ at least partly enters the network already at low x values, and the borate network progressively adapts with increasing x.Citation402 The same is also true, but to a lesser extent, for Pb2+ and Sn2+, given the lower discrepancy between the Tg and N4 peaks.
It is interesting to compare the maximum single bond valence range for Bi3+ (0·8–1·3) and Pb2+ (0·6–0·7) in crystalline compounds with the oxygen bonding deficit for different types of bonding and different boron coordinations, accounting for the variability of about ±0·05 seen for a given B–O bond in crystalline compounds (see and the section on ‘Coordination of bismuth in crystalline oxides’ in Supplementary Material 1 http://dx.doi.org/10.1179/1743280412Y.0000000010.S1, Table S13). Allowing for total variability of two B–O bonds, i.e. ±0·1 for a given Ø, some of the strongest Pb–O bonds (0·6–0·7) may still be taken up by the bonding deficit of bridging oxygen anions bound to two borate tetrahedra (about 0·5+0·1). This is not enough for the strongest Bi–O bonds, which will therefore favour direct linkage to the ‘terminal’ oxygen anions of higher nominal valence, 1·00 or 1·25 (), the bridging oxygen ions in BΔ–Ø–BT and BT–Ø–BT links being able to take up the weaker bonds for both cations. This speculative interpretation, which still requires confirmation, agrees well with observed behaviour at low x: Bi3+ has much higher deviations from ‘ideal modifier’ behaviour of N4 than Pb2+, and Tg is similar for both cations at same x, in spite of the higher valence of Bi3+. Also, the preference of Bi3+ for higher CNs and bond disorder agrees with conservation of high N4 values (i.e. mixed BΔ+BT) in a wide x range, compared to the more ordered crystalline phases and to PbO, which has lower valence and favours lower CNs ().
Table 16. Nominal bonding deficit of oxygen anions in borate glasses versus structure
Anomalies in propertiesCitation317,Citation318,Citation350 such as density and Tg yield other hints on the structure; they are often correlated with compositions close to that of crystalline phases in the corresponding oxide systems. Based on this observation, a tendency to form local groupings in the glass similar to those that exist in the crystals was also postulated for the BaO–Bi2O3–B2O3 system,Citation350 as typically found in borate glasses.Citation402 Comparing data on glassy and crystallised Bi2O3–B2O3 samples on , however, one can see that this structural similitude progressively breaks down at high x values, where Bi2O3 becomes the dominant species and obviously assumes the function of network former, with significant amounts of O2− anions not bound to boron (i.e. only to Bi3+) identified at about x⩾65%.Citation255,Citation394
In these Bi2O3 rich compositions, in spite of extensive characterisation work with well controlled samples, there are significant discrepancies in the reported N4 values, as illustrated in ; the work of Terashima et al. used by DimitrovCitation327 seems somewhat at odds with that of Bajaj et al.Citation318 (and previous work cited by the latterCitation247), although the same method (MAS-NMR) was used in both cases and sample fabrication appeared to be well controlled (moderate melting temperatures and noble metal crucibles). Residual impuritiesCitation318 could possibly account for some of the discrepancies, as well as thermal history (quenching rate and subsequent annealing), which significantly influences glass propertiesCitation376 and even structure (see discussion on ‘polyamorphism’Citation280,Citation318); interestingly, Terashima's data lies roughly halfway between Bajaj’s for glassy and crystallised samples.
Given the differences in N4 between crystalline and glassy samples, shifts of the CN of Bi3+ in glass vs. in crystals can also be expected, but Bi3+ is less sensitive in this respect than Pb2+, as seen in the section on ‘Coordination of bismuth in crystalline oxides’ in Supplementary Material 1 http://dx.doi.org/10.1179/1743280412Y.0000000010.S1. In fact, most structural studiesCitation132,Citation255,Citation275,Citation276,Citation326,Citation352,Citation359,Citation360 in the binary or almost binary Bi2O3–B2O3 system favour retention of ‘distorted [BiO6] octahedra’ throughout the composition range, and low CN groups are not seen in Bi2O3–B2O3 glasses at least up to x≈70%. Above this level, presence of a minority of [BiO3] groups has been reportedCitation357,Citation394 (threshold uncertain due to likely significant contamination from the Al2O3 crucible), in line with the structure of high Bi2O3 crystalline boron sillenite Bi12(Bi0·25B0·50)O19·125 (see the section on ‘Coordination of bismuth in crystalline oxides’ in Supplementary Material 1 http://dx.doi.org/10.1179/1743280412Y.0000000010.S1). This agrees well with [BiO3] groups appearing together with sillenite crystallisation for about x ⩾65% in heat treated glasses.Citation276,Citation394 The reported presence of [BiO3] groups at moderate Bi contents in borate, borosilicateCitation362 and aluminoborateCitation277,Citation278 glasses is doubtful, and most likely results from IR peak misassignment, expected [BiO3] peaks lying at ∼480 and 840 cm−1.Citation276,Citation357,Citation374,Citation394
Substituting some of the Bi2O3 with compounds such as ZnO, PbO and BaOCitation350,Citation352,Citation355 yields results very similar to that of Bajaj and Bishay,Citation247,Citation318 as shown for Zn in , with only slight offsets due to the elemental substitution. Ba2+ enters the glass as a modifier,Citation266 while Zn2+ may do the same at low concentrations,Citation266 but forms [ZnO4] tetrahedra at high ones.Citation122,Citation406
Concerning more complex systems, presence of [BiO3] groups is reported in (Li2O)–ZnO–Bi2O3–B2O3Citation264,Citation337,Citation406 and Li2O–Bi2O3–(B2O3)Citation334,Citation335 glasses only at high Bi2O3 and low B2O3 contents, Bi3+ being otherwise present as [BiO6] only.
Compared to Bi3+, the coordination of Pb2+ is somewhat more ordered and much more dependent on x in binary borate glasses, according to XRD and MAS-NMR studies:Citation397 Coordination number is 6 up to about x = 25%, then decreases continuously, reaching 3 for x equal or greater than ∼55%, which is matched by a corresponding decrease of average bond length from ∼300 down to 233 pm. Pb2+ is therefore roughly present as [PbO6] octahedra and behaves somewhat as a classical modifier at low x, and progressively switches at higher x, well within the vitrification range, to [PbO3] network forming trigonal pyramids (3-PyM), with fewer, stronger Pb-O bonds. However, while the average bond length found for [PbO3] agrees well with bonding in Pb rich crystalline compounds (the section on ‘Coordination of bismuth in crystalline oxides’ in Supplementary Material 1 http://dx.doi.org/10.1179/1743280412Y.0000000010.S1), the corresponding length for [PbO6] is clearly too long, which suggests bonding is also somewhat inhomogeneous at low x (existence of shorter bonds). This, together with N4 being slightly less than ideal and Tg starting to drop at lower x values than the CN, hints at some departure of Pb2+ from pure modifier behaviour, even at low x, albeit to a much lesser extent than Bi3+. At high x, there is also some ambiguity in the 3-PyM configuration, as there are additional, weaker bonds, as seen in the crystals;Citation407 the configuration can be viewed as 4-PyM (more 3+1, with one longer bond), with additional, much longer ones above the pyramid apex.
Silicate and germanate glasses
The case of silicateCitation408 and germanateCitation231,Citation374,Citation393,Citation409 glasses is much simpler, as the [SiO4] and [GeO4] tetrahedra are conserved when Bi2O3 is added, with no reported formation of [GeO6] octahedra as found in the alkaline germanate glasses. The only change is gradual weakening of the original network, as attested by the continuous drop of Tg with increasing x in the xBiO1·5+(1−x)(Ge,Si)O2 system, as shown in for silicates. Absence of [GeO6] octahedra was also reported in complex germanate glasses, with V2O5,Citation231 Ga2O3Citation359,Citation360 and PbO–Ga2O3Citation370 additions.
Bi3+ is generally reported as being present in the form of [BiO6] groups, but also as [BiO5], from X-ray absorption spectra and molecular dynamics calculations.Citation408 Given the very high disorder around Bi3+, this difference in reported structure is probably not very significant. As for borates, no [BiO3] groups were found to high x values (80%) in Bi2O3–GeO2(–Eu2O3) glasses. On the other hand, after heat treatment and crystallisation, [BiO3], [BiO6], [GeO4] and [GeO6] groups appeared, with Bi2GeO5, Bi4Ge3O12 and unidentified peaks seen in the XRD spectra.Citation374 Neither [BiO3] 3-PyM pyramids nor [GeO6] octahedra exist in the identified crystalline structures (the section on ‘Coordination of bismuth in crystalline oxides’ in Supplementary Material 1 http://dx.doi.org/10.1179/1743280412Y.0000000010.S1), but this apparent conflict may be resolved for [BiO3], assuming some of the unidentified crystalline phase is the [BiO3] containing Ge sillenite Bi12GeO20, whose formation would be expected at x = 80%, as seen in borates.Citation276,Citation394 The presence of [GeO6] is more doubtful, as it is also absent in sillenite and Bi2Ge3O9 as well, but could be possible in the residual glass due to the thermal history or in an unidentified metastable phase.
The existence of [GeO6] groups in PbO–GeO2 glasses has been reported, albeit to a much lower extent than in the alkali germanates,Citation12,Citation410 but more recent workCitation409 concludes to all Ge4+ being in [GeO4] tetrahedra. There is basic consensus that Pb2+ forms [PbO3/4] (3/4-PyM) pyramids in Pb rich PbO–SiO2Citation407,Citation411,Citation412 and PbO–GeO2Citation413 glasses. On the other hand, Pb2+ coordination at lower lead contents has been questioned recently. CN = 6 was found at up to 40%PbO in PbO–GeO2,Citation410 and progressive switch from network modifier to former behaviour (presumably [PbO6]→[PbO3/4]) up to 40% PbO in PbO–SiO2.Citation414 Somewhat at odds with these results, Pb2+ was found to form [PbO3/4] pyramids down to 30%PbO in PbO–SiO2,Citation412 a behaviour similar to that found in SnO–SiO2 glasses, where Sn2+ essentially appears in 3-PyM coordination, with CN only slightly increasing at low SnO contents.Citation415
Phosphate glasses
The binary Bi2O3–P2O5 system has received only scant attention due to its limited vitrification range, which probably stems from easy crystallisation of high melting BiPO4;Citation377 Bi2O3 is therefore mostly found as an additive (intended or as wasteCitation96,Citation98) in multicomponent glasses.
Replacing part of Fe3+ in a 40Fe2O3–60P2O5 glass with isovalent Bi3+ is found to effect only limited changes to the structure;Citation228 expectedly, the phosphate groups are mostly present as Q1 pyrophosphate units,Citation209,Citation210 and both Fe3+ and Bi3+ are present as hexacoordinated octahedral units. Similar incorporation as [BiO6] was determined for ZnO–Bi2O3–P2O5Citation262,Citation265,Citation267 and Li2O–Bi2O3–P2O5Citation378 glasses. As for the other systems, Bi2O3 was concluded to behave partly as a network former. In comparison, SnO in 3-PyM coordination can also enter the glass network, being able to vitrify with fully depolymerised phosphate groups.Citation218
Gallate glasses
Ga3+ is found to form [GaO4] tetrahedral groups in HMO glasses throughout the ternary PbO–Bi2O3–Ga2O3Citation109,Citation111,Citation385,Citation386 and Bi2O3–Ga2O3–B2O3 CitationCitation359,360 systems, as well as more complex PbO–(PbF2)–Bi2O3–Ga2O3–GeO2Citation370,Citation371 compositions, with good agreement of Ga–O bond lengths with those found in crystals.Citation109,Citation111 As in other HMO rich systems, Bi3+ and Pb2+ are reported to form ‘[BiO6]’ groups and [PbO3/4] (3/4-PyM) pyramids respectively, with a higher degree of disorder around Bi3+.
In these glasses, coordination around Bi3+ and Pb2+ has been examined more extensively. Assignment of Raman bands for Bi–O bondsCitation360 agrees with the Bi3+ bonding in crystals (the section on ‘Coordination of bismuth in crystalline oxides’ in Supplementary Material 1 http://dx.doi.org/10.1179/1743280412Y.0000000010.S1, deformed 5-Py14 pyramids), with a short apical bond, and two groups of unequal bonds on either side of the pyramid base. The last, much weaker bond assigned in glasses to complete a ‘[BiO6] octahedron’ could actually correspond to a pair, as found in the 7-Py142 configuration often reported in crystals. These results also agree very well with detailed neutron and XRD studies of a binary 80BiO1·5+20GaO1·5 glass,Citation109 which yield CN≈5 for Bi3+, with roughly 1, 2 and 2 oxygen anions at 213, 224 and 252 pm respectively. Obviously, CN of Bi3+ stays high even for a very Bi rich glass, influencing the CN of Pb2+ in the ternary PbO–Bi2O3–Ga2O3 system, which was found to decrease from 3·5 for Bi free glasses to 3·0 for Bi rich ones.Citation111 This is logical, as the higher oxygen CN of Bi3+ creates a severe oxygen shortage, which is partly compensated by decrease of Pb2+ CN. Nevertheless, in all these HMO rich glasses, CN > 2 for oxygen, with CN = 3·5 for the binary 80BiO1·5+20GaO1·5 glass.
Other glasses
VanadateCitation231,Citation232 and molybdateCitation232,Citation234 glasses exhibit a change of network former coordination polyhedra when adding Bi2O3:[VO5] trigonal bipyramids and [MoO6] octahedra are converted to [VO4] and [MoO4] tetrahedra respectively. Tellurates are even more complex, and tend to integrate only little Bi2O3, together with a third oxide; the original irregular [TeO4] trigonal bipyramid (4-BPy, Te4+ also being a lone pair ion) of TeO2 is partly converted, depending on Bi2O3 and other oxides, to [TeO3] (3-PyM) pyramids and [TeO3+1] polyhedra.Citation120,Citation126,Citation237,Citation239,Citation240 Bi3+ is reported to form ‘[BiO6]’ groups, as with standard network formers.
Glasses without network formers
Structural studies on systems where mainly Bi2O3 forms the network are relatively scarce. In Li2O–Bi2O3 glasses, a disordered local structure, analogous to crystalline Bi2O4, was assumed; it was rationalised that the nominal additional oxygen was provided by Li2O, and even very atypical partial oxidation to Bi5+ (see the section on ‘Oxidation state of Bi species in glasses’ in Supplementary Material 1 http://dx.doi.org/10.1179/1743280412Y.0000000010.S1), the rest being compensated by defects.
Structural analysis of glasses based on a nominal 89BiO1·5+11PbO formulation, probably contaminated with Al2O3 from the crucible and optionally doped with MnOy, expectedly yields coordination of Pb2+ as [PbO3/4] (3/4-PyM) groups. Bi3+ was found in the Mn free glass mainly as [BiO6] groups, with a minority of [BiO3]. However, the reported exclusive formation of [BiO3] pyramids in Mn doped glass must be taken with caution, as this does not correspond to any relevant Bi based compound.
Conclusions
Concerning the coordination of Bi3+ in glass, most IR and Raman spectroscopic studies on conclude that Bi3+ essentially forms distorted [BiO6] (CN≈6) octahedral-like configuration, with little variation of CN over a wide concentration range; a minority of [BiO3] pyramids is found only in very Bi rich compositions, in agreement with their presence in the sillenite crystalline structure (see the section on ‘Coordination of bismuth in crystalline oxides’ in Supplementary Material 1 http://dx.doi.org/10.1179/1743280412Y.0000000010.S1). The term ‘[BiO6] octahedron’ must be taken with proper caution, as coordination around Bi3+ tends to be ill defined; more dedicated studiesCitation109,Citation408 yield CN≈5 with nonuniform bond lengths, corresponding to a deformed octahedron with an oxygen vertex replaced by the Bi3+ lone pair E and thus yielding a [BiO5E] unit (, 5-Py14 configuration). This agrees well with assignment of Raman bands;Citation360 the additional Raman band attributed to a further, weaker bond could in fact correspond to two such bonds, matching the 7-Py142 configuration often found in crystalline compounds.
The high CN maintained by Bi3+ to high concentrations necessitates a CN of O2− greater than 2, apparently violating the classical rules for glass formation.Citation416 This is also true for Pb2+ and Sn2+, which have lower CN but lower valence as well. However, in contrast to Bi3+ and Sn2+, coordination around Pb2+ is seen, at least in some cases such as the PbO–Bi2O3–Ga2O3 and PbO–B2O3 systems, to be more dependent on the local environment and available oxygen to bond to.
A comparison of the analogous divalent and trivalent lone pair cations () sheds new light into their structural features and trends. In line with original predictions,Citation416 the rigid 3-PyM oxygen coordination shell of the lightest trivalent cation, As3+, makes it a classical network former, as is (almost) the case for Sb3+, whose coordination is somewhat less rigid.Citation417,Citation418 Sn2+ is relatively rigid and favours a similar coordination, but intrinsically cannot form a glass on its own due to its valence being lower than its CN. Note that As3+, Sb3+ and Sn2+ are not stable in air, and Sn2+ tends to disproportionate into Sn0 and Sn4+.
Table 17. Oxygen coordination trends of ‘lone pair’ cations versus concentration x
The larger polarisable cations, Pb2+ and Bi3+ have much more variable and disorderly coordination shells, with CN behaving somewhat the same way as in crystals, i.e. being somewhat concentration dependent for Pb2+, and less so for Bi3+. Nevertheless, a tendency remains to form a limited number (3–4) of comparatively stronger bonds. One can speculatively view this inhomogeneous metal oxygen bonding as a reflection of the ambiguous behaviour of these cations, as both network formers (through the stronger metal oxygen bonds) and network modifiers (through the weak ones); considering the strong bonds only reduces the CN of oxygen, making it more comfpatible with classical rules of glass formation. More studies are clearly needed to arrive at a better definition of these complex and disordered coordination environments, possibly assisted by molecular dynamics simulationsCitation408 coupled with cation–oxygen interactions based on recent revisonsCitation403 (see also the section on ‘Coordifnation of bismuth in crystalline oxides’ in Supplementary Material 1 http://dx.doi.org/10.1179/1743280412Y.0000000010.S1) of lone pair bonding in crystals.
Oxidoreduction issues
Possible reduction of Bi3+ to metal during glass preparation,Citation118 and later during processing, for instance through transient reducing conditions brought about by binder burnout, is even more pronounced than for Pb2+, as Bi2O3 is even less stable towards reduction than PbO.Citation59,Citation419–Citation422 This can be a problem for processing, especially of low melting glasses due to difficulty in burning out the organics. One must, however, mention that precious metal oxides used in TFRs, such as RuO2, are even much less stable towards reduction (see PDC-5015) than Bi2O3, so Bi2O3 reduction is not the limiting problem overall for standard air firing TF electronics.Citation423 Finally, a moderate sensitivity to reduction actually can be beneficial in some respects, especially solderability of conductors (see the section on ‘Metallisations and TF conductors’).
Unfortunately, information about the thermodynamics of Bi2O3 (and other oxides) in glasses is rather limited: polarimetric studies were carried outCitation424 on a borosilicate glass with very low (0·25% mol) Bi2O3 additions, but the results are not directly applicable to glasses where Bi2O3 is one of the main components, as those concerned in the present work. Nevertheless, recent reduction experimentsCitation366,Citation408,Citation425–Citation427 and results of high temperature firingCitation118,Citation375 do confirm easy reduction and formation of Bi0 nanoparticles, or, for glasses doped with low amounts Bi, presumably reduced species, whose nature is still subject to debate.Citation428–Citation430
Control of reduction, as in more common industrial glasses, may be achieved by ‘fining agents’, i.e. oxidoreduction buffers that inhibit reduction to Bi0 under practical firing conditions; this has been shown to be successful with low Sb, As, Ce or Cu additions,Citation112,Citation114,Citation273,Citation361,Citation431 with CeO2 often found in the patent literature.Citation311,Citation314,Citation432 Alternatively, using a fugitive oxidant such as KClO4 and KNO3 allows controlled reduction and precipitation of Bi0 nanoparticles to create a well defined surface plasmon resonance band.Citation427 Additionally, the other main glass constituents, by affecting the overall basicity of the glass, will also somewhat influence the tendency of Bi3+ towards reduction.Citation399,Citation430
Finally, further oxidoreduction issues involving interaction with adjacent layers, such as adhesion on metal (the section on ‘Dielectrics on metal substrates’), staining of glasses by in-diffusion from Ag conductors (the section on ‘Overglazing/enamelling’) and contacts to PV cells (the section on ‘Metallisations and TF conductors’), as well as optical properties, are discussed in the corresponding sections. Also, a strong point is made in the section on ‘Oxidation state of Bi species in glasses’ in Supplementary Material 1 http://dx.doi.org/10.1179/1743280412Y.0000000010.S1 against, except in unusual circumstances, the occasionally reported presence of significant amounts of Bi5+ in glasses.
Applications in layer form
This section discusses in more detail the application of Bi2O3 based glasses, using TF or similar technology, to electronics, automotive and architectural glass, display panels and PVs. Uses in bulk form are discussed in the section on ‘Other applications’. The present discussion will mostly concentrate on materials covering the low processing temperature range, the main application of the Bi2O3 based glasses and the PbO based ones they should replace.
The following four sections, from ‘Sealing and glass stability during reflow’ to ‘Dielectrics on metal substrates’ discuss applications of insulating glass based layers in the four main configurations illustrated in , each corresponding to a specific role for the glass based layer and determining the required behaviour during firing and the insulating characteristics: sealing, overglazing/enamelling, multilayer dielectrics and dielectrics for insulating metal substrates. The section on ‘Sealing and glass stability during reflow’ also discusses glass stability upon refiring, as low temperature sealing is the most demanding application in this respect. Finally, the sections on ‘Metallisations and TF conductors’ and on ‘Glasses for TFRs’ discuss conductors/metallisations, and TFRs respectively.
Sealing and glass stability during reflow
Sealing stands apart from the other applications in that the sealing material must ideally be able to flow extensively during processing, in contrast to the other applications, where densification only is to be achieved. In the classical leaded sealing glasses, some of the PbO is often replaced by Bi2O3 to improve flowability,Citation150,Citation151,Citation242 stability against devitrification and compatibility with temperature lowering fluoride additions,Citation149 or even strength,Citation152 However, as discussed in the ‘Introduction’ part of the section on ‘Bismuth glasses’, replacing most or all of the PbO by Bi2O3 results in increased viscosity,Citation149 which may in most cases be mitigated by alkaline or fluoride additions to the extent durability and stability is not excessively degraded. As possible conductors going through the seal are usually only in a side-by-side configuration (), insulating properties should not be critical in most applications, except in PDPs,CitationCitation181,308 where conductors are on both sides.
Sealing glass may be formulated to be either stable or devitrifying.Citation15,Citation18,Citation74 Ideally, devitrifying seals yield the best properties, but they also tend to have tight processing requirements, limited flowability and are usually not applicable for low temperature sealing, where ‘composite’ glasses are used, i.e. stable glasses with low expansion fillers to adjust the CTE.Citation18 In any case, extensive flow must be insured without or before crystallisation, which means sealing is arguably the most demanding application in terms of glass stability. In terms of glass formulation, this requirement tends to be in contradiction with the need for low softening temperature, as discussed hereafter.
Glass stability and devitrification in the Bi2O3–B2O3 binary system, which is the basis for most of the commercial formulations, has been the object of several studies.Citation110,Citation317,Citation318,Citation326 Except the oldest study,Citation110 they agree on a practical stability optimum near 45% BiO1·5, in agreement with the break in the liquidus temperature (), versus falling Tg (); this somewhat lower optimum than would suggest lies in crystallisation of the metastable phase BiBO3.Citation317
As discussed in the section on ‘Glass formation’, small additions of other network formers and modifiers, as well as oxides such as Fe2O3 and lanthanides, hinder crystallisation; ZnO–Bi2O3–B2O3–SiO2 compositions specified in the early Soviet patents (: B80, B82 and B89), reported there as non crystallising and containing no alkalis, provide a good starting basis for low temperature sealing glasses, and the overwhelming majority of the subsequent research and patent literature report very similar compositions.
Hasegawa et al.,Citation313,Citation314 in their patent applications, performed arguably the most extensive discussion, although very empirical and on a weight basis, of composition optimisation and stability in this system; the resulting restrictions, converted to a cation molar percentage basis, are given in and discussed hereafter.
Table 18. Composition ranges and ratios specified for sealing glass stabilityCitation314
The indicated data are according the second application,Citation314 which specifies a minimum amount of Al2O3 (recognising its beneficial effect on glass stability), and introduces a second, more restrictive composition range for low temperature FPD sealing with fillers, which apparently requires even better stability against crystallisation. This can be due to the filler possibly promoting crystallisation and the sealing temperature lying in the range where crystallisation is most apt to occur; sealing processes that require stronger capillary flow or firing of conductors/resistor frits occur at higher temperatures where crystallisation is less likely, i.e. slightly below or even above the liquidus.
Overall, the requirements stated in and corresponding compositions (, Hg) correlate fairly well with glass formation (the section on ‘Glass formation’) and properties (the ‘Introduction’ part of the section on ‘Bismuth glasses’), as well as with our own preliminary refire stability experiments, performed with glass frits printed on alumina and glass and summarised in . The main network former is B2O3, to minimise processing temperatures, with SiO2 and preferably some Al2O3 being added to improve stability.
Figure 9. Results, in cation-%, of our experiments on stability of Bi2O3 based glasses in TF firing cycles (belt oven, 45 min total time with 10 min at peak, 400–700°C)
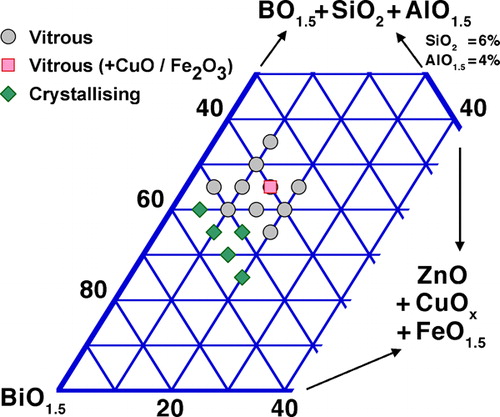
The beneficial effects of mixing B2O3, SiO2 and Al2O3 are illustrated by comparing with other work where either B2O3 or SiO2 were used alone;Citation311,Citation355,Citation433 densification is reported to be problematic, with a strong tendency to crystallisation.Citation355,Citation433 Crystallisation problems may also be inferred from a higher sealing temperature, in spite of a low filler volume fraction.Citation311 In accordance with ,Citation314 using a very low amount of SiO2 in SrO–ZnO–Bi2O3–B2O3 (Nch-01), is reported to yield a devitrified seal, albeit with enough prior flow to achieve successful densification.Citation312
Stability is improved by low levels of ZnO and alkaline earth additions, and degraded by excessive amounts, in line with vitrification studies (see e.g. ) and other work.Citation263 The stated maximum amounts of alkaline earths should be taken with caution, as they were added without regard to ZnO; a more pertinent test would be to add them with corresponding reduction of ZnO, i.e. keeping Zn+alkaline earths constant. Rare earths (Ln), including cerium, are stated to improve mechanical strength, an advantage compounded by CeO2 being a useful fining agent (the section on ‘Oxidoreduction issues’), and a low level of lanthanides providing stability versus devitrification.Citation275 One not mentioned compound is Fe2O3, which is reported, together with CuOy/MnOy, to be a useful alternative to Al2O3 against devitrification in modern very low melting lead bearing sealing glasses;Citation149,Citation151,Citation242 interestingly, Fe2O3 seems to stabilise Bi2O3 based glasses as well.Citation255 These effects are expected to strongly depend on composition, i.e. formed crystalline phase(s) according to phase diagram, i.e. borates for low melting glasses. In higher melting, essentially Bi2O3–SiO2 glasses, crystallisation of Bi2SiO5 has been found to be promoted by Cr2O3, but inhibited by CuOy and MnOy.Citation301
Practical sealing examples, taken from the patent literature,Citation149,Citation242,Citation311,Citation314 given in , illustrate the potential of the new Bi based glasses (Hg-203/324/Usu-5) for low temperature sealing, well below the softening point of common float glass, and thus clearly demonstrates their industrial usefulness.
Table 19. Composite sealing glasses (compositions: see )
On the other hand, these examples also illustrate the remaining gap with the Pb bearing frits (LTS) for ultra low temperature sealing of electronic and optical devices at temperatures compatible with some engineering polymers, e.g. glass fibre coatings,Citation242 in spite of these compositions not containing any alkalis. However, further studies combining fluxing additives such as F−, TeO2, V2O5, Nb2O5 and MoO3 with stabilising agents (Ln2O3, Fe2O3, etc.), i.e. optimisation similar to that of the lead bearing frits, should yield further progress in lowering achievable sealing temperatures.
Overglazing/enamelling
Low melting glassy layers (overglazes, or enamels) find a large palette of applications in electronics,Citation291,Citation302,Citation307,Citation321 displays,Citation181 automotive/architectural/container glassCitation33,Citation47,Citation432 and cookware/dishware/chinaware,Citation47,Citation56 as protective and decorative layers. Examples and properties are given in .
Table 20. Overglaze/enamel/dielectric properties (glass compositions: see )
In TF electronics, overglazes protect resistors against humidity, and Ag rich conductors against electrochemical migration, thereby improving circuit reliability.Citation63,Citation434 Other functions useful during processing are solder masking and protection of resistors against acid electrochemical plating baths. As for sealing, conductors are absent or lie side-by-side (); excellent electrical insulation characteristics are therefore in most cases not necessary, and minor alkali additions are usually allowed.
The choice of a TF overglaze is a balance between limiting the shift in resistor characteristics and guaranteeing a sufficient level of protection. Both standard (e.g. ESL 4771P, ) and acid resistant (such as Her CL90-8325, roughly equivalent to lead bearing ESL-481) lead free overglazes are now available from most TF materials suppliers. For very low required processing temperatures, one may use the sealing glasses discussed in the previous section, adding fillers to increase strength/abrasion resistance or to match the CTE to that of the substrate, or slightly higher firing glasses.Citation291
As for the older lead bearing glasses, acid resistant types require a higher firing temperature, ca. 600°C, than standard ones. However, extensive publications on the chemistry and properties of Bi based TFR overglazes are still lacking, although some examples are available in the patent literature (, Hy-29Citation321 and Don-01/04Citation307 for the ∼600°C firing types); information may be garnered, for the low firing (⩽550°C) types, from sealing glasses.Citation289,Citation290,Citation293,Citation313Citation,314
A related application is the overglazing of displays (FPDs), where, besides providing suitable protection, the overglaze must avoid excessive degradation of the transparent conductor, usually indium tin oxide on the glass. This is achieved using low firing glasses similar to the sealing types, with a low level of In2O3, SnO2 and SbOy additives.Citation297,Citation308
Low firing TF circuits (e.g. for FPDs) mostly use pure or almost pure Ag conductors, as Pd tends to oxidise in the intermediate firing range,Citation64 which raises the issue of staining by in- diffusion of Ag+ and probable reprecipitation of Ag0. This is prevented by CuCitation308,Citation435 and CrCitation306 additions, presumably through oxidoreduction mechanisms.
Compared to consumer electronics, automotive and architectural enamels are exposed to much more severe outdoors environments, such as acid rain.Citation33,Citation47,Citation48 In a way typical of the high volume cost sensitive automotive industry, single window rim dark enamel coating fulfils multiple roles, as illustrated in : antistick agent during forming of the panel, and hiding of the adhesive and its protection against ultraviolet (UV) light. Moreover, to optimise costs, the enamel must fire and achieve antistick properties reliably during the heating step required for forming of the window pane (650–700°CCitation47,Citation436), and be sufficiently resistant to atmospheric attack to avoid requiring extra protective layers.
Figure 10. Application of enamel to automotive glass, as a antistick layer during forming and b adhesive protection layer (redrawn from SakoskeCitation47)
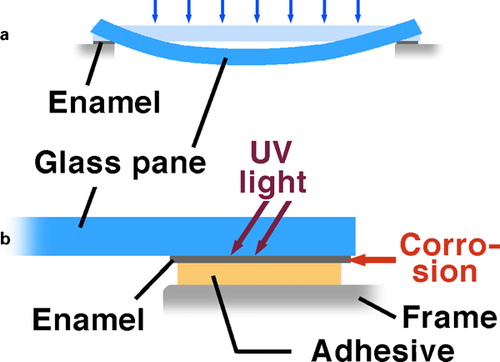
Such enamels have recently undergone extensive development, as attested in the patent literature.Citation298,Citation299,Citation301,Citation432,Citation436–Citation439 Firing temperatures are in the 600–675°C range suitable for glass processing. First compositions were mainly glassy, relying on pigments to provide antistick properties, but have been replaced by crystallising compositions optimised to achieve the best chemical durability. In this respect, the trend has gone from Zn boratesCitation437 to Zn/Bi silicatesCitation438–Citation440 and finally to mixed Bi (Bi4Ti3O12, Bi2Ti2O7, Bi2Ti3O9) and other (Zn, V–Bi) titanates,Citation302,Citation436 which are claimed to exhibit better chemical resistance to acid attack. This is generally in accordance with the investigations of Fredericci et al.,Citation48 who report increasing acid resistance going from Zn silicate to Bi silicate to Bi titanate phases, and the facile crystallisation of titanate phases.Citation48,Citation363 Other compounds with ‘acidic’ oxides such as V2O5, Nb2O5, Ta2O5, MoO3 and WO3, alone or mixed,Citation364 may probably have similar beneficial effects on acid resistance, but the composition must also be tuned in order to ensure sufficient durability of the remaining glass phase.
When coating glass or ceramics, an important issue is the degradation of the strength of the glass substrate by application of the enamel.Citation47,Citation441 This is not specific to Bi based glasses, as similar effects have also been observed by our group on ceramic TF circuits with ‘classical’ lead bearing materials.Citation442 The implications are significant, as automotive windshields play a structurally important role in cars, and TF electronics are used in high reliability, safety critical applications.
Insulating dielectrics
As reliable crystallisable lead free dielectrics are readily available for standard 850°C firing conditions,Citation163 Bi based glasses are mainly applied to low firing insulating layers for circuitry on glass (FPDs/PDPs)Citation181,Citation308,Citation431 or metal.Citation71,Citation323 Typical configurations are multilayer circuits and crossovers (), as well as the deposition of complete circuits on metal (D).
Dielectrics may be formulated essentially the same way as filled sealing glasses, with the difference that some sort of ‘stabilisation’ is desirable. Otherwise, the dielectric (e.g. L-Bi12, ) tends to reflow upon subsequent firing of conductors and resistors. Stabilisation may be achieved by crystallisation (spontaneous or filler nucleated)Citation312 and/or by chemical reaction with the filler, as successfully experimented with PbO bearing glasses.Citation161,Citation162 Bi2O3 should react similarly, with the ‘acidic’ oxides mentioned in the previous section; tuning the reactivity may be achieved by varying the glass composition, as well as the chemistry, amount and particle size of reactive filler.
Dielectrics on metal substrates
Commercial TF dielectrics/enamels for firing onto stainless steel exist (see ), but, as the porcelain enamelled steel they replace, they require high processing temperatures unsuitable for sensors or application to substrates such as titanium (limited by oxidation) or aluminium (limited by melting),Citation68,Citation71 and low firing dielectrics must be used.
However, dielectrics on metal face one important issue stemming from the reducing conditions (the section on ‘Oxidoreduction issues’) at the substrate/dielectric interface; to ensure good adhesion, the formation of soft and low melting metallic Bi and Pb should be avoided. Pre-oxidation of the metal is not always possible or useful due to reasons such as manufacturing process requirements, insufficient oxide adhesion or thickness. Moreover additional reducing conditions may be generated, on alloys forming Cr2O3 scales such as stainless steels, by chromate formation (Cr3+→6+) with glass components such BaO, PbO and Bi2O3,Citation443,Citation444 although Cr may also remain as Cr3+ in the glass.Citation373 Therefore, a generally applicable approach on metallic substrates consists in including significant amounts of transition metals that may be partially reduced in the glass (Fe3+→2+, Mn4+→3+→2+, Co3+→2+, Cu2+→1+), and that are moreover not deleterious when fully reduced to metal; Co is in fact found in dielectrics for steel substrates (). As the situation is very similar to PbO, it is surmised that our previous experiments with Pb based glasses, where Fe2O3 additions were successfully applied,Citation68,Citation71 may be extended to Bi based ones.
It must be mentioned no adhesion problems were encountered on Al alloys,Citation445 in spite of the very strong reducing power of Al; it is surmised that formation of Al2O3 rapidly results in the effective stoppage of oxygen diffusion, and hence limited interaction with the substrate, a hypothesis supported by the absence of oxidoreduction buffers in commercial enamels for Al.Citation56
The high CTE some metals, such as austenitic stainless steel (17 ppm K−1) and aluminium alloys (23) constitutes an additional issue, causing warping of thin substrates and excessive stress build-up in the dielectric. This may be corrected by suitable high expansion fillers such as quartz, cristobalite, CaF2 and AlPO4, using chemical particle coating techniques if chemical reactions must be limited. In contrast, ferritic low alloy or stainless steel (∼11 ppm K−1) and Ti alloys (8–10) are reasonably well matched with low melting Bi based frits (8–11).
As a final note, commercial lead and alkali free bilayer low firing dielectrics on aluminium substrates have recently become available,Citation446 but no detailed studies on their composition has been carried out to date.
Metallisations and TF conductors
Metallisations and conductors for electroceramic components such as resistors, capacitors voltage limiters, for solar cells, for automotive window heaters and TF conductors fundamentally share the same technology, i.e. the starting material is a finely divided conductor powder such as Ag, Ag/Pd, Au, Cu, with oxide and/or glass frit additive that promote densification and bonding to the substrate.Citation21,Citation60,Citation63,Citation64,Citation447–Citation451 While traditional lead borosilicate frits are widely used, application of Bi based frits in conductors is hardly new, with the 1st patent going back to 1945,Citation283 and such conductors being widely in use by the early 1970sCitation88,Citation449,Citation452 (see also ). Mixed PbO–Bi2O3–B2O3–SiO2 glass frits have also been used, and Bi2O3 is also added in its own right, without nominally being part of a glass frit,Citation158,Citation159,Citation288,Citation310,Citation452–Citation457 in order to impart good adhesion by reacting with the substrate and potentially also with the metals, as well as for promoting liquation in fritless conductors.Citation158,Citation457,Citation458 Its reactivity with 96%Al2O3 (+3·8 mass-%SiO2 and 0·2 mass-%CaO), 99·9%Al2O3 and BeO has been extensively studied.Citation459 Bi2O3 does reacts with Al2O3, but reaction with the SiO2 contained in the glass phase of 96%Al2O3 is dominant below 850°C. Finally, Bi2O3 (and PbO) may also be added to conductor metallisations for electroceramics that contain these elements, in order to control the chemical interactions.Citation451 The technical importance of integrating elements in the frit or adding them separately is not clear, i.e. avoidance of patent infringement may also play an important role.
The rather low affinity to oxygen of bismuth makes it easily reduced, at least partially, during firing or soldering. It is believed that partial reduction of Bi and Cu and partition between noble metal and oxides favours the formation of bridging bonds and thereby good adhesion.Citation88,Citation159,Citation251 Solderability is of course also favourably impacted, but aged adhesion of solder pads can be affected if reduction of bismuth by tin in solder proceeds in service,Citation64,Citation449 a problem that may be mitigated by incorporation of Bi2O3 into other oxides or glass frit (initially or during firing) or by adding oxides that act as barriers against this reaction.Citation160,Citation456 Bi2O3 may also lead to formation of lossy semiconducting interfacial reaction products at electrocomponent interfacesCitation310 and, in popular Ag–Pd metallisations, reinforce the problematic oxidation of Pd to PdO at intermediate temperatures by formation of Bi2PdO4, retarding Pd reduction at high temperatures.Citation64,Citation460
The above considerations are summarised in . Relatively recent workCitation159,Citation180,Citation250,Citation433,Citation455,Citation456 details both fritless and fritted (, Hw-00) Bi containing Pb free silver based conductors, and such conductors have recently become much more widely available from TF materials suppliers.
Table 21. Advantages and issues of Bi in conductors and component metallisations.
The case of solar cell front side Ag metallisations is rather special: the traditionally PbO bearing glass frit must dissolve the antireflective layer (normally SiNy) and ideally create a good ohmic contact with the underlying n-type silicon emitterCitation43,Citation50 in a highly non-equilibrium dissolution–oxidoreduction–precipitation process. The overall reaction sequence has been shown to involve dissolution of Ag into the glass and reduction by Si to form crystallites, but the exact conduction mechanism – some direct connections or only tunnelling in a similar fashion to TFRs (next section) and the role of PbO in the frit are still somewhat elusive.Citation43 Nevertheless, successful substitution of PbO by Bi2O3 has been demonstrated recently, with presence of Ag ions in the glass enhanced by prior incorporation into the frit as Ag2O (Jeo-1, or Jeo-1' if Al2O3 and B2O3 mistakenly inverted in the paper, as is likely)Citation53 or by firing in pure oxygen.Citation52 These results allow removal of lead from what has become a high volume industry.
Glasses for TFRs
Current ‘cermet’ TFR compositions consist of a granular conductive phase dispersed in a glassy insulating matrix,Citation59,Citation61,Citation62,Citation461–Citation465 with additives to control the electrical properties;Citation466,Citation467 conduction occurs by percolation through the conductive grain network, with intergrain transport through a nanometre thin glass film, thought to occur by tunnelling, essentially accounting for the overall resistivity ().Citation84,Citation463,Citation468–Citation474 In classical TFR series, the conductive phase is mainly ruthenium oxide (RuO2) and/or its pyrochlore compounds with PbO and Bi2O3, lead and bismuth ruthenate (Pb2Ru2O7−y and Bi2Ru2O7), and the glassy matrix is a high lead lead borosilicate glass ().Citation59,Citation62,Citation475 Ruthenates give a higher resistivity than RuO2,Citation423 and the composition of the glass also has a strong effect, both on the stability of ruthenates versus RuO2 and directly on the resistivity.Citation464,Citation476 Alternative conductive phases for resistors can be based on IrO2 (properties similar to RuO2, more expensive,)Citation477,Citation478 and compounds with CaO, SrO and BaO, which have the perovskite structure, are also possible.Citation155 The synthesis and properties of RuO2, IrO2, their pyrochlore compounds and similar substances have been extensively studied.Citation155,Citation203,Citation479–Citation489 They belong to the class of electrically conductive oxides, which have been (very) extensively reviewed.Citation490
Figure 11. Microstructure and conduction mechanism of TFRsCitation1
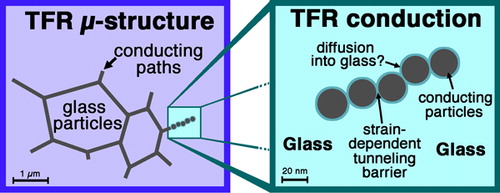
Figure 12. Typical composition (without temporary vehicle/binder) of TFRs with lead borosilicate glass matrixCitation1
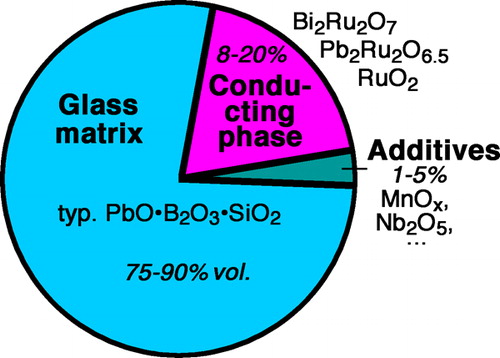
Such a complex resistor chemistry suggests possible particle glass chemical reactions, as Bi2O3 and PbO may be both in the glass and in the particles, and the configuration before firing may not be the equilibrium one. This is common in commercial systems, which frequently combine (Pb,Bi)2Ru2O7−y as the (initial) conductive phase dispersed in lead borosilicate glass, giving rise to exchange of Bi2O3 and PbO between the glass and the conducting phase as well as decomposition reactions.Citation61,Citation62,Citation465,Citation491,Citation492 One may write the following main chemical equilibria(1)
(2)
(3)
(4) where [g] is glass phase, [r] is conductive particles: rutile RuO2 (or IrO2) phase and [p] is conductive particles: pyrochlore ruthenate (or iridate) phase.
Equation (1) relates to the equilibrium between the pyrochlore ruthenate phase and the simple rutile oxide, and equation (2) describes the equilibrium composition of the pyrochlore phase relative to that of the glass; equations (3) and (4) relate to dissolution into/precipitation from the glass, which are quantitatively small due to the low solubility of RuO2 in the glass,Citation493,Citation494 but may nevertheless play an important role in the electrical properties.Citation495 Reactions (1) and (2) have indeed been observed experimentally,Citation61,Citation62,Citation465,Citation491 and a detailed study, for PbO only, established lead ruthenate was stable/formed only at moderate temperatures in glasses with lead concentrations much higher than that used in commercial systems;Citation464 this was confirmed in our studies on model RuO2 glass TFRs.Citation85,Citation496 Therefore, most ruthenate based formulations are not at thermodynamic equilibrium during firing. However, even in model systems where the conducting phase is thermodynamically stable (in practice, those formulated using RuO2), the glass composition strongly influences the resistivity.Citation476,Citation496
It must be additionally noted that the oxygen stoichiometry of Pb2Ru2O7−y is reported to be x = 0·5,Citation483,Citation484 which implies an average valence of +2·5 for Pb (or +4·5 for Ru?) in this compound. This adds a further complication, as formation/decomposition of lead ruthenate may involve a change of the oxidation state of Pb, requiring exchange of oxygen with the firing atmosphere, an implication supported by results of TFR annealing studies in different atmospheres.Citation497,Citation498 On the other hand, Bi and Ru essentially have the same valence in Bi2Ru2O7 as in the individual oxides Bi2O3 and RuO2; while Bi2Ru2O7 transforms at low temperatures to Bi3Ru3O11,Citation460,Citation499 formally linked with a slight oxidation, this transformation is apparently not observed in TFRs, and does not involve a change of the Bi/Ru stoichiometry (Bi2Ru2O7 made by high temperature synthesis is actually reported to be slightly nonstoichiometric, Bi2Ru2O6·9 or Bi1·9Ru2O6·9 (see the section on ‘Coordination of bismuth in crystalline oxides’ in Supplementary Material 1 http://dx.doi.org/10.1179/1743280412Y.0000000010.S1), but this does not necessarily apply to ‘resistor grade’ nanopowders calcined at lower temperatures,Citation483,Citation488 whose stoichiometry would require further study).
Therefore, it would seem logical to use a Bi based glass in order to enhance resistor stability to firing, given the otherwise similar properties to Pb based glasses. This would also lessen the property changes that classical resistors formulated with Pb based frits encounter when fired with Bi containing terminations.Citation79,Citation500 Therefore, it is not surprising that several such compositions have been patentedCitation251 (, Hm). Nevertheless, making the switch is not trivial, given the intricacies of TFR chemistry. Recently, first encouraging results, some of which by our group, have been published on lead free TFRs with bismuth based glasses and RuO2 conducting particles,Citation1,Citation70,Citation322,Citation323 for both standard 850°CCitation251,Citation322 and relatively low (∼600°C) firing temperaturesCitation1,Citation70,Citation323 (see also , Hm/Hy/L-Bi12). The former is useful for application as standard TFRs, while the latter may be used for low firing compositions suitable for firing onto glass or high strength temperature sensitive metallic alloys to fabricate high performance force and pressure sensors.Citation1,Citation68,Citation69,Citation71 An example of our results is given in , for resistors consisting of L-Bi12 glass with 11 mass-%RuO2 (∼9 vol. %) without additives. The results are very encouraging, featuring reproducible properties at firing temperature ⩾550°C and moderate, normal termination effects, i.e. without the increase of resistivity at the terminationsCitation77,Citation79 seen with the equivalent lead bearing systems.Citation69
Figure 13. Sheet resistivity at 25°C and temperature coefficient (HTCR, 25–100°C), versus length and firing temperature, of experimental TFRs fired on Al2O3 with Ag terminationsCitation1
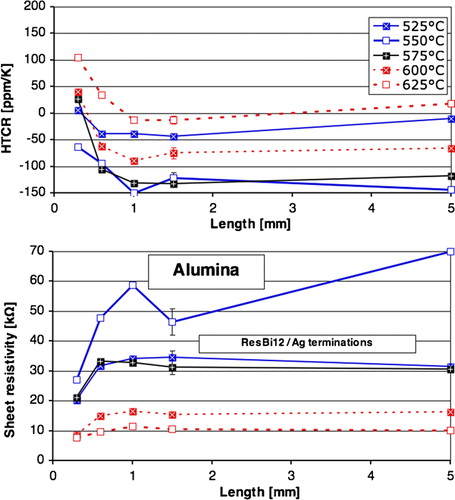
Several aspects must be studied carefully for Bi2O3 based glasses to become a standard matrix for TFRs. First, thermodynamic properties of RuO2, Bi2Ru2O7 and related compounds in regard to Bi based glasses must be known, especially the solubility of RuO2 in glass and the equilibrium between oxide and ruthenate, as was investigated in the lead based systems.
Additionally, as oxides of transition metals such as Ti, Zr, Nb, Mn, Fe, Cu and Zn are often added to control the temperature coefficient of resistance, it is important to know their possible interactions with Bi2O3 and RuO2. This has been the object of several studies, in pure oxide form, for ZnO,Citation501 TiO2,Citation502, CuO,Citation503 and NiO.Citation504 The Bi2Ru2O7 phase has been shown to be able to incorporate a significant amount of divalent transition metals (MO) as nominally (Bi2−xMx)Ru2O7−x/2 solid solutions, which seems to be a major difference with Pb2Ru2O7−y, where such solid solutions were not detected with CuO.Citation505 For tetravalent TiO2, there is some disagreement as to the extent of Bi2(Ru,Ti)2O7 solid solutions.Citation482,Citation502 Additionally, one must note that these studies only give clues to the observed behaviour in real TFR systems, because a) the activities of the oxides are different when dissolved in glass, and b) TFRs are usually non-equilibrium systems.
A more long term potential development would be the avoidance of costly precious metals, by using other metallic (or almost metallic) oxides and compounds as conductive phases, of which a very wide variety exists,Citation490 or metal borides, silicides, etc. To circumvent the often observed processing issues with nitrogen firing TFRs,Citation183–Citation189,Citation190,Citation506 air firing systems should be developed. Several promising studies using molybdenum oxides have been carried out towards this goal,Citation507–Citation512 with the most recent embodimentCitation512 having achieved transition to a bismuth glass frit, paving the way to low cost lead free resistors. These compositions are air fireable, because the conductive phase, MoO2 and/or other intermediate oxides (which are unstable in air) form in situ, after densification of the glass frit, by reduction of MoO3. This is brought about by a suitable reducing agent, usually boron. Another base metal conductive filler successfully investigated with bismuth glasses is SnO2/Sb,Citation166 which seems, however, more suitable for higher ranges of surface resistance (>100 kΩ), in line with the semiconductive properties of this filler.
Other applications
Optics
Heavy metal ions such as Bi3+, Pb2+ and Tl+ feature a unique combination of properties: high mass and polarisability, together with low bond strength. For optical applications of HMO materials, be it in glassy, ceramic or single crystal form, this translates into high refractive indices n (), dispersion and optical non-linear susceptibility χ(3), as well as low phonon energies.Citation36,Citation112,Citation124,Citation128,Citation329,Citation371 In addition, low levels of partly reduced Bi in both Bi based and other glasses have been found to display broad IR luminescence, with possible application to optical amplificationCitation353,Citation428–Citation430 (see also discussion in the section on ‘Oxidation state of Bi species in glasses’ in Supplementary Material 1 http://dx.doi.org/10.1179/1743280412Y.0000000010.S1). Glasses of HMO are a promising alternative to fluorides,Citation37,Citation192,Citation193 due to their better chemical durability.
Table 22. Optical properties of HMO glasses
Infrared cut-off wavelength is related to the highest frequency bond vibrations/maximum phonon energy. Therefore, HMO glasses for light amplification, and especially for far IR transmission, should contain no standard network formers (B2O3, SiO2, P2O5) or light intermediates with strong bonding such as Al2O3. Although GeO2 constitutes a good drop-in replacement for SiO2, much better IR performance can be obtained () with non-standard formers such as Ga2O3 in the PbO–Bi2O3–Ga2O3 systemCitation36,Citation371 and TeO2 in Bi2O3–TeO2–WO3,Citation123 and even systems entirely without glass formers such as Li2O–[BaO/PbO]–Bi2O3Citation381,Citation382,Citation388 and SrO–PbO–Bi2O3.Citation117 Considerable care must be exercised when melting Bi containing glasses for optical applications, as they are quite susceptible to reduction by trace organics or excessive melting temperatures (the section on ‘Oxidoreduction issues’), and to contamination by crucibles.Citation36,Citation387 Reduction or Pt pickup leads to darkening/staining of the glass, while even relatively low contamination by oxides such as SiO2 and Al2O3 will introduce high frequency vibrations into the network, degrading far IR transmission.
Obtaining a high refractive index and non-linear susceptibility, on the other hand, only involves the average glass properties, allowing limited use of standard network formers and thereby greatly facilitating vitrification. Synergistic vitrification through mixing of SiO2, B2O3 and Al2O3 and substituting some Bi2O3 by ZnO (the section on ‘Glass formation’) allows maximisation of HMO content; recent results on glasses optimised this wayCitation116 yield n = 2·31 at 633 nm and χ(3) = 4·9×10−11 esu at 748 nm. The glass composition was not specified exactly, but mentioned as Bi2O3–B2O3–SiO2, and is probably close to Smt-7 (). The high and very fast response of these glasses makes them very promising for high frequency fully optical switching.Citation112
In the case of upconversion, recent measurementsCitation119,Citation371 indicate that average properties (phonon density) rather than maximal phonon energy dominates efficiency, which is supported by the good results achieved by others with doped/codoped HMO glasses containing a low amount of network formers,Citation121,Citation124,Citation125,Citation325,Citation370 usually GeO2 given its lower impact on performance. An additional advantage of Bi2O3 based HMO glasses for this application is their good compatibility with lanthanide additions (the section on ‘Glass formation’).
Finally, the above considerations, though essentially focussed on glasses, are also applicable to similar crystalline (single crystal, ceramic or glass–ceramic) compounds, of which many exhibit promising optical properties.Citation351
γ-ray protection glasses
Due to their ideal combination of high γ-ray absorption coefficient (imparted by their large atomic mass) and good glass forming ability as oxides, Pb and Bi are useful in γ-ray absorbing windows in the nuclear industry and high energy physics.Citation129–Citation135 Requirements for these glasses are in general similar to that for nonlinear optics: a HMO glass is needed for a good attenuation factor, but moderate concentrations of traditional network formers (B2O3 and SiO2) are allowed, facilitating fabrication of the thick parts needed for good shielding.
An early study of was carried out by Brekhovskikh in the PbO–Bi2O3–SiO2 system;Citation129 ternary glasses down to ∼17%SiO2 and with density >8000 kg m−3 could be manufactured (Rad-1), but practical considerations (need for casting in thick sections requires ∼30%SiO2, Rad-2). An analogous borate glass (Rad-4, ∼21%BO1·5) was formulatedCitation130, but no indication was given on its castability in thick sections. The claim of a Bi2O3–SiO2 glass with ∼95 cation-%BiCitation131 is dubious, as the indicated density of 7284 kg m−3 corresponds to ∼70%Bi,Citation248 In the light of the section on ‘Glass formation’, maximising HMO content in a Bi based Pb free glass would most likely best be achieved in a more complex system, such as SrO–BaO–ZnO–Bi2O3–Al2O3–B2O3–SiO2. Compared to PbO, γ-ray attenuation coefficients with Bi2O3 are similarCitation129,Citation134 (the apparent observed increase when substituting PbO with Bi2O3Citation130 is simply due to the ‘molecular’ formulation, where Bi is counted twice versus Pb), yielding a progressive increase in HMO content from 69 to 79% on a cation basis.
This said, the main issue with γ-ray protection glasses lies with radiation induced darkening, for which results are relatively unclear.Citation131–Citation133,Citation135 Significant darkening in the short wavelength portion of the visible spectrum was observed after 2·5 kGy from Citation60Co, but no systematic trend versus composition was observed for Bi2O3–B2O3 glasses,Citation133 which is also the case for Bi2O3–SiO2 glasses after 10, 20 and 40 kGy Citation60Co.Citation131 This darkening on the other hand was absent or very low in the visible for Bi2O3–B2O3 glasses optionally doped with CuOy after up to 50 kGy Citation60Co.Citation132 Although these latter results are very encouraging, systematic studies of the mechanisms of radiation induced damage and of the good resistance against it of some glasses, including doping/impurity effects, still need to be carried out to reliably achieve low darkening Bi based glasses.
Bi2O3 in (glass–)ceramics and crystals
A short discussion is given in this section of Bi2O3 in five types of crystalline materials:
i. | piezoceramics | ||||
ii. | ionic and mixed conductors with high oxygen mobility | ||||
iii. | high temperature superconductors | ||||
iv. | scintillators | ||||
v. | ferroelectric memories. |
Besides these applications, Bi2O3 is a component of many electroceramics, in both the active crystalline phase or as a sintering aid,Citation9 and compounds such as bismuth ruthenates (Bi2Ru2O7/Bi3Ru3O11), besides being a useful conductive phase for TFRs (see the section on ‘Glasses for TFRs’), are envisioned as chemical catalysts and electrodes.Citation499,Citation513 Bismuth vanadate (BiVO4) is a relatively new yellow pigment that provides an alternative to toxic Pb and Cd based pigments,Citation514 and crystalline bismuth borates have interesting optical properties,Citation351 akin to the corresponding glasses (see the section on ‘Optics’). A quite extensive list of Bi2O3 based compounds and corresponding applications is given in recent work,Citation403,Citation515 together with an extensive discussion on their structural features (some of which are also discussed in the section on ‘Coordination of bismuth in crystalline oxides’ in Supplementary Material 1 http://dx.doi.org/10.1179/1743280412Y.0000000010.S1, as they yield insight into the structure of the corresponding glasses). Finally, it is worth mentioning that derivatives of the analogous chalcogenide, Bi2Te3, are the dominant room temperature thermoelectric materials.Citation516
Piezoceramics
Besides glass, the main application of PbO bearing materials is arguably PZT = Pb(Zr,Ti)O3 and similar ceramics, mainly used for their large piezoelectric or electrostrictive properties. As these ceramics are present in many small essentially disposable electronic devices, considerable effort has been devoted to finding lead free substitutes with at least acceptable performance. Recently, complex mixtures of Bi2O3 perovskite compounds such as Bi0·5(Na,K)0·5TiO3 and Bi(Fe,Sc)O3 with (Ba,Sr)TiO3 and (Na,K)NbO3 have given rise to promising materials achieving strains comparable to that of the classical PZT ceramics, although many issues remain.Citation517,Citation518 Note that bismuth titanate (Bi4Ti3O12) is also a piezoceramic, albeit a specialised, high temperature and low activity one.
Ionic and mixed ionic electronic conductors with high oxygen mobility
The high temperature disordered defect fluorite cubic δ-Bi2O3 phase contains a very large amount of disordered oxygen vacancies, it may in fact be written as Bi(O3/4□1/4)2, i.e. with a quarter of the oxygen anion sublattice randomly empty, and therefore exhibits exceptionally high ionic conductivity. Although δ-Bi2O3 is only stable above 730°C, Takahashi et al.Citation519 established that this disordered phase may be stabilised at lower temperatures, much like cubic zirconia, by various dopants, thus giving birth to a new family of solid electrolyte materialsCitation519–Citation522 with high ionic conductivity at moderate temperatures. For instance, Bi2O3 solid solutions stabilised by oxides such as Y2O3 or Er2O3 have ionic conductivities exceeding 1 S m−1 at 500°C, more than 20× that of cubic or tetragonal zirconia, and more recent ‘BIMEVOX’ materials with an oxygen deficient Aurivillius structure have even higher conductivity. However, as all Bi2O3 based materials, these ionic conductors have limited stability towards reduction, and their use is limited in solid oxide fuel cell (SOFC) applications. Nevertheless, they may still be used to this end, provided the reduction potential is limited or a thin protective layer of another electrolyte material is used on the reducing side. In addition, Bi2O3 ionic and mixed ionic electronic conductors may find other important applications such as air side electrodes for SOFCs, chemical electrodes, oxygen sensing and oxygen extraction from air.Citation521,Citation523
High temperature superconductor materials
The discovery of high temperature (>100 K) superconductivity in the Bi–Sr–Ca–Cu oxide (‘BiSCCO’) family (with possible substitution of Bi by Pb)Citation99,Citation524,Citation525 having low melting points opened up the possibility of greatly easier processing of these materials. In fact, these high temperature superconductor compounds have compositions (examples in ) corresponding to the ‘heavy metal oxide’ glasses,Citation36 and are therefore amenable to processing by the vitroceramic route, i.e. glass melting and quenching, followed by shaping and finally crystallisation and annealing to yield the superconducting phaseCitation39,Citation99,Citation101,Citation102,Citation104,Citation105 with zero resistance temperatures in excess of 100 K under optimal conditions.Citation101 Low concentrations of suitable dopants do not significantly enhance the superconducting properties, but are added to facilitate processing and yield more consistent products. Reported melting temperatures are in the 1100–1300°C range, with long term annealing around 850°C to form the superconducting phase(s). These materials feature rather high resistivity in their glassy state,Citation100 in contrast to amorphous Bi3Ru3O11.Citation499
Table 23. Compositions of selected BiSCCO superconductors, given in cation molar proportions [z = Pb/(Pb+Bi)]
Besides these materials, doping BaBiO3 (with Bi in mixed +3 and +5 valence) with K or Pb (Ba,K)BiO3 or Ba(Bi,Pb)O3 respectively, yields superconductors with transition temperatures up to 30 K that are of theoretical interest due to their simple cubic structure.Citation526
Scintillators
In addition to heavy glasses potentially improving scintillation efficiency,Citation36 crystals such as Bi4Ge3O12, Bi4Si3O12 and their solid solutionsCitation368,Citation527,Citation528 are useful as γ-ray scintillators, the active ion in this case being directly Bi3+ (i.e. ‘self-activated’). Potential preparation of Bi4Ge3O12 by the glass–ceramic route has been explored.Citation368
Ferroelectric memories
Besides being useful as a piezoceramic, PZT in thin film form is also of interest for non-volatile ferroelectric memories. However, it was soon realised that its sensitivity to so called fatigue, i.e. degradation upon repeated switching, was an important, although alleviated by the use of oxide electrode contacts.Citation529 This problem was largely solved by the introduction of Aurivillius type layered perovskite compounds derived from Bi4Ti3O12 such as Bi3·25La0·75Ti3O12 (simple substitution with La),Citation530 SrBi2Ta2O9Citation531 or SrBi4Ti4O15,Citation532 which feature vastly improved switching endurance.
Conclusions
The many examples discussed in this review show that replacement of lead by bismuth in TF and related glass frits seems the most feasible route in the short to middle term. In fact, this is already happening in several important industries such as electronics, displays and automotive/architectural glass, in spite of the still considerable technological issues. Moreover, promising new applications are currently undergoing development in the sensors and optics field.
The considerably higher price of bismuth, ∼10 times that of leadCitation533 should not constitute a problem in many high added value applications, where a relatively low overall volume of material is used, such as TF electronics, sensor cells and specialised optics. Moreover, Bi is not expected to replace lead in high volume applications such as vehicle batteries, and only to a very modest extent in electronic solders, Bi–Sn and similar low melting eutectic solder having only limited use. The same applies for use of Pb–Bi eutectic (LBE) coolants.Citation422
For TF electronics, the very strong increase in prices of all noble metals (Ag, Pd, Pt, Ru) in recent timesCitation533 is therefore a much more pressing issue for conductor and resistor materials. Development should be concentrated on new materials which reduce or altogether avoid their use, as has already been initiated for resistors.Citation166,Citation512 On the other hand, the price of Bi can be a factor in high volume and very cost sensitive architectural and automotive applications,Citation47 so further developments to reduce its use in these sectors are likely.
From a scientific point of view, more well controlled experimental data is necessary for bismuth based glasses, as the reported results, especially on glass stability and properties, are somewhat conflicting, even for the simplest systems. Detailed studies on devitrification, separately assessing the composition dependent dynamics of crystal nucleation and growth, are strongly needed as well, especially in the practically important low melting borosilicates and HMO gallate systems.
Also, the very disordered structure Bi3+ adopts within the glass network, and how it is influenced by glass composition and thermal history, requires further clarification, especially in the light of recent structural studiesCitation403 on the peculiar behaviour of ‘lone pair’ ions such as Bi3+, Sb3+, Pb2+ and Sn2+ (see also the section on ‘Coordination of bismuth in crystalline oxides’ in Supplementary Material 1 http://dx.doi.org/10.1179/1743280412Y.0000000010.S1). Apparently, PbO based glasses and two important envisioned substitutes, those based on Bi2O3 and on SnO, share fundamental structural features. Extending the detailed structural studies and computer modelling to glasses would lead to a clearer picture of how glass formation and structure are affected by the stereochemically active lone pair, the valence and the polarisability.
Finally, it must be noted that this review has only dealt with relatively standard glassmaking techniques of Bi based glasses; chemical methods such as solution coating of powders, nanopowder synthesis or sol–gel castingCitation534 constitute promising alternative routes, potentially allowing the manufacture of novel glasses and glass matrix composites with a degree of control not achievable by standard methods.
yimr_a_11743737_sm0001.pdf
Download PDF (1.9 MB)The author gratefully acknowledges partial financial support from the Swiss National Science Foundation NCCR CO-ME programme, as well as the assistance and patience of present and former colleagues at EPFL, especially C. Jacq and M. Garcin for experimental work and help from N. Takano and I. Stolichnov with Japanese- and Russian-only sources. The coordination shell drawings were created with the Avogadro molecular editing software.
References
- Maeder T, Jacq C, Grimaldi C, Ryser P: ‘Lead-free low-firing thick-film resistors based on bismuth glasses and ruthenium oxide’, Proc. XXXIII Int. Conf. of IMAPS Poland Chapter, Gliwice–Pszczyna, Poland, September 2009, IEEE, 222–229.
- Donald IW: ‘Inorganic glasses and glass-ceramics: a review’; 1984, Aldermaston, Atomic Weapons Research Establishment.
- Bansal N, Doremus R: ‘Handbook of glass properties’; 1986, Orlando, FL, Academic Press.
- Volf MB: ‘Glass Sci. Technol. 10: a technical approach to glass’; 1990 , Amsterdam, Elsevier.
- Kurkjian CR, Prindle WR: ‘Perspectives on the history of glass composition’, J. Am. Ceram. Soc., 1998, 81, (4), 795–813.
- Boyd DC, Danielson PS, Thompson DA, Velez M, Reis ST, Brow RK: ‘Glass’, in ‘Kirk–Othmer encyclopedia of chemical technology’, 5th edn, 565–626; 2005, Hoboken, Wiley.
- Donald I: ‘Novel glasses and their applications’, Discovery, 2001, (2), 2–9.
- Donald I: ‘Methods for improving the mechanical properties of oxide glasses’, J. Mater. Sci., 1989, 24, (12), 4177–4208.
- Sebastian MT, Jantunen H: ‘Low loss dielectric materials for LTCC applications: a review’, Int. Mater. Rev., 2008, 53, (2), 57–90.
- Leiser C: ‘Importance of lead in glass – Part one’, Glass Indust., 1962, 44, 509–513.
- Leiser C: ‘Importance of lead in glass – Parts two/III (conclusion)’, Glass Indust., 1963, 45, 574–576; 630–632.
- Rabinovich E: ‘Lead in glasses’, J. Mater. Sci., 1976, 11, 925–948.
- Bobkova NM: ‘Low-melting glasses based on lead-borate systems (review)’, Glass Ceram., 2009, 66, (5–6), 206–209.
- Scott W: ‘Glass-to-metal seal design’, J. Sci. Instrum., 1946, 23, (9), 193–202.
- Broukal J: ‘Beitrag zur Untersuchung der in der Vakuumelektronik verwendeten Sondergläser (Glaslote) [Contribution to the investigation of the special glasses used in vacuum electronics (sealing glasses)]’, Silikattechnik, 1962, 13, (12), 428–433.
- Broukal J: ‘Thermisch entglasbare Glaslote [Thermally devitrifying sealing glasses]’, Silikattechnik, 1966, 17, (8), 242–248.
- Frieser R: ‘A review of solder glasses’, Electrocompon. Sci. Technol., 1975, 2, 163–199.
- Paschke H: ‘Die Anwendung von Glasloten [The application of solder glasses]’, DVS Berichte, 1980, 66, 177–178.
- Rabinovich E: ‘Solder glasses and vitreocrystalline cements’, Inorg. Mater., 1976, 11, 925–948.
- Donald IW: ‘Preparation, properties and chemistry of glass-ceramic-to-metal seals and coatings’, J. Mater. Sci., 1993, 28, (11), 2841–2886.
- Monneraye M: ‘Les encres sérigraphiables en microélectronique hybride: les matériaux et leur comportement [Screenable inks in hybrid microelectronics: the materials and their behaviour]’, Acta Electron., 1978, 21, (4), 263–281.
- Hoffman L: ‘An overview of thick film hybrid materials’, Am. Ceram. Soc. Bull., 1984, 63, (4), 572–576.
- Wada T, Stein S, Stein M, Chitale S: ‘The state-of-the-art of thick film technology for automotive sensors’, Proc. 1st 1997 IEMT/IMC Symp., Tokyo, Japan, April 1997, IEEE, 41–46.
- Sergent JE: ‘Hybrid microelectronics technology’, in ‘Electronic systems maintenance handbook’, (ed. , Whitaker J C, 2nd edn, Chap. 11; 2002, Boca Riton, FL, CRC Press.
- Pitt K, (ed.): ‘Handbook of thick film technology’, 2nd edn; 2005, Isle of Man, Electrochemical Publications.
- White NM: ‘Advances in thick-film sensors’, Proc. 14th Int. Conf. on ‘Solid-state sensors, actuators and microsystems’, Lyon, France, June 2007, IEEE, MINATEC, 107–111.
- Jacq C, Maeder T, Ryser P: ‘Sensors and packages based on LTCC and thick-film technology for severe conditions’, SĀDHANĀ, 2009, 34, (4), 677–687.
- Jacq C, Maeder T, Ryser P: ‘High-strain response of piezoresistive thick-film resistors on titanium alloy substrates’, J. Eur. Ceram. Soc., 2004, 24, (6), 1897–1900.
- Shimbo M, Tai S, Tanzawa K: ‘Glass formation range, acid resistivity, and surface charge density of ZnO–B2O3–SiO2 passivation glass containing Al2O3’, J. Am. Ceram. Soc., 1986, 69, (1), 23–26.
- Shimbo M, Furukawa K: ‘Physical and electrical properties of acid resistive zinc–lead borosilicate passivation glass’, J. Ceram. Soc. Jpn Int Edn, 1988, 96, 201–205.
- Bobkova NM: ‘Glass enamels in the electronics industry’, Glass Ceram., 1995, 52, (5), 107–111.
- Gerasimov VV, Spirina OV: ‘Low-melting borosilicate glazes for special purpose and construction ceramics (a review)’, Glass Ceram., 2004, 61, (11–12), 382–388.
- Heider GL: ‘Bismuth key to lead-free success’, Glass, 1992, 69, (7), 273–275.
- Yashchishin IN, Babadzhanova OF, Vasiichuk VA: ‘Low-melting coatings for glass decoration’, Glass Ceram., 2001, 58, (7–8), 287–289.
- Leveaux M, Crevit N, Lips K, Schepers B, Aerts A, Verboom E: ‘Nuovi orizzonti nella smaltatura dell’alluminio [New horizons in the enamelling of aluminium]’, Smalto Porcellanato, 2002, 44, (2).
- Dumbaugh WH, Lapp JC: ‘Heavy-metal oxide glasses’, J. Am. Ceram. Soc., 1992, 75, (9), 2315–2326.
- Rault G, Adam J, Smektala F, Lucas J: ‘Fluoride glass compositions for waveguide applications’, J. Fluor. Chem., 2001, 110, 165–173.
- Stroganova E, Mikhailenko N, Moroz O: ‘Glass-based biomaterials: present and future’, Glass Ceram., 2003, 70, (9–10), 315–319.
- Shishkov NV: ‘Superconducting glass ceramics in the Bi–Sr–Ca–Cu–O system – a review’, Sci. Ceram. Indust., 1992, 9, 16–20.
- Erickson D, Li DQ: ‘Integrated microfluidic devices’, Anal. Chim. Acta, 2004, 507, (1), 11–26.
- Schneider MA, Maeder T, Ryser P, Stoessel F: ‘A microreactor-based system for the study of fast exothermic reactions in liquid phase: characterization of the system’, Chem. Eng. J., 2004, 101, 241–250.
- Cheek GC, Mertens RP, Van Overstraeten R, Frisson L: ‘Thick-film metallization for solar cell applications’, IEEE Trans. Electr. Dev., 1984, 31, (5), 602–605.
- Schubert G, Huster F, Fath P: ‘Physical understanding of printed thick-film front contacts of crystalline Si solar cells – review of existing models and recent developments’, Solar Energy Mater. Solar Cells, 2006, 90, (18–19), 3399–3406.
- Krüger R, Roosen A, Schaper W: ‘Hermetic glass sealing of AlN packages for high temperature applications’, J. Eur. Ceram. Soc., 1999, 19, 1067–1070.
- Geodakyan D, Petrosyan B, Stepanyan S, Geodakyan K: ‘Investigation of the possibility of replacing high-lead glasses in fusible glass solders by less toxic glasses’, Glass Ceram., 2009, 66, (11–12), 381–384.
- Shinkareva EV: ‘Lead free enamels for decoration of glass articles’, Glass Ceram., 2004, 61, (9–10), 303–305.
- Sakoske GE: ‘Functional glass coatings (presentation)’, Proc. IMI Int. Workshop on ‘Scientific challenges of new functionalities in glass’, Washington, DC, USA, April 2007, IMI.
- Fredericci C, Yoshimura HN, Molisani AL, Fellegara H: ‘Effect of TiO2 addition on the chemical durability of Bi2O3–SiO2–ZnO–B2O3 glass system’, J. Non-Cryst. Solids, 2008, 354, (42–44), 8592–8598.
- Needes CR: ‘Thick film silver metallizations for silicon solar cells’, Patent US4235644, 1980-11, 1980.
- Ballif C, Huljic DM, Willeke G, Hessler Wyser A: ‘Silver thick-film contacts on highly doped n-type silicon emitters: structural and electronic properties of the interface’, Appl. Phys. Lett., 2003, 82, (12), 1878–1880.
- Wang YL, Hang KW: ‘Electroconductive thick film composition(s), electrode(s), and semiconductor device(s) formed therefrom’, Patent US2006/0231803, 2006.
- Cho SB, Hong KK, Chung BM, Huh JY: ‘Influence of firing ambience on fire-through silver contact metallization for crystalline silicon solar cells’, Proc. 34th IEEE Photovoltaic Specialists Conf., Philadelphia, PA, USA, June 2009, IEEE, 766–769.
- Jeon SJ, Koo SM, Hwang SA: ‘Optimization of lead- and cadmium-free front contact silver paste formulation to achieve high fill factors for industrial screen-printed Si solar cells’, Solar Energy Mater. Solar Cells, 2009, 93, (6–7), 1103–1109.
- Eliseev SY, Rodtsevich SP, Lukashevich GF: ‘A low boron dielectric coating for aluminum’, Glass Ceram., 2000, 57, (11–12), 402–403.
- Yatsenko EA, Zubekhin AP, Shkurakova EA: ‘Tinted low melting enamels for aluminum’, Glass Ceram., 2001, 58, (11–12), 428–430.
- Leveaux M, Humez S: ‘Particular compositions of porcelain enamels’, Patent EP1331207, 2003.
- Monzio Compagnoni A, Ferraro A: ‘Smaltatura dell’aluminio ad alto tenore di magnesio [enamelling of aluminium with high magnesium content]’, Smalto Porcellanato, 2005, 47, (2).
- Monzio Compagnoni A: ‘Riduzione o eliminazione del vanadio nello smalto per alluminio [Reduction or elimination of vanadium in enamels for aluminium]’, Smalto Porcellanato, 2006, 48, (1).
- Vest R: ‘Materials science of thick film technology’, Am. Ceram. Soc. Bull., 1986, 65, (4), 631–636.
- Dell’Acqua R: ‘Substrates for thick film technology’, in ‘Thick film sensors’, (ed. , Prudenziati M, 59–72; 1994, Amsterdam, Elsevier.
- Shah J, Hahn W: ‘Material characterization of thick film resistor pastes’, IEEE Trans. Compon. Hybrids Manuf. Technol., 1978, CHMT-1, (4), 383–392.
- Prudenziati M, Dell’Acqua R: ‘Thick film resistors’, in ‘Thick film sensors’, 85–97; 1994, Amsterdam, Elsevier.
- Dell'Acqua R: ‘Thick films conductors’, in ‘Thick film sensors’, (ed. , Prudenziati M, 73–83; 1994, Amsterdam, Elsevier.
- Wang SF, Dougherty JP, Huebner W: ‘Silver-palladium thick-film conductors’, J. Am. Ceram. Soc., 1994, 77, (12), 3051–3072.
- Dell'Acqua R: ‘Thick film dielectric materials’, in ‘Thick film sensors’, (ed. , Prudenziati M, 99–111; 1994, Amsterdam, Elsevier.
- Hsieh YH, Fu SH: ‘BaPbO3-based thick film resistor’, IEEE Trans. Compon. Hybrids Manuf. Technol., 1992, 15, (3), 348–352.
- Jacq C, Maeder T, Martinerie S, Corradini G, Carreño M, Ryer P: ‘High performance thick-film pressure sensors on steel’, Proc. 4th Eur. Microelectronics and Packaging Symp., Terme Catez, Slovenia, May 2006, IMAPS, 105–109.
- Jacq C, Maeder T, Ryser P: ‘Piezoresistive properties of low-firing temperature thick-films on steel sensors’, Proc. 4th Int. Conf. on ‘Ceramic interconnect and ceramic microsystems technologies’, Munich, Germany, April 2008, IMAPS, 411–416 (P413).
- Jacq C, Maeder T, Ryser P: ‘Load sensing surgical instruments’, J. Mater. Sci., 2009, 20, (S1), S223–S227.
- Totokawa M, Yamashita S, Morikawa K, Mitsuoka Y, Tani T, Makino H: ‘Microanalyses on the RuO2 particle–glass matrix interface in thick film resistors with piezoresistive effects’, Int. J. Appl. Ceram. Technol., 2009, 6, (2), 195–204.
- Maeder T, Jacq C, Ryser P: ‘Low-firing thick-film piezoresistive sensors for medical instruments’, Sens. Actuators A, 2011, 172A, (1), 228–232.
- Kaneko I, Tagushi S, Kashiwagi T: ‘Metal-glazed thick-film resistors fired at low temperature on glass substrate’, IEICE Trans. Electron., 2000, E83 C(10), 1669–1676.
- Sack W, Scheidler H, Petzoldt J: ‘Zum Kristallisationsverhalten der Glaslote [On the crystallisation behaviour of sealing glasses]’, Glastechnische Berichte, 1968, 41, (4), 138–145.
- Hinz W, Solow G: ‘Bemerkungen zu entglasenden Verbindungsgläsern [Remarks on devitrifying sealing glasses]’, Silikattechnik, 1962, 13, (8), 272–278.
- Bobinski JG: ‘Low temperature sealing glass’, Patent US6248679, 2001–06, 2001.
- Prudenziati M, Morten B, Cilloni F, Ruffi G, Sacchi M: ‘Interactions between alumina and high lead glasses for hybrid components’, J. Appl. Phys., 1989, 65, (1), 146–153.
- Prudenziati M, Morten B, Ruffi G, Argentino E, Iachetti C: ‘Size effect due to silver diffusion in RuO2-based resistors’, Proc. 7th Eur. Hybrid Microelectronics Conf., Hamburg, Germany, May 1989, ISHM, 162–169.
- Prudenziati M, Morten B, Cilloni F, Ruffi G: ‘Very high strain sensitivity in thick-film resistors: real and false super gauge factors’, Sens. Actuators, 1989, 19, (4), 401–414.
- Prudenziati M, Sirotti F, Sacchi M, Morten B, Tombesi A, Akomolafe T: ‘Size effects in ruthenium-based thick-film resistors: rutile versus pyrochlore-based resistors’, Act. Passive Electron. Compon., 1991, 14, (3), 163–173.
- Prudenziati M, Morten B, Savigni P, Guizzeti G: ‘Influence of the preparing conditions on the physicochemical characteristics of glasses for thick film hybrid microelectronics’, J. Mater. Res., 1994, 9, (9), 2304–2313.
- Prudenziati M, Morten B, Forti B, Gualtieri AF, Dilliway GM: ‘Devitrification kinetics of high lead glass for hybrid microelectronics’, Int. J. Inorg. Mater., 2001, 3, (7), 667–674.
- Hoffman LC, Horowitz SJ: ‘Stable pyrochlore resistor compositions’, Patent US4302362, 1981.
- Vionnet-Menot S, Grimaldi C, Maeder T, Ryser P, Strässler S: ‘Study of electrical properties of piezoresistive pastes and determination of the electrical transport’, J. Eur. Ceram. Soc., 2005, 25, (12), 2129–2132.
- Vionnet-Menot S, Grimaldi C, Maeder T, Ryser P, Strässler S: ‘Tunneling-percolation origin of nonuniversality: theory and experiments’, Phys. Rev. B, 2005, 71B, (6), 064201.
- Vionnet-Menot S: ‘Low firing temperature thick-film piezoresistive composites – properties and conduction mechanism’, PhD thesis, EPFL, Lausanne, Switzerland, 2005.
- Vionnet-Menot S, Maeder T, Grimaldi C, Jacq C, Ryser P: ‘Properties and stability of thick-film resistors with low processing temperatures – effect of composition and processing parameters’, J. Microelectron. Electron. Packag., 2006, 3, (1), 37–43.
- Hoffman LC: ‘Metallizing compositions containing critical proportions of metal (Pt–Au or Pd–Au) and a specific high density frit’, Patent US3440062, 1969.
- Zdaniewski W, Silverman L: ‘Effect of localized redox equilibria on adhesion between gold and thick-film dielectrics’, J. Mater. Sci., 1990, 25, (7), 3155–3158.
- Hynes M, Jonson B: ‘Lead, glass and the environment’, Chem. Soc. Rev., 1997, 26, 133–146.
- Hynes M, Forde S, Jonson B: ‘Element migration from glass compositions containing no added lead’, Sci. Total Environ., 2004, 319, (1), 39–52.
- Levin E M, Robbins C R, McMurdie H F, (eds): ‘Figures 1 2066’, Vol. 1; 1964, Columbus, OH, American Ceramic Society.
- Levin E M, Robbins C R, McMurdie H F, (eds): ‘Figures 2067 4149’, Vol. 2; 1969, Columbus, OH, American Ceramic Society.
- Levin E M, McMurdie H F, (eds): ‘Figures 4150-4999’, Vol. 3, 1975, Columbus, OH, American Ceramic Society.
- Roth R, Negas T, Cook (eds) L: ‘Phase diagrams for ceramists’, Vol. 4, 5000–5590; 1981, Columbus, OH, American Ceramic Society.
- Sales BC, Boatner LA: ‘Lead-iron phosphate glass: a stable storage medium for high-level nuclear waste’, Science, 1984, 226, (4670), 45–48.
- Karabulut M, Marasinghe K, Ray CS, Day DE, Ozturk O, Waddill GD: ‘X-ray photoelectron and Mössbauer spectroscopic studies of iron phosphate glasses containing U, Cs and Bi’, J. Non-Cryst. Solids, 1999, 249, (2–3), 106–116.
- Reis ST, Karabulut M, Day DE: ‘Chemical durability and structure of zinc iron phosphate glasses’, J. Non-Cryst. Solids, 2001, 292, (1–3), 150–157.
- Reis ST, Karabulut M, Day DE: ‘Structural features and properties of lead–iron–phosphate nuclear wasteforms’, J. Nucl. Mater., 2002, 304, (2–3), 87–95.
- Komatsu T, Sato R, Meguro H, Matusita K, Yamashita T: ‘Effect of copper content on glass formation and superconductivity in the Bi–Pb–Sr–Ca–Cu–O system’, J. Mater. Sci., 1991, 26, (3), 683–688.
- Som KK, Molla S, Bose K, Chaudhuri BK: ‘Nonlinear physical properties of amorphous Bi4Sr3Ca3CuyOx semiconducting oxides with y between 0 and 5’, Phys. Rev. B, 1992, 45, (4), 1655–1659.
- Tatsumisago M, Inoue S, Tohge N, Minami T: ‘Dopants in high Tc superconductors from rapidly quenched Bi1·6Pb0·4Ca2Sr2Cu3Ow glasses’, J. Mater. Sci., 1993, 28, (15), 4193–4196.
- Hu Y, Zheng H, Mackenzie JD: ‘High-Tc superconducting Bi(Al)–Ca–Sr–Cu–O glass-ceramic fibres drawn from glass preforms’, J. Mater. Sci., 1995, 30, (15), 3913–3918.
- Hu Y, Liu N, Lin U: ‘Glass formation and glass structure of the BiO1·5 PbO–CuO system’, J. Mater. Sci., 1998, 33, (1), 229–234.
- Gazda M: ‘The (Bi,Pb)4Sr3Ca3Cu4Ox glass–ceramics: disordered metal and superconductor’, J. Non-Cryst. Solids, 2006, 352, (40–41), 4246–4249.
- Gazda M, Kusz B, Murawski L: ‘Electronic conduction in (Bi,Pb)–Sr–Ca–Cu–O granular superconductors’, J. Non-Cryst. Solids, 2008, 354, (35–39), 4323–4325.
- Dumbaugh WH, Tyndell BP: ‘Bi2O3–Ga2O3 glasses’, Patent US4456692, 1984.
- Khalilov GS, Artamonova MV, Shaplygin IS: ‘Glass formation in bismuth-containing oxide systems’, Doklady Akademii Nauk SSSR, 1984, 276, (4), 908–910.
- Dimitriev Y, Mihailova V: ‘Glass formation in binary systems with Bi2O3 and PbO participation’, J. Mater. Sci. Lett., 1990, 9, 1251–1254.
- Miyaji F, Yoko T, Jin J, Sakka S, Fukunaga T, Misawa M: ‘Neutron and X-ray diffraction studies of PbO–Ga2O3 and Bi2O3–Ga2O3 glasses’, J. Non-Cryst. Solids, 1994, 175, (2–3), 211–223.
- Stehle C, Vira C, Hogan D, Feller S, Affatigato M: ‘Optical and physical properties of bismuth borate glasses related to structure’, Phys. Chem. Glasses, 1998, 39, (2), 83–86.
- Choi YG, Kim KH, Chernov VA, Heo J: ‘EXAFS spectroscopic study of PbO–Bi2O3–Ga2O3 glasses’, J. Non-Cryst. Solids, 1999, 259, (1–3), 205–211.
- Sugimoto N, Kanbara H, Fujiwara S, Tanaka K, Shimizugawa Y, Hirao K: ‘Third-order optical nonlinearities and their ultrafast response in Bi2O3–B2O3–SiO2 glasses’, J. Opt. Soc. Am. B, 1999, 16, (11), 1904–1908.
- Blanchandin S, Thomas P, Marchet P, Champarnaud, JC M, Frit B: ‘New heavy metal oxide glasses: investigations within the TeO2-Nb2O5-Bi2O3 system’, J. Alloys Compd, 2002, 347, 206–212.
- Ohara S, Sugimoto N, Kondo Y, Ochiai K, Kuroiwa Y, Fukasawa J, Hirose T, Hayashi H, Tanabe S: ‘Bi2O3-based glass for S-band amplification’, Proc. SPIE, 2002, 8, 4645.
- Milanova M, Iordanova R, Dimitriev Y, Klissurski D: ‘Glass formation in the MoO3–Bi2O3–PbO system’, J. Mater. Sci., 2004, 39, (9), 5591–5593.
- Hasegawa T, Nagashima T, Sugimoto N: ‘Z scan study of third-order optical nonlinearities in bismuth-based glasses’, Opt. Commun., 2005, 250, (4–6), 411–415.
- Sun H, Xu S, Dai S, Wen L, Zhang J, Hu L, Jiang Z: ‘Efficient frequency upconversion emission in Er3+-doped novel strontium lead bismuth glass’, J. Non-Cryst. Solids, 2005, 351, (3), 288–292.
- Sanz O, Haro Poniatowski E, Gonzalo J, Fernández N, J.M: ‘Influence of the melting conditions of heavy metal oxide glasses containing bismuth oxide on their optical absorption’, J. Non-Cryst. Solids, 2006, 352, (8), 761–768.
- Dai S, Lu L, Xu T, Nie Q, Shen X, Wang X: ‘Optical properties of and concentration quenching in Bi2O3–B2O3–Ga2O3 glasses’, J. Non-Cryst. Solids, 2007, 353, (28), 2744–2749.
- Hill CJ, Jha A: ‘Development of novel ternary tellurite glasses for high temperature fiber optic mid-IR chemical sensing’, J. Non-Cryst. Solids, 2007, 353, (13–15), 1372–1376.
- Shi DM, Zhang QY, Yang GF, Jiang ZH: ‘Spectroscopic properties and energy transfer in Ga2O3–Bi2O3–PbO–GeO2 glasses codoped with Tm3+ and Ho3+’, J. Non-Cryst. Solids, 2007, 353, (16–17), 1508–1514.
- Bale S, Rahman S, Awasthi AM, Sathe V: ‘Role of Bi2O3 content on physical, optical and vibrational studies in Bi2O3–ZnO–B2O3 glasses’, J. Alloys Compd, 2008, 460, (1–2), 699–703.
- Chen Y, Nie Q, Xu T, Dai S, Wang X, Shen X: ‘A study of nonlinear optical properties in Bi2O3–WO3–TeO2 glasses’, J. Non-Cryst. Solids, 2008, 354, 3468–3472.
- Sugimoto N: ‘Erbium doped fiber and highly non-linear fiber based on bismuth oxide glasses’, J. Non-Cryst. Solids, 2008, 354, (12–13), 1205–1210.
- Yang GF, Shi DM, Zhang QY, Jiang ZH: ‘Spectroscopic properties of Er3+/Yb3+-codoped PbO–Bi2O3–Ga2O3–GeO2 glasses’, J. Fluoresc., 2008, 18, (1), 131–137.
- Ozdanova J, Tichá H, Tichý L: ‘Optical band gap and Raman spectra in some (Bi2O3)x(WO3)y(TeO2)100−x−y and (PbO)x(WO3)y(TeO2)100−x−y glasses’, J. Non-Cryst. Solids, 2009, 354, (45–47), 3468–3472.
- Zhou B, Pun EY, Lin H, Yang D, Huang L: ‘Judd–Ofelt analysis, frequency upconversion, and infrared photoluminescence of Ho3+-doped and Ho3+/Yb3+-codoped lead bismuth gallate oxide glasses’, J. Appl. Phys., 2009, 106, (10), 103105.
- McCloy J, Riley B, Johnson B, Schweiger M, Qiao HA, Carlie N: ‘The predictive power of electronic polarizability for tailoring the refractivity of high-index glasses: optical basicity versus the single oscillator model’, J. Am. Ceram. Soc., 2010, 93, (6), 1650–1662.
- Brekhovskikh SM: ‘Glasses with high bismuth and lead contents’, Glass Ceram., 1957, 14, (8), 264–267.
- Singh N, Singh K: ‘Comparative study of lead borate and bismuth lead borate glass systems as gamma-radiation shielding materials’, Nucl. Instrum. Methods Phys. Res. B, 2004, 225B, (3), 305–309.
- El Batal FH: ‘Gamma ray interaction with bismuth silicate glasses’, Nucl. Instrum. Methods Phys. Res. B, 2007, 254, (2), 243–253.
- El Batal FH, Marzouk SY, Nada N, Desouky SM: ‘Gamma-ray interaction with copper doped bismuth–borate glasses’, Physica B, 2007, 391, (1), 88–97.
- Sharma G, Thind KS, Monika, Singh H, Manupriya, Gerward L: ‘Optical properties of heavy metal oxide glasses before and after γ-irradiation’, Phys. Status Solidi A, 2007, 204A, (2), 591–601.
- Kaewkhao J, Pokaipisit A, Limsuwan P: ‘Study on borate glass system containing with Bi2O3 and BaO for gamma-rays shielding materials: comparison with PbO’, J. Nucl. Mater., 2010, 399, (1), 38–40.
- Ou Y, Baccaro S, Zhang Y, Yang Y, Chen G: ‘Effect of gamma-ray irradiation on the optical properties of PbO–B2O3–SiO2 and Bi2O3–B2O3–SiO2 glasses’, J. Am. Ceram. Soc., 2010, 93, (2), 338–341.
- Johnson D, Hummel F: ‘Phase equilibria and liquid immiscibility in the system PbO–B2O3–SiO2’, J. Am. Ceram. Soc., 1968, 51, (4), 196–201.
- Shinkai N, Bradt R, Rindone G: ‘The hardness of PbO–ZnO–B2O3 glasses’, J. Mater. Sci., 1983, 18, (8), 2466–2270.
- Sarma D, Vest R: ‘Kinetics of liquid spreading and penetration with application to RuO2-glass thick-film resistors’, J. Am. Ceram. Soc., 1985, 68, 249–253.
- Nemkovich IK, Nevar OV, Formago IA, Maksimova IE: ‘Low-melting glasses for the potting of thick-film microcircuits’, Glass Ceram., 1991, 48, (12), 544–546.
- Mattox DM, Robinson JH: ‘Chemical durability of lead-oxide-based, thick-film binder glasses’, J. Am. Ceram. Soc., 1997, 80, (5), 1189–1192.
- Trubnikov I: ‘Thermal expansion and corrosion behavior of lead-borosilicate glasses’, Refract. Indust. Ceram., 2000, 41, (5–6), 169–171.
- Fujino S, Hwang C, Morinaga K: ‘Density, surface tension, and viscosity of PbO–B2O3–SiO2 glass melts’, J. Am. Ceram. Soc., 2004, 87, (1), 10–16.
- Fujino S, Hwang C, Morinaga K: ‘Surface tension of PbO–B2O3 and Bi2O3–B2O3 glass melts’, J. Mater. Sci., 2005, 40, 2207–2212.
- Roth R S, Dennis J R, McMurdie H F, (eds): ‘Figures 6255 6951’, Vol. 6; 1987, Columbus, OH, American Ceramic Society.
- Busdiecker RA: ‘Solder glass compositions and method of sealing metal therewith’, Patent US3454408, 1969.
- Hoffman LC: ‘Low melting glass and compositions containing the same’, Patent US3480566, 1969.
- Finkelstein L, Dumesnil ME, Tetschlag RR: ‘Sealing glass compositions’, Patent US5013360, 1991.
- Mandal S, Ghosh A: ‘Structure and physical properties of glassy lead vanadates’, Phys. Rev. B, 1993, 48B, (13), 9388–9394.
- Hikata H, Tanaka K, Shindo K: ‘Low temperature sealing composition’, Patent US5346863, 1994.
- Busio M, Steigelmann O: ‘New frit glasses for displays’, Glastechnische Berichte, 2000, 73, (10), 319–325.
- Jimura Y, Hayashi M: ‘CRT frit capable of sealing a CRT bulb at a relatively low temperature and in a short time’, Patent US6583079, 2003.
- Cheng Y, Xiao H, Guo W: ‘Influence of compositions on sealing temperature and properties of lead borate non-crystallizing sealing glasses’, Eng. Sci. Eng. A, 2007, A464, (1–2), 210–215.
- Tick P: ‘Water durable glasses with ultra low melting temperature’, Phys. Chem. Glasses, 1984, 25, (6), 149–154.
- Smith EF: ‘Sealing glass composite’, Patent US4801488, 1989.
- van Loan P: ‘Conductive ternary oxides of ruthenium and their use in thick film resistor glazes’, Ceram. Bull., 1972, 51, (3), 231–233; 242.
- Prudenziati M, Morten B: ‘Piezoresistive properties of thick film resistors: an overview’, Microelectron. Int., 1986, 3, (2), 20–23; 37.
- Tankiewicz S, Morten B, Prudenziati M, Golonka LJ: ‘New thick-film material for piezoresistive sensors’, Sens. Actuators A, 2001, 95A, (1), 39–45.
- Coleman M, Gurnett G: ‘The limitations of reactively-bonded thick film gold conductors’, Solid State Technol., 1979, 22, 45–51.
- Riemer DE: ‘Method for making thick film gold conductor’, Patent US5039552, 1991.
- Hara H, La Branche MH, Taylor BE: ‘Silver-rich conductor compositions for high thermal cycled and aged adhesion’, Patent US5250229, 1993.
- Pavlushkin N, Zhuravlev A, Egorova L: ‘Change in the phase compositions of lead glasses during crystallization’, Inorg. Mater., 1968, 4, 403.
- Hrovat M, Maeder T, Jacq C, Holc J, Bernard J: ‘Subsolidus phase equilibria in the PbO-poor part of the TiO2–PbO–SiO2 system and its application in low-temperature thick-film dielectrics’, J. Mater. Res., 2006, 21, (12), 3210–3214.
- Hoffman L: ‘Crystallizable dielectrics in multilayer structures for hybrid microcircuits: a review’, Ceram. Substr. Packages Electron. Appl., 1989, 26, 249–253.
- Birol H, Maeder T, Ryser P: ‘Influence of processing and conduction materials on properties of co-fired resistors in LTCC structures’, J. Eur. Ceram. Soc., 2006, 26, (10–11), 1937–1941.
- Smernos S: ‘Filler for a glass-ceramic material comprising CaF2 granules overcoated with a silicon dioxide film’, Patent US4185139, 1980.
- Bouchard RJ, Cheng LT, Roach DH, Moffett PJ: ‘Screen printable dielectric for field emission displays’, J. Am. Ceram. Soc., 2005, 88, (6), 1465–1467.
- Jacq C, Vionnet S, Maeder T: ‘Analyse FX des diélectriques commerciaux [XRF analysis of commercial dielectrics]’, EPFL, Lausanne, Switzerland, 2004.
- Jones WK, Liu Y, Larsen B, Wang P, Zampino M: ‘Chemical, structural and mechanical properties of the LTCC tapes’, Proc. Int. Symp. on ‘Microelectronics’, Boston, MA, USA, September 2000, SPIE, 669–674.
- Nishigaki S, Goebel U, Roethlingshoefer W: ‘LTCC (LFC) material systems and its application in automotive ECU’s’, Proc. 2004 IMAPS Conf. on ‘Ceramic interconnect technology’, Denver, CO, USA, June 2004, IMAPS, WP32.
- Bienert C, Roosen A: ‘Characterization of material behavior of low temperature cofired ceramics at elevated temperatures’, J. Eur. Ceram. Soc., 2010, 30, (2), 369–374.
- Anon: ‘On the restriction of the use of certain hazardous substances in electrical and electronic equipment (RoHS)’, Directive 2002/95/EC of the European Parliament and of the Council, 2002.
- Hua F, Glazer J: ‘Lead-free solders for electronic assembly’, Proc. TMS Symp. on ‘Design and reliability of solder interconnects’, Orlando, FL, USA, February 1997, TMS, 65–73.
- Anon: ‘Frequently asked questions on directive 2002/95/EC on the restriction of the use of certain hazardous substances in electrical and electronic equipment (RoHS) and directive 2002/96/EC on waste electrical and electronic equipment (WEEE)’, European Commission, Directorate-General Environment, 2006.
- Eppler R: ‘Formulation of glazes for low Pb release’, Ceram. Bull., 1975, 54, (5), 496–499.
- Graziano J, Blum C: ‘Lead exposure from lead crystal’, Lancet, 1991, 337, (8734), 141–142.
- Barbee S, Constantine L: ‘Release of lead from crystal decanters under conditions of normal use’, Food Chem. Toxic., 1994, 32, (3), 285–288.
- Tack F, Singh S, Verloo M: ‘Leaching behaviour of Cd, Cu, Pb and Zn in surface soils derived from dredged sediments’, Environ. Pollut., 1999, 106, (1), 107–114.
- Rebenklau L, Uhlemann J, Thummler M, Wolter KJ: ‘Biological characteristics of commercial low temperature cofiring ceramics’, Proc. 14th Eur. Microelectronics and Packaging Conf., Friedrichshafen, Germany, June 2003, IMAPS, 325–330.
- Uhlemann J, Schlottig G, Schindler S, Starcke S, Vollmer G, Wolter K: ‘Evaluation of cytotoxicity of chip-on-board-materials’, Proc. XXIX Int. Conf. of IMAPS Poland, Koszalin, Poland, September 2005, IMAPS, 61–68.
- Achmatowicz S, Jakubowska M, Kalenik J, Kisiel R, Mlozniak A, Zwierkowska E: ‘Lead-free silver based thick film pastes’, Proc. XXVIII Int. Conf. of IMAPS Poland Chapter, Wroclaw, Poland, September 2004, IMAPS, 151–154.
- Jeon J, Cha M, Kim H: ‘Optical properties of Bi2O3–ZnO–SiO2 glass system for eco transparent dielectric in PDP’, Mater. Sci. Forum, 2005, 486–487, 321–324.
- Morena R: ‘Phosphate glasses as alternatives to Pb-based sealing frits’, J. Non-Cryst. Solids, 2000, 263–264, 382–387.
- Coleman M: ‘Thick film materials for hybrids’, Radio Electron. Eng., 1982, 52, (5), 227–234.
- Sayers P, Petsis W: ‘Characteristics of nitrogen fireable materials for hybrids and multilayers’, Proc. Int. Symp. on ‘Microelectronics’, Minneapolis, MN, USA, September 1987, ISHM, 11–18.
- Kuo CY: ‘Nitrogen fireable tin oxide thick film resistor system’, Proc. Int. Symposium on ‘Microelectronics’, Baltimore, MD, USA, October 1989, ISHM, 150–167.
- Iseki T, Makino O, Nishida K: ‘A new copper-compatible nitrogen firing system’, Proc. Int. Symposium on ‘Microelectronics’, Baltimore, MD, USA, October 1989, ISHM, 168–174.
- Tanabe R, Chiba J: ‘Properties of new nitrogen fireable resistor system for thick film circuits’, Proc. Int. Symposium on ‘Microelectronics’, Chicago, IL, USA, October 1990, ISHM, 92–97.
- Tanabe R, Nishihara Y: ‘Resistor paste and ceramic substrate’, Patent US5264272, 1993.
- Dyshel D, Blank K: ‘Properties of resistive thick films based on powdered Sn0·9Sb0·1O2 and obtained by heat treatment in nitrogen’, Powder Metall. Met. Ceram., 1995, 34, (3–4), 219–222.
- Sutherland R, Videlo I: ‘A comparison of the reliability of copper and palladium-silver thick-film crossovers’, IEEE Trans. Compon. Hybrids Manuf. Technol., 1987, CHMT 12, (4), 676–682.
- Andriesh A, Ponomar V, Smirnov V, Mironos A: ‘Applications of chalcogenide glasses in integrated and fiber optics (review)’, Soviet J. Quant. Electron., 1986, 16, (6), 195–204.
- Lucas J: ‘Fluoride glasses’, J. Mater. Sci., 1989, 24, (1), 1–13.
- Parker J: ‘Fluoride glasses’, Ann. Rev. Mater. Sci., 1989, 19, 21–41.
- Fuxi G: ‘Structure, properties and applications of chalcohalide glasses: a review’, J. Non-Cryst. Solids, 1992, 140, 184–193.
- Prudenziati M, Zanardi F, Morten B, Gualtieri AF: ‘Lead-free thick film resistors: an explorative investigation’, J. Mater. Sci., 2002, 13, (1), 31–37.
- Fu SL, Hsi CS, Kang CY, Chin WH, Hsieh TY: ‘Influences of additives on the electrical properties of lead-free thick film resistors’, Proc. XXIX Int. Conf. of IMAPS Poland, Koszalin, Poland, September 2005, IMAPS, 19–24.
- Kiełbasiński K, Młożniak A, Jakubowska M: ‘High ohm eco-friendly resistors in thick film technology’, Proc. XXXII Int. Conf. of IMAPS Poland Chapter, Pułtusk, Poland, September 2008, IMAPS, B09.
- Kiełbasiński K, Młożniak A, Jakubowska M: ‘Vanadium oxide as a devitrification exhibitor in lead-free glass for environmental friendly thick film resistors’, Proc. XXXIII Int. Conf. of IMAPS Poland Chapter, Gliwice–Pszczyna, Poland, September 2009, IMAPS, 175–179.
- Prudenziati M, Morten B, Rane SB: ‘Perovskite ruthenate based lead free thick film resistors’, Proc. Eur. Microelectronics and Packaging Symp., Prague, Czech, June 2004 , IMAPS, 277–282.
- Rane SB, Prudenziati M, Morten B: ‘Microstructure and electrical properties of perovskite ruthenate-based lead-free thick film resistors on alumina and LTCC’, Proc. XXVIII Int. Conf. of IMAPS Poland Chapter, Wroclaw Poland, Setember 2004, IMAPS, 362–365.
- Rane SB, Prudenziati M, Morten B: ‘Environment friendly perovskite ruthenate based thick film resistors’, Mater. Lett., 2007, 61, (2), 595–599.
- Jagtap S, Rane S, Amalnerkar D: ‘Environmentally sustainable composite resistors with low temperature coefficient of resistance’, Microelectron. Eng., 2009, 86, 2026–2029.
- Hormadaly J: ‘Thick film compositions containing pyrochlore related compounds’, Patent US2004-043885, 2004.
- Ermolaeva A: ‘Glass formation and properties of glasses in the PbO–ZnO–B2O3 system’, Glass Phys. Chem., 1983, 27, (4), 306–314.
- Clinton J, Coffeen W: ‘Low melting glasses in the system B2O3–ZnO–CaO–P2O5’, Ceram. Bull., 1984, 63, (11), 1401–1404.
- Masuda H, Kimura R, Sakamoto N, Morinaga K: ‘Properties and structure of glasses in the system BaO–B2O3–ZnO’, J. Jpn Inst. Met., 1999, 63, (3), 6284–6288.
- Kim J, Choi G, Yim D, Park J, Hong K: ‘Thermal and dielectric properties of ZnO–B2O3–MO3 glasses (M = W, Mo)’, J. Electroceram., 2006, 17, 65–69.
- Hoffman L: ‘Non-toxic aluminium enamel frits’, Patent US2911312, 1959.
- Ray NH: ‘The structure and properties of inorganic polymeric phosphates’, Brit. Polym. J., 1979, 11, (4), 163–177.
- Brow RK: ‘Review: the structure of simple phosphate glasses’, J. Non-Cryst. Solids, 2000, 263–264, 1–28.
- Shih PY, Chin TS: ‘Preparation of lead-free phosphate glasses with low Tg and excellent chemical durability’, J. Mater. Sci. Lett., 2001, 20, (19), 1811–1813.
- Grebenshchikova N, Kolosenko G, Lisitsyna E: ‘Стeкло [Glass]’, Patent SU1768537, 1992.
- Jermoumi T, Hafid M, Niegisch N, Mennig M, Sabir A, Toreis N: ‘Properties of (0·5 x)ZnO–xFe2O3–0·5P2O5 glasses’, Mater. Res. Bull., 2002, 37, 49–57.
- Setina J, Akishin V, Vaivads J: ‘Glass composition for the thick film resistors’, Mater. Sci. Forum, 2005, 502, 231–236.
- Kim H, Jung B: ‘Application of lead free glass to barrier rib materials in plasma display panel’, Mater. Sci. Forum, 2003, 439, 18–22.
- Hormadaly J: ‘Lead free phosphate glasses’, Patent US2006-0128549, 2006.
- Aitken BG, Bookbinder DC, Greene ME, Morena RM: ‘Non-lead sealing glasses’, Patent US5246890, 1993.
- Bekaert E, Montagne L, Delevoye L, Palavit G, Revel B: ‘Structure and properties of x SnO–(100–x) P2O5 glasses’, Comptes Rendus de Chimie, 2004, 7, (3–4), 377–382.
- Popova E, Dimitriev Y: ‘Tin-based amorphous and composite materials’, J. Mater. Sci., 2007, 42, (10), 3358–3366.
- Shaw CM, Shelby JE: ‘The effect of stannous oxide on the properties of stannous fluorophosphate glasses’, Phys. Chem. Glasses, 1988, 29, (3), 87–90.
- Morinaga K, Fujino S: ‘Preparation and properties of SnO−SnCl2−P2O5 glass’, J. Non-Cryst. Solids, 2001, 282, (1), 118–124.
- Kikutani T: ‘Sealing material’, Patent JP2001 019472, 2001.
- Masuda H: ‘Lead-free low softening point glass’, Patent US2006/0052230, 2006.
- Mott NF: ‘Conduction in glasses containing transition metal ions’, J. Non-Cryst. Solids, 1968, 1, (1), 1–17.
- Sayer M, Mansingh A: ‘Transport properties of semiconducting phosphate glasses’, Phys. Rev. B, 1972, 6B, (12), 4629–4643.
- Murawski L, Barczynski RJ: ‘Dielectric properties of transition metal oxide glasses’, J. Non-Cryst. Solids, 1995, 185, (1–2), 84–93.
- Fang X, Ray CS, Moguš-Milanković A, Day DE: ‘Iron redox equilibrium, structure and properties of iron phosphate glasses’, J. Non-Cryst. Solids, 2001, 282, (1–3), 162–172.
- Moguš Milanković A, Šantič A, Ličina V, Day DE: ‘Dielectric behavior and impedance spectroscopy of bismuth iron phosphate glasses’, J. Non-Cryst. Solids, 2005, 351, 3235–3245.
- Rajnikant BA, Hoffman LC: ‘Air fireable compositions containing vanadium oxide and boron, and devices therefrom’, Patent US3622523, 1969.
- Dumesnil ME, Hewitt RE, Bozarth JL: ‘Low-expansion, low-melting zinc phosphovanadate glass compositions’, Patent US3650778, 1972.
- Dimitrov V, Dimitriev Y, Montenero A: ‘IR spectra and structure of V2O5–GeO2–Bi2O3 glasses’, J. Non-Cryst. Solids, 1994, 180, (1), 51–57.
- Iordanova R, Dimitrov V, Dimitriev Y, Klissurski D: ‘Glass formation and structure of glasses in the V2O5–MoO3–Bi2O3 system’, J. Non-Cryst. Solids, 1994, 180, (1), 58–65.
- Iordanova R, Dimitriev Y, Dimitrov V, Kassabov S, Klissurski D: ‘Glass formation and structure in the V2O5–Bi2O3–Fe2O3 glasses’, J. Non-Cryst. Solids, 1996, 204, (2), 141–150.
- Iordanova R, Dimitriev Y, Dimitrov V, Kassabov S, Klissurski D: ‘Glass formation and structure in the system MoO3–Bi2O3–Fe2O3’, J. Non-Cryst. Solids, 1998, 231, 227–233.
- Iordanova R, Lefterova E, Uzunov I, Dimitriev Y, Klissurski D: ‘Non isothermal crystallization kinetics of V2O5−MoO3−Bi2O3 glasses’, J. Therm. Anal. Calorim., 2002, 70, (2), 393–404.
- Aitken BG, Dejneka MJ: ‘Tungstate, molybdate, vanadate base glasses’, Patent US6376399, 2002.
- El-Mallawany RAH: ‘Tellurite glasses handbook – physical properties and data’; 2002, Boca Raton, FL, CRC Press.
- Basu A, Lewis JM, O’Biren P, Feller H, Starns SR, Fruch J, Feller SA, Affatigato M: ‘Glass formation, molar volumes and glass transition temperatures of binary vanadate glasses’, Phys. Chem. Glasses, 2003, 44, (1), 1–4.
- Rajendran V, Palanivelu N, Chaudhuri B, Goswami K: ‘Characterisation of semiconducting V2O5–Bi2O3–TeO2 glasses through ultrasonic measurements’, J. Non-Cryst. Solids, 2003, 32, 195–209.
- Udovic M, Thomas P, Mirgorodsky A, Durand O, Soulis M, Masson O, Merle Méjean T, Champarnaud Mesjard JC: ‘Thermal characteristics, Raman spectra and structural properties of new tellurite glasses within the Bi2O3–TiO2–TeO2 system’, J. Solid State Chem., 2006, 179, (10), 3252–3259.
- Yousef E, Hotzel M, Rüssel C: ‘Effect of ZnO and Bi2O3 addition on linear and non linear optical properties of tellurite glasses’, J. Non-Cryst. Solids, 2007, 353, (4), 333–338.
- Snowdon K: ‘Sealing glass composition’, Patent EP1391437, 2004.
- Fukaya M, Matsuo T, Nishigaki S, Higuchi C: ‘Highly reliable and lead (Pb) free thick film resistor paste system for low thermal expansion LTCC application’, Proc. 1997 Int. Symp. on ‘Microelectronics’, Philadelphia, PA, USA, October 1997, IMAPS, 65–71.
- Heynes MSR, Rawson H: ‘Bismuth trioxide glasses’, J. So.iety of Glass Technol., 1957, 41, 347–349.
- Imaoka M: ‘Glass-formation and glass structure’, Proc. Int. Cong. Glass Brussels, 1965, 7, 149–164.
- Janakirama-Rao BV: ‘Unusual properties and structure of glasses in the systems Bi2O3–B2O3–SrO; Bi2O3–B2O3–BaO; Bi2O3–B2O3–ZnO and Bi2O3–B2O3–PbO’, Proc. 7th Int. Cong. on ‘Glass’, Brussels, Belgium, June 1965, 104.101–104.106.
- Bishay AM, Maghrabi C: ‘Properties of bismuth glasses in relation to structure’, Phys. Chem. Glasses, 1969, 10, (10), 1–11.
- George HB, Vira C, Stehle C, Meyer J, Evers S, Hogan D, Feller S, Affatigato M: ‘A structural analysis of the physical properties of bismuth and lead based glasses’, Phys. Chem. Glasses, 1999, 40, (6), 326–332.
- Shuster NS, Novruzova FA, Zeinalova CLK, Zargarova MI: ‘Glass forming and physico-chemical properties of glasses in the system CuO−Bi2O3−B2O3’, Fizika i Chimija Stekla, 1990, 16, (2), 197–200.
- Hormadaly J: ‘Cadmium-free and lead-free thick film conductor composition’, Patent US5439852, 1995.
- Hormadaly J: ‘Cadmium-free and lead-free thick film paste composition’, Patent US5491118, 1996.
- Qiu HH, Ito T, Sakata H: ‘DC conductivity of Fe2O3–Bi2O3–B2O3 glasses’, Mater. Chem. Phys., 1999, 58, 243–248.
- Watanabe T, Muratsubaki K, Benino Y, Saitoh H, Komatsu T: ‘Hardness and elastic properties of Bi2O3 based glasses’, J. Mater. Sci., 2001, 36, (10), 2427–2433.
- El Batal F, Azooz M, Ezz-Eldin F: ‘Thermal expansion and infrared studies of binary Bi2O3−B2O3 and ternary Bi2O3−B2O3−PbO glasses’, Phys. Chem. Glasses, 2002, 43, (5), 260–266.
- Baia L, Stefan R, Popp J, Simon S, Kiefer W: ‘Vibrational spectroscopy of highly iron doped B2O3–Bi2O3 glass systems’, J. Non-Cryst. Solids, 2003, 324, (1–2), 109–117.
- Rachkovskaya G, Zakharevich G: ‘Properties, structure, and application of low-melting lead–bismuth glasses’, Glass Ceram., 2004, 61, (1–2), 9–12.
- Dyamant I, Itzhak D, Hormadaly J: ‘Thermal properties and glass formation in the SiO2–B2O3–Bi2O3–ZnO quaternary system’, J. Non-Cryst. Solids, 2005, 351, 3503–3507.
- Sindhu S, Sanghi S, Agarwal A, Seth VP, Kishore N: ‘Effect of Bi2O3 content on the optical band gap, density and electrical conductivity of MO·Bi2O3·B2O3 (M = Ba, Sr) glasses’, Mater. Chem. Phys., 2005, 90, (1), 83–89.
- Hamezan M, Sidek H, Zaidan A, Kaida K, Zainal A: ‘Elastic constants and thermal properties of lead bismuth borate glasses’, J. Appl. Sci., 2006, 6, (4), 943–949.
- Kim YJ, Hwang SJ, Kim HS: ‘Thermal properties of Bi2O3–B2O3–ZnO glass system’, Mater. Sci. Forum, 2006, 510–511, 578–581.
- Aziz S, Hamezan A, Zaidan A, Zainal A, Zainal A, Shaari A, Senin H: ‘Ultrasonic and thermal properties of borate and phosphate glasses containing bismuth and lead’, AIP Conf. Proc., 2007, 909, 197–209.
- Jirák J, Koudelka L, Pospíšil J, Mošner P, Montagne L, Delevoye L: ‘Study of structure and properties of ZnO–Bi2O3–P2O5 glasses’, J. Mater. Sci., 2007, 42, (20), 8592–8598.
- Kim BS, Lim ES, Lee JH, Kim JJ: ‘Effect of Bi2O3 content on sintering and crystallization behavior of low-temperature firing Bi2O3–B2O3–SiO2 glasses’, J. Eur. Ceram. Soc., 2007, 27, (2–3), 819–824.
- Saritha D, Markandeya Y, Salagram M, Vithal M, Singh AK, Bhikshamaiah G: ‘Effect of Bi2O3 on physical, optical and structural studies of ZnO–Bi2O3–B2O3 glasses’, J. Non-Cryst. Solids, 2008, 354, (52–54), 5573–5579.
- Na YH, Kim NJ, Im SH, Cha JM, Ryu BK: ‘Effect of Bi2O3 on structure and properties of zinc bismuth phosphate glass’, J. Ceram. Soc. Jpn, 2009, 117, (1371), 1273–1276.
- Doweidar H, Saddeek YB: ‘FTIR and ultrasonic investigations on modified bismuth borate glasses’, J. Non-Cryst. Solids, 2009, 355, 348–354.
- Im S, Na Y, Kim N, Kim D, Hwang C, Ryu B: ‘Structure and properties of zinc bismuth phosphate glass’, Thin Solid Films, 2010, 518, (24S1), e46–e49.
- Koo HY, Yi JH, Kang YC: ‘Characteristics of Bi based glass powders with various glass transition temperatures prepared by spray pyrolysis’, Ceram. Int., 2010, 36, (5), 1749–1753.
- Majhi K, Varma KBR: ‘Dielectric relaxation in CaO–Bi2O3–B2O3 Glasses’, Phys. Chem. Glasses, 2010, 7, (S1), E89–E97.
- Oganesyan MR, Oganesyan RM, Knyazyan NB: ‘Interrelation between glass formation, liquidus temperature, and the cooling rate of barium-bismuth-borate melts’, Theor. Found. Chem. Eng., 2010, 44, (4), 500–502.
- Qiao W, Chen P: ‘Study on the properties of Bi2O3–B2O3–BaO lead free glass using in the electronic pastes’, Glass Phys. Chem., 2010, 36, (3), 1956–1960.
- Shapaan M, Ebrahima F: ‘Structural and electric–dielectric properties of B2O3–Bi2O3–Fe2O3 oxide glasses’, Physica B, 2010, 405, (16), 3217–3222.
- Honma T, Benino Y, Fujiwara T, Komatsu T, Sato R: ‘Crystalline phases and YAG laser induced crystallization in Sm2O3–Bi2O3–B2O3 glasses’, J. Am. Ceram. Soc., 2005, 88, (4), 989–992.
- Simon S, Eniu D: ‘Spectroscopic characterisation of local structure in Y2O3–B2O3–Bi2O3 glasses doped with gadolinium’, J. Mater. Sci., 2007, 42, (15), 5949–5953.
- Cheng Y, Xiao H, Guo W: ‘Influences of La3+ and Er3+ on structure and properties of Bi2O3–B2O3 glass’, Ceram. Int., 2008, 34, (5), 1335–1339.
- Pascuta P, Borodi G, Culea E: ‘Influence of europium ions on structure and crystallization properties of bismuth borate glasses and glass ceramics’, J. Non-Cryst. Solids, 2008, 354, (52–54), 5475–5479.
- Pascuta P, Rada S, Borodi G, Bosca M, Pop L, Culea E: ‘Influence of europium ions on structure and crystallization properties of bismuth-alumino-borate glasses and glass ceramics’, J. Molec. Struct., 2009, 924–926, 214–220.
- Rada S, Culea M, Rada M, Pascuta P, Matles V, Culea E: ‘The double role played by the Gd2O3 in the gadolinium–aluminum–borate–bismuthate quaternary glass forming tendency. GdBO3 crystalline phase’, J. Molec. Struct., 2009, 937, 70–74.
- Zargarova MI, Shuster NS: ‘Projection of the liquidus surface for the system PbO−Bi2O3−B2O3 system’, Inorg. Mater., 1993, 29, (4), 540–544.
- Zhereb VP, Skorikov VM: ‘Metastable states in bismuth containing oxide systems’, Inorg. Mater., 2003, 39, (S2), S121–S145.
- Fei YT, Fan SJ, Sun RY, Xu JY, Ishii M: ‘Crystallizing behavior of Bi2O3–SiO2 system’, J. Mater. Sci. Lett., 2000, 19, (11), 893–895.
- Kargin YF, Zhereb VP, Egorysheva AV: ‘Metastable phase diagram for the Bi2O3–B2O3 system’, Russ. J. Inorg. Chem., 2002, 47, (8), 1240–1242.
- Knox JJ: ‘Vitrifiable flux and bonding composition containing same’, Patent US2385580, 1945.
- Short OA: ‘Vitrifiable flux and silver compositions containing same’, Patent US2819170, 1958.
- Dumesnil ME: ‘Vitrifiable inorganic ceramic binder and silver compositions containing the same’, Patent US2942992, 1960.
- Martin JH: ‘Ceramic with metal film via binder of copper oxide containing glass’, Patent 1966-12, 1966.
- Hoffman L: ‘Metallizing compositions containing bismuthate glass ceramic conductor binder’, Patent US3741780, 1973.
- Taylor BE: ‘Binder glass of Bi2O3–SiO2–GeO2–(PbO optional) admixed with ZnO/ZnO and Bi2O3’, Patent US4567151, 1986.
- Ermolenko NN, Manchenko ZF, Samujlova VN, Shamkalovich V: ‘Low-melting glass’, Patent SU775061, 1980.
- Ermolenko NN, Manchenko ZF, Saevich NG, Samujlova VN, Grigorev VM, Ivolgin VM, Titova RA: ‘Low-melting glass’, Patent SU923'976, 1982.
- Ermolenko NN, Diatlova EM, Martinova LN: ‘Low-melting glass’, Patent SU947102, 1982.
- Ermolenko NN, Manchenko ZF, Karpovich EF, Tikhonov IA, Saevich NG, Panov LI, Sokolovskii BR: ‘Glass’, Patent SU1169951, 1985.
- Tikhonov I, Ermolenko N, Manchenko Z, Saevich N, Savelov I: ‘Low-melting glass’, Patent SU1’477’706, 1989.
- Matveev GM, Zainullin GG: ‘New glass systems and the development of glasses based on them’, Glass Ceram., 1980, 37, (4), 211–213.
- Francel J: ‘Chemical resistant lead-free glass frit composition’, Patent US4554258, 1985.
- Reinherz BP: ‘Lead-free glass frit compositions’, Patent US4892847, USA, 1990.
- Wada M, Toru H: ‘Glass compound for overcoat or sealing of transparent electrode’, Patent JP05-165042, 1993.
- Prunchak R: ‘High bismuth oxide based flux and paint compositions for glass substrates’, Patent US5629247, 1997.
- Sakoske GE: ‘Niedrig schmelzende, bleifreie Glas- und Emailzusammensetzungen mit hohem Bismutgehalt [low-melting, lead-free glass and glaze compositions with high bismuth content]’, Patent EP0955274, 2000.
- Sakoske GE: ‘Bleifreie, alkalimetallfreie Glaszusammensetzungen [Lead-free and alkali-metal-free glass compositions]’, Patent EP1006088, 2000.
- Sridharan S, Blonski RP, Emlemdi H, Roberts GJ, Joyce IH: ‘Glass enamel for automotive applications’, Patent WO00-41977, 2000.
- Sridharan S, Moroz M: ‘Electronic device having lead and cadmium free electronic overglaze applied thereto’, Patent US7740899, 2010.
- Carroll AF, Hang KW: ‘Lead-free thick film paste composition’, Patent US5378408, 1995.
- Carroll AF, Hang KW: ‘Lead-free thick film paste composition’, Patent US5468695, 1995.
- Fujii T, Neyagawa T: ‘Electrode material, dielectric material and plasma display panel using them’, Patent US6787239, 2004.
- Hormadaly J, Taylor BE: ‘Encapsulant composition’, Patent US5137851, 1992.
- Donohue PC: ‘Lead and cadmium-free encapsulant composition’, Patent EP0858973, 1999.
- Hayakawa N, Shimooka T: ‘Lead-free low-melting glass’, Patent EP1361199, 2003.
- Sanada T: ‘Electroconductive composition for glass substrate and anti fog window glass for automobiles’, Patent USA6787239, 1999.
- Miki T, Maeda A: ‘Conductive paste and ceramic electronic component’, Patent US6500362, 2003.
- Usui H, Manabe T, Harada K, Tanabe R: ‘Hermetic sealing composition’, Patent US5733828, 1998.
- Nachmansohn B: ‘Verre de soudure à dévitrification sans plomb ni autres matériaux toxiques [Devitrifying solder glass without lead or other toxic materials]’, Patent FR2794120, 2000.
- Hasegawa S, Kanai M, Torii H, Kamimoto T: ‘Glass composition, sealing glass for magnetic head and magnetic head’, Patent European Patent 1’291’329, 2003.
- Hasegawa S, Onishi H, Kanai M, Kamimoto T: ‘Bismuth glass composition, and magnetic head and plasma display panel including the same as sealing member’, Patent US2005 0181927, 2005.
- Nemilov SV, Burunova ON, Gilev IS: ‘Viscosity and elasticity of glasses in the system Bi2O3–B2O3’, J. Appl. Chem. USSR, 1972, 45, (6), 1234–1239.
- Dimesso L, Gnappi G, Montenero A, Fiorani D, Pye LD: ‘Crystallization of Bi–Zn–Fe–B–O glasses’, J. Non-Cryst. Solids, 1989, 112, (1–3), 191–197.
- Becker P: ‘Thermal and optical properties of glasses of the system Bi2O3–B2O3’, Cryst. Res. Technol., 2003, 38, (1), 74–82.
- Bajaj A, Khanna A, Chen B, Longstaffe JG, Zwanziger UW, Zwanziger JW, Gómez Y, González F: ‘Structural investigation of bismuth borate glasses and crystalline phases’, J. Non-Cryst. Solids, 2009, 355, (1), 45–54.
- Kusz B, Trzebiatowski K, Barczynski RJ: ‘Ionic conductivity of bismuth silicate and bismuth germanate glasses’, Solid State Ion., 2003, 159, (3–4), 293–299.
- Hwang SJ, Lee SW, Kim HS: ‘Sintering behavior of silver conductive thick film with frit in information display’, J. Electroceram., 2008, 23, (2–4), 351–355.
- Hormadaly J: ‘Cadmium-free and lead-free glass compositions, thick film formulations containing them and uses thereof’, Patent US6171987, 2001.
- Busana MG, Prudenziati M, Hormadaly J: ‘Microstructure development and electrical properties of RuO2 based lead free thick film resistors’, J. Mater. Sci., 2006, 17, (11), 951–962.
- Jacq C, Maeder T, Ryser P: ‘Development of low-firing lead-free thick-film materials on steel alloys for piezoresistive sensor applications’, Proc. 17th IMAPS Eur. Microelectronics and Packaging Conf., Rimini, Italy, June 2009, IMAPS, P07.
- Hirayama C, Subbarao EC: ‘Dielectric properties of bismuth borate glasses’, Phys. Chem. Glasses, 1962, 3, (4), 111–115.
- Saisudha MB, Koteswara Rao KSR, Bhat HL, Ramakrishna J: ‘The fluorescence of Nd3+ in lead borate and bismuth borate glasses with large stimulated emission cross section’, J. Appl. Phys., 1996, 80, (9), 4845–4853.
- Cheng Y, Xiao H, Guo W, Guo W: ‘Structure and crystallization kinetics of Bi2O3–B2O3 glasses’, Thermochim. Acta, 2006, 444, (2), 173–178.
- Dimitrov V, Komatsu T: ‘Average single bond strength and optical basicity of Bi2O3–B2O3 and Sb2O3–B2O3 glasses’, J. Non-Cryst. Solids, 2010, 356, (4–5), 258–262.
- Yawale SP, Pakade SV: ‘D.C. conductivity and hopping mechanism in Bi2O3-B2O3 glasses’, J. Mater. Sci., 1993, 28, (20), 5451–5455.
- Dimitrov V, Komatsu T: ‘Electronic polarizability, optical basicity and non-linear optical properties of oxide glasses’, J. Non-Cryst. Solids, 1999, 249, (2–3), 160–179.
- Oprea I, Hesse H, Betzler K: ‘Optical properties of bismuth borate glasses’, Opt. Mater., 2004, 26, 235–237.
- Oprea I: ‘Optical properties of borate glass-ceramics’, PhD thesis, Universität Osnabrück, Osnabrück, Germany, 2005.
- Watanabe Y, Sakata SI, Watanabe T, Tsuchiya T: ‘Two-photon absorption in binary Bi2O3–B2O3 glass at 532 nm’, J. Non-Cryst. Solids, 1998, 240, (1–3), 212–220.
- Kirdsiri K, Kaewkhao J, Chewpraditkul W, Limsuwan P: ‘Photon interaction in borate glass system containing Bi2O3, PbO and BaO at 60 keV: theoretical calculation’, Adv. Mater. Res., 2010, 93–94, 9–12.
- El Sayed M, Saddeek YB, Shaaban ER: ‘Structural and optical properties of lithium borobismuthate glasses’, J. Phys. Chem. Solids, 2008, 69, (9), 2281–2287.
- Saddeek YB, Shaaban ER, El Sayed M, Hesham MM: ‘Spectroscopic properties, electronic polarizability, and optical basicity of Bi2O3–Li2O–B2O3 glasses’, Physica B, 2008, 403, (13–16), 2399–2407.
- Subhadra M, Kistaiah P: ‘Characterization and optical absorption studies of VO2+:Li2O–K2O–Bi2O3–B2O3 glass system’, J. Alloys Compd, 2010, 505, 634–639.
- Bale S, Rahman S: ‘Glass structure and transport properties of Li2O containing zinc bismuthate glasses’, Opt. Mater., 2008, 31, (2), 333–337.
- Rani S, Sanghi S, Anshu A, Agarwal A, Kishore N, Seth VP: ‘Effect of ZnO/CdO on the structure and electrical conductivity in Li2O·MO·Bi2O3·B2O3 glasses (M = Zn, Cd)’, J. Phys. Chem. Solids, 2008, 69, (7), 1855–1860.
- Al Hajry A, Soliman AA, El Desoky MM: ‘Electrical and thermal properties of semiconducting Fe2O3–Bi2O3–Na2B4O7 glasses’, Thermochim. Acta, 2005, 427, (1–2), 181–186.
- Saddeek YB, Aly KA, Dahshan A, El Kashef IM: ‘Optical properties of the Na2O–B2O3–Bi2O3–MoO3 glasses’, J. Alloys Compd, 2010, 494, (1–2), 210–213.
- El Desoky MM, Tashtoush NM, Habib H: ‘Characterization and electrical properties of semiconducting Fe2O3–Bi2O3–K2B4O7 glasses’, J. Mater. Sci., 2005, 16, (8), 533–539.
- El Desoky MM, Al Shahrani A: ‘Variable-range hopping in Fe2O3–Bi2O3–K2B4O7 glasses’, J. Mater. Sci., 2005, 16, (4), 221–224.
- El Desoky MM, Al Shahrani A: ‘Iron doping of semiconducting bismuth alkali borate glasses’, Physica B, 2006, 383B, (2), 163–170.
- Kargin YF, Ivicheva SN, Shvorneva LI: ‘Phase relations in the MgO–Bi2O3–Bi2O3 system’, Russ. J. Inorg. Chem, 2008, 53, (8), 1297–1299.
- Kargin YF, Ivicheva SN, Shvorneva LI, Komova MG, Krut’ko VA: ‘Phase relations in the CaO–Bi2O3–B2O3 system in the subsolidus region’, Russ. J. Inorg. Chem, 2008, 53, (9), 1512–1516.
- Kargin YF, Ivicheva SN, Komova MG, Krut’ko VA: ‘Phase relations in the solidus region of the SrO–Bi2O3–B2O3 system’, Russ. J. Inorg. Chem, 2008, 53, (3), 474–478.
- Egorysheva AV, Skorikov VM, Volodin VD, Myslitskii OE, Kargin YF: ‘Phase equilibria in the BaO–Bi2O3–B2O3 system’, Russ. J. Inorg. Chem, 2006, 51, (12), 1956–1960.
- Egorysheva AV, Kargin YF: ‘Phase equilibria in the Bi2O3–BaB2O4–B2O3 system in the subsolidus region’, Russ. J. Inorg. Chem, 2006, 51, (7), 1106–1110.
- Egorysheva AV, Volodin VD, Skorikov VM: ‘Glass formation in the Bi2O3–B2O3–BaO system’, Inorg. Mater., 2008, 44, (11), 1261–1265.
- Tichý L, Tichá H, Herzigová L, Ozdanova J: ‘Some physical properties of (Bi2O3)x(BaO)0·5−x(B2O3)0·5 glasses’, Mater. Chem. Phys., 2011, 126, (1–2), 289–294.
- Barbier J, Penin N, Denoyer A, Cranswick LMD: ‘BaBiBO4, a novel non-centrosymmetric borate oxide’, Solid State Sci., 2005, 7, (9), 1055–1061.
- Kotková K, Tichá H, Tichý L: ‘Raman studies and optical properties of some (PbO)x(Bi2O3)0·2(B2O3)0·8−x glasses’, J. Raman Spectrosc., 2008, 39, (9), 1219–1226.
- Peng M, Cordt Z, Wondraczek L: ‘Origin of broad NIR photoluminescence in bismuthate glass and Bi-doped glasses at room temperature’, J. Phys.: Condensed Matter, 2009, 21, (28), 285106.
- Zargarova MI, Kasumova MF: ‘Projection of the liquidus surface of the ZnO−Bi2O3−B2O3 system’, Inorg. Mater., 1990, 26, (8), 1427–1430.
- Kim BS, Lim ES, Lee JH, Kim JJ: ‘Effect of structure change on thermal and dielectric characteristics in low temperature firing Bi2O3–B2O3–ZnO glasses’, J. Mater. Sci., 2007, 42, (12), 4260–4264.
- Zargarova MI, Mustafaev NM, Shuster NS: ‘CuO–Bi2O3–B2O3 system’, Inorg. Mater., 1996, 32, (1), 65–70.
- Pascuta P, Pop L, Rada S, Bosca M, Culea E: ‘The local structure of bismuth borate glasses doped with europium ions evidenced by FT-IR spectroscopy’, J. Mater. Sci., 2008, 19, (5), 424–428.
- Saddeek YB, Yahia IS, Aly KA, Dobrowolsky W: ‘Spectroscopic, mechanical and magnetic characterization of some bismuth borate glasses containing gadolinium ions’, Solid State Sci., 2010, 12, (8), 1426–1434.
- Fan H, Wang G, Hu L: ‘Infrared, Raman and XPS spectroscopic studies of Bi2O3–B2O3–Ga2O3 glasses’, Solid State Sci., 2009, 11, (12), 2065–2070.
- Fan H, Hu L, Yang K, Fang Y: ‘Structure and physical properties of Bi2O3–B2O3–Ga2O3 glasses’, J. Non-Cryst. Solids, 2010, 356, (35–36), 1814–1818.
- Zhang Y, Yang Y, Zheng J, Hua W, Chen G: ‘Effects of oxidizing additives on optical properties of Bi2O3–B2O3–SiO2 glasses’, J. Am. Ceram. Soc., 2008, 91, (10), 3410–3412.
- Gao G, Hu L, Fan H, Wang G, Li K, Feng S, Fan S, Chen H: ‘Effect of Bi2O3 on physical, optical and structural properties of boron silicon bismuthate glasses’, Opt. Mater., 2009, 32, (1), 159–163.
- Gerth K, Rüssel C: ‘Crystallization of Bi4Ti3O12 from glasses in the system Bi2O3/TiO2/B2O3’, J. Non-Cryst. Solids, 1997, 221, (1), 10–17.
- Gerth K, Rüssel C: ‘Crystallization of Bi3TiNbO9 from glasses in the system Bi2O3/TiO2/Nb2O5/B2O3/SiO2’, J. Non-Cryst. Solids, 1999, 243, (1), 52–60.
- Fei YT, Fan SJ, Sun RY, Ishii M: ‘Study on phase diagram of Bi2O3−SiO2 system for Bridgman growth of Bi4Si3O12 single crystal’, Prog. Cryst. Growth Charact. Mater., 2000, 40, (1–4), 183–188.
- Gackowska J, Gazda M, Trzebiatowski K, Kusz B: ‘Structure and electric conductivity of reduced lead–germanate, bismuth–germanate and bismuth–silicate glasses modified with potassium’, J. Non-Cryst. Solids, 2008, 354, (35–39), 4319–4322.
- Abo Naf SM, Elwan RL, Marzouk M: ‘Structure–property correlations in the SiO2–PbO–Bi2O3 glasses’, J. Mater. Sci., 2012, 23, (5), 1022–1030.
- Dimesso L, Gnappi G, Montenero A, Fabeni P, Pazzi GP: ‘The crystallization behaviour of bismuth germanate glasses’, J. Mater. Sci., 1991, 26, (15), 4215–4219.
- Nassau K, Chadwick DL: ‘Glass-forming systems involving GeO2 with Bi2O3, Tl2O, and PbO’, J. Am. Ceram. Soc., 1982, 65, (10), 486–491.
- Shi DM, Zhao YG, Qian Q, Chen DD, Zhang QY: ‘Role of PbO substitution by Bi2O3 on 1·47 μm luminescence properties of Tm3+/Tb3+-doped Bi2O3–GeO2–Ga2O3 glass’, J. Alloys Compd, 2010, 499, (1), 126–130.
- Zhang QY, Li T, Jiang ZH, Ji XH, Buddhudu S: ‘980 nm laser-diode-excited intense blue upconversion in Tm3+/Yb3+-codoped gallate–bismuth–lead glasses’, Appl. Phys. Lett., 2005, 87, (17), 171911.
- Sen A, Chakravorty D, Kumar J: ‘Infrared transmitting bismuth germanate glasses containing zinc oxide’, J. Non-Cryst. Solids, 1986, 88, (2–3), 253–261.
- Ardelean I, Peteanu M, Simon V, Bob C, Filip S: ‘EPR and magnetic susceptibility studies of Cr2O3–Bi2O3–GeO2 glasses’, J. Mater. Sci., 1998, 33, (2), 357–362.
- Pascuta P, Pop L, Rada S, Bosca M, Culea E: ‘The local structure of bismuth germanate glasses and glass ceramics doped with europium ions evidenced by FT IR spectroscopy’, Vibrat. Spectrosc., 2008, 48, (2), 281–284.
- Feltz A, Morr A: ‘Redox reactions in condensed oxide systems. III. Glass formation and properties in the Bi2O3−P2O5 system’, J. Non-Cryst. Solids, 1985, 74, (2–3), 313–324.
- El Adawy AA: ‘Effect of annealing temperature on the elastic properties of bismuth phosphate glasses’, J. Mater. Sci. Letters, 1996, 15, (23), 2061–2064.
- Voevodskii VY, Kargin YF, Skorikov VM: ‘System Bi2O3–P2O5 in composition range 50–85 mol% P2O5’, Russ. J. Inorg. Chem, 1997, 42, (5), 715–717.
- Rani S, Sanghi S, Agarwal A, Ahlawat N: ‘Influence of Bi2O3 on optical properties and structure of bismuth lithium phosphate glasses’, J. Alloys Compd, 2009, 477, 504–509.
- Pöppl L, Szaller Z: ‘Reactions and phases within the TeO2-rich part of the Bi2O3–TeO2 system − the non-equilibrium phase diagram’, J. Therm. Anal. Calorim., 2003, 74, (2), 375–386.
- Udovic M, Valant M, Jančar B, Suvorov D: ‘Phase formation and crystal structure determination in the Bi2O3−TiO2−TeO2 system prepared in an oxygen atmosphere’, J. Am. Ceram. Soc., 2006, 89, (11), 3462–3469.
- Fu J: ‘Novel bismuthate glasses’, J. Non-Cryst. Solids, 1996, 194, (1–2), 207–209.
- Pan A, Ghosh A: ‘A new family of lead-bismuthate glass with a large transmitting window’, J. Non-Cryst. Solids, 2000, 271, (1–2), 157–161.
- Wong Ng W, Cook LP, Jiang F: ‘Subsolidus and melting study of the Bi2O3–PbOx−CuO system in air’, Appl. Superconduct., 1996, 4, (9), 385–397.
- Radu A, Baia L, Kiefer W, Simon S: ‘The influence of manganese cations on the structure of lead high bismuthate glasses and glass ceramics’, Vibrat. Spectrosc., 2005, 39, (2), 127–130.
- Miyaji F, Sakka S: ‘Structure of PbO–Bi2O3–Ga2O3 glasses’, J. Non-Cryst. Solids, 1991, 134, (1–2), 77–85.
- Kharlamov AA, Almeida RM, Heo J: ‘Vibrational spectra and structure of heavy metal oxide glasses’, J. Non-Cryst. Solids, 1996, 202, (3), 233–240.
- dos Santos IMG, Martins Moreira RC, de Souza AG, Lebullenger R, Hernandes AC, Leite ER, Paskocimas CA, Longo E: ‘Ceramic crucibles: a new alternative for melting of PbO–BiO1·5–GaO1·5 glasses’, J. Non-Cryst. Solids, 2003, 319, 304–310.
- Watanabe T, Nanba T, Miura Y: ‘X-ray and neutron scattering study of the structure of lithium bismuth oxide glass’, J. Non-Cryst. Solids, 2002, 297, (1), 73–83.
- Nassau K, Grasso M, Glass AM: ‘Quenched glasses in the systems of Li2O with Al2O3, Ga2O3 and Bi2O3’, J. Non-Cryst. Solids, 1979, 34, (3), 425–436.
- Hazra S, Ghosh A: ‘Structure and properties of nonconventional glasses in the binary bismuth cuprate system’, Phys. Rev. B, 1995, 51B, (2), 851–856.
- Gosh AW: ‘Transport properties of iron – bismuthate glassy semiconductors’, J. Appl. Phys., 1989, 66, (6), 2425.
- Nitta A, Koide M, Matusita K: ‘Glass formation and thermal properties of Bi2O3–ZnO–B2O3–R2O quaternary systems’, Phys. Chem. Glasses, 2001, 42, (4–5), 275–278.
- Baia L, Ilescu T, Simon S, Kiefer W: ‘Raman and IR spectroscopic studies of manganese doped GeO2–Bi2O3 glasses’, J. Molec. Struct., 2001, 599, (1–3), 9–13.
- Baia L, Stefan R, Kiefer W, Popp J, Simon S: ‘Structural investigations of copper doped B2O3–Bi2O3 glasses with high bismuth oxide content’, J. Non-Cryst. Solids, 2002, 303, (3), 379–386.
- Nitta A, Miura T, Komatsu T, Matusita K: ‘Glass formation in the system SiO2–PbO containing transition metal oxides’, J. Am. Ceram. Soc., 1989, 72, (1), 163–165.
- Boonin K, Kaewkhao J, Limsuwan P: ‘Optical investigation of Bi2O3−B2O3 glass system’, Adv. Mater. Res., 2010, 93–94, 336–339.
- Takaishi T, Jin J, Uchino T, Yoko T: ‘Structural study of PbO–B2O3 glasses by X-ray diffraction and 11B MAS NMR techniques’, J. Am. Ceram. Soc., 2000, 83, (10), 2543–2548.
- Hayashi A, Nakai M, Tatsumisago M, Minami T, Himei Y, Miura Y, Katada M: ‘Structural investigation of SnO–B2O3 glasses by solid-state NMR and X-ray photoelectron spectroscopy’, J. Non-Cryst. Solids, 2002, 306, (3), 227–237.
- Duffy JA, Ingram MD: ‘An interpretation of glass chemistry in terms of the optical basicity concept’, J. Non-Cryst. Solids, 1976, 21, (3), 373–410.
- Dimitrov V, Komatsu T: ‘Classification of simple oxides: a polarizability approach’, J. Solid State Chem., 2002, 163, (1), 100–112.
- Duffy J: ‘Relationship between cationic charge, coordination number, and polarizability in oxidic materials’, J. Phys. Chem. B, 2004, 108B, (37), 14137–14141.
- Meera BN, Ramakrishna J: ‘Raman spectral studies of borate glasses’, J. Non-Cryst. Solids, 1993, 159, (1–2), 1–21.
- Walsh A: ‘The origin of structural distortions in metal ceramics’, PhD thesis, University of Dublin, Dublin, Germany, 2006.
- Yiannopoulos YD, Chryssikos GD, Kamitsos EI: ‘Structure and properties of alkaline earth borate glasses’, Phys. Chem. Glasses, 2001, 42, (3), 164–172.
- Zhong J, Bray PJ: ‘Change in boron coordination in alkali borate glasses, and mixed alkali effects, as elucidated by NMR’, J. Non-Cryst. Solids, 1989, 111, (1), 67–76.
- Bale S, Purnima M, Srinivasu C, Rahman S: ‘Vibrational spectra and structure of bismuth based quaternary glasses’, J. Alloys Compd, 2008, 457, (1–2), 545–548.
- Yoko T, Tadanaga K, Miyaji F, Sakka S: ‘A 207Pb MAS-NMR study of Pb containing glasses’, J. Non-Cryst. Solids, 1992, 150, (1–3), 192–196.
- Witkowska A, Rybicki J, di Cicco A: ‘Structure of partially reduced bismuth–silicate glasses: EXAFS and MD study’, J. Alloys Compd, 2005, 401, 135–144.
- Witkowska A, Sikora B, Trzebiatowski K, Rybicki J: ‘Germanate anomaly in heavy metal oxide glasses: an EXAFS analysis’, J. Non-Cryst. Solids, 2006, 352, (40–41), 4356–4361.
- Umesaki N, Brunier TM, Wright AC, Hannon AC, Sinclair RN: ‘Neutron scattering from PbO–GeO2 glasses’, J. Non-Cryst. Solids, 1995, 213–214, 490–492.
- Imaoka M, Hasegawa H, Yasui I: ‘X-ray diffraction analysis on the structure of the glasses in the system PbO–SiO2’, J. Non-Cryst. Solids, 1986, 85, (3), 393–412.
- Fayon F, Landron C, Sakurai K, Bessada C, Massiot D: ‘Pb2+ environment in lead silicate glasses probed by Pb-LIII edge XAFS and 207Pb NMR’, J. Non-Cryst. Solids, 1999, 243, (1), 39–44.
- Ghigna P, Mustarelli P, Tomasi C, Quartarone E, Scavini M, Speghini A, Bettinelli M: ‘A combined nuclear magnetic resonance and X ray absorption fine structure study on the local structures of Ge and Pb in PbO–GeO2 glasses and their relationships with thermal properties and devitrification products’, J. Phys. Chem. B, 2002, 106, (38), 9802–9809.
- Wang PW, Zhang LP: ‘Structural role of lead in lead silicate glasses derived from XPS spectra’, J. Non-Cryst. Solids, 1996, 194, (1–2), 129–134.
- Bent JF, Hannon AC, Holland D, Karim MMA: ‘The structure of tin silicate glasses’, J. Non-Cryst. Solids, 1998, 232–233, 300–308.
- Zachariesen WH: ‘The atomic arrangement in glass’, J. Am. Ceram. Soc., 1932, 54, (10), 3841–3851.
- Ellison AJG, Sen S: ‘Role of Sb3+ as a network-forming cation in oxide glasses’, Phys. Rev. B, 2003, 67B, (5), 052203.
- Holland D, Hannon AC, Smith ME, Johnson CE, Thomas MF, Beesley AM: ‘The role of Sb5+ in the structure of Sb2O3–B2O3 binary glasses – an NMR and Mössbauer spectroscopy study’, Solid State Nucl. Magn. Reson., 2004, 26, (3–4), 172–179.
- Seki H, Satoh K, Satoh H: ‘A new adhesion process of glass to metal using the redox reaction’, J. Mater. Sci., 1996, 31, (18), 4891–4898.
- Humpston G, Jacobson D: ‘The joining environment’, Chap. 3, in ‘Principles of soldering’; 2004, Materials Park, OH, ASM International, 103–113.
- Yuan Z, Fan J, Li J, Ke J, Mukai K: ‘Surface tension of molten bismuth at different oxygen partial pressure with the sessile drop method’, Scand. J. Metall., 2004, 33, (6), 338–346.
- Zhang J, Li N: ‘Review of studies on fundamental issues in LBE corrosion’, Report LA-UR-04-0869, Los Alamos National Laboratory, Los Alamos, NM, USA, 2004.
- Pierce J, Kuty D, Larry J: ‘The chemistry and stability of ruthenium-based resistors’, Proc. 3rd Eur. Hybrid Microelectronics Conf., ISHM, Avignon, France, May 1981, 283–301.
- Claussen O, Rüssel C: ‘Thermodynamics of various polyvalent main group elements in a borosilicate glass melt’, J. Non-Cryst. Solids, 1997, 209, (3), 292–298.
- Kusz B, Trzebiatowski K: ‘Bismuth germanate and bismuth silicate glasses in cryogenic detectors’, J. Non-Cryst. Solids, 2003, 319, (3), 257–262.
- Khonthon S, Morimoto S, Arai Y, Ohishi Y: ‘Redox equilibrium and NIR luminescence of Bi2O3-containing glasses’, Opt. Mater., 2009, 31, 1262–1268.
- Singh SP, Karmakar B: ‘Oxidative control of surface plasmon resonance of bismuth nanometal in bismuth glass nanocomposites’, Mater. Chem. Phys., 2010, 119, (3), 355–358.
- Peng M, Wu B, Da N, Chen W, Chen D: ‘Bismuth-activated luminescent materials for broadband optical amplifier in WDM system’, J. Non-Cryst. Solids, 2008, 354, (12–13), 1221–1225.
- Ren J, Qiu J, Chen D, Hu X, Jiang X, Zhu C: ‘Luminescence properties of bismuth-doped lime silicate glasses’, J. Alloys Compd, 2008, 463, (1–2), L5–L8.
- Chi G, Zhou D, Song Z, Qiu J: ‘Effect of optical basicity on broadband infrared fluorescence in bismuth doped alkali metal germanate glasses’, Opt. Mater., 2009, 31, 945–948.
- Song JY, Park TJ, Choi SY: ‘Preparation and characterization of CuO doped Bi2O3–B2O3–BaO–ZnO glass system for transparent dielectric layer’, J. Non-Cryst. Solids, 2006, 352, (50–51), 5403–5407.
- Roberts GJ: ‘Bismuth containing lead free glass enamels and glazes of low silica content’, Patent US5326591, 1994.
- Hwang S, Kim Y, Park M, Kim H: ‘Devitrification of bismuth oxide seal frit for back-light unit lamp in LCD’, Molec. Cryst. Liquid Cryst., 2007, 470, 137–144.
- Coleman M, Winster A: ‘Silver migration in thick film conductors and chip attachment resins’, Microelectron. J., 1981, 12, (4), 23–29.
- Lee S, Hwang S, Cha M, Kim H: ‘Role of copper ion in preventing silver nanoparticles forming in Bi2O3–B2O3–ZnO glass’, J. Phys. Chem. Solids, 2008, 69, (5–6), 1498–1500.
- Sridharan S, Blonski R, Joyce IH, Korn GC, Anquetil J: ‘Durable glass enamel composition’, Patent US6936556, 2003.
- Sakoske GE: ‘Partially crystallizing enamel containing crystalline zinc borate seed material’, Patent US5677251, 1997.
- Sakoske GE, Ryan JW: ‘Partially crystallizing ceramic enamel composition containing bismuth silicate, and use thereof’, Patent USA5714420, 1998.
- Sakoske GE: ‘Partially crystallizing lead-free enamel composition for automobile glass’, Patent US6022624, 2000.
- Sakoske GE, Tunker G: ‘High durability low temperature lead free glass and enamel compositions with low boron content’, Patent US2002/0004443, 2002.
- Krohn MH, Hellmann JR, Shelleman DL, Pantano CG, Sakoske GE: ‘Effect of enameling on the strength and dynamic fatigue of soda–lime–silica float glass’, J. Am. Soc., 2002, 85, (10), 2507–2514.
- Maeder T, Jacq C, Ryser P: ‘Long term mechanical reliability of ceramic thick-film circuits and mechanical sensors under static load’,. Sens. Actuators A, 2012, 10.1016/j.sna.2012.05.031.
- Ram S, Narayan KA: ‘Controlled crystallization of PbO–Cr2O3–B2O3 glasses and a catalytic effect of Al2O3 for the growth of Pb2CrO5 microcrystals’, Indust. Eng. Chem. Res., 1987, 26, 1051–1055.
- Liu YH, Li JB, Liang JK, Luo J, Ji LN, Zhang JY, Rao GH: ‘Phase diagram of the Bi2O3–Cr2O3 system’, Mater. Chem. Phys., 2008, 112, (1), 239–243.
- Jacq C, Maeder T, Vionnet S, Ryser P: ‘Low-temperature thick-film dielectrics and resistors for metal substrates’, J. Eur. Ceram. Soc., 2005, 25, (12), 2121–2124.
- Bilinski M, Eisermann E, Jones W, Smetana KW, Unger M, Whitmarsh J: ‘Thick film pastes for the manufacture of low cost, insulated aluminium substrates for use as integrated heat sinks for high intensity LEDs’, Proc. XXXII Int. Conf. of IMAPS Poland Chapter, Pułtusk, Poland, September 2008, IMAPS, I12.
- Cole S: ‘Sintering of Ag–Pd in the presence of a reactive glass’, J. Am. Ceram. Soc., 1972, 55, (6), 296–299.
- Larry J, Rosenberg R, Uhler R: ‘Thick-film technology: an introduction to the materials’, IEEE Trans. Compon. Hybrids Manuf. Technol., 1980, CHMT 3, (2), 211–225.
- Taylor BE, Felten JJ, Larry JR: ‘Progress in and technology of low-cost silver containing thick-film conductors’, IEEE Trans. Compon. Hybrids Manuf. Technol., 1980, 3, (4), 504–517.
- Wang S, Huebner W: ‘Interaction of Ag/Pd metallization with lead and bismuth oxide-based fluxes in multilayer ceramic capacitors’, J. Am. Ceram. Soc., 1992, 75, (9), 2339–2352.
- Wang SF, Huebner W: ‘Interaction of silver/palladium electrodes with lead- and bismuth-based electroceramics’, J. Am. Ceram. Soc., 1993, 76, (2), 474–480.
- Inoue T: ‘Conductive paste for thick-film circuit’, Patent JP54060497, 1979.
- Nordstrom TV, Yost FG: ‘Sintering behavior of a reactively bonded thick film gold ink’, J. Electron. Mater., 1978, 7, (1), 109–122.
- Chung Y, Kim H: ‘Effect of oxide glass on the sintering behavior and electrical properties in Ag thick films’, IEEE Trans. Compon. Hybrids Manuf. Technol., 1988, 11, (2), 195–199.
- Rane S, Puri V, Amalnerkar D: ‘A study on sintering and microstructure development of fritless silver thick film conductor’, J. Mater. Sci., 2000, 11, 667–674.
- Rane SB, Seth T, Phatak GJ, Amalnerkar D, Ghatpande M: ‘Effect of inorganic binders on the properties of silver thick films’, J. Mater. Sci., 2004, 15, (2), 103–106.
- Takaoka K: ‘Gold thick film pastes’, in ‘Gold – science and applications’, (ed. C. Corti et al.), 279–286; 2010, Boca Raton, FL, CRC Press.
- Hrovat M, Kolar D: ‘Investigation in the Al2O3–Bi2O3–CuO system’, J. Mater. Sci. Lett., 1984, 3, (8), 659–662.
- Immovilli S, Morten B, Prudenziati M, Gualtieri A, Bersani M: ‘Interactions between bismuth oxide and ceramic substrates for thick film technology’, J. Mater. Res., 1998, 13, (7), 1865–1874.
- Hrovat M, Bernik S, Kolar D: ‘Phase equilibria in the RuO2–Bi2O3–PdO system’, J. Mater. Sci. Lett. 1988, 7, (6), 637–638.
- Iles G, Casale M: ‘Ruthenium oxide glaze resistors – new screen printing preparations for thick film circuitry’, Platin. Met. Rev., 1967, 11, (4), 126–129.
- Ansell M: ‘Conduction processes in thick film resistors. Part I’, Electrocompon. Sci. Technol. 1976, 3, (3), 131–140.
- Chiang Y, Silverman L, French R, Cannon R: ‘Thin glass film between ultrafine conductor particles in thick-film resistors’, J. Am. Ceram. Soc., 1994, 77, (5), 1143–1152.
- Adachi K, Kuno H: ‘Decomposition of ruthenium oxides in lead borosilicate glass’, J. Am. Ceram. Soc., 1997, 80, (5), 1055–1064.
- Vionnet S, Maeder T, Ryser P: ‘Firing, quenching and annealing studies on thick-film resistors’, J. Eur. Ceram. Soc., 2004, 24, (6), 1889–1892.
- Inokuma T, Taketa Y: ‘Control of electrical properties of RuO2 thick film resistors’, Act. Passive Electron. Compon., 1987, 12, (3), 155–166.
- Weissmann R, Wang C: ‘Glasses for high-resistivity thick-film resistors’, Adv. Eng. Mater., 2000, 2, (6), 359–362.
- Shante V, Kirkpatrick S: ‘An introduction to percolation theory’, Adv. Phys. 20, 325 (1971), 1971, 20, 325–357.
- Pike G, Seager C: ‘Electrical properties and conduction mechanisms of Ru-based thick-film (cermet) resistors’, J. Appl. Phys., 1977, 48, (12), 5152–5169.
- Ewen P, Robertson J: ‘A percolation model of conduction in segregated systems of metallic and insulating materials: application to thick film resistors’, J. Phys. D: Appl. Phys., 1981, 14, 2253–2268.
- Prudenziati M: ‘Electrical transport in thick film (cermet) resistors’, Electrocompon. Sci. Technol. 1983, 10, (4), 285–293.
- Dziedzic A: ‘Bibliography on electrical conduction in thick film resistors’, Microelectron. Reliab., 1991, 31, (2/3), 549–558.
- Grimaldi C, Maeder T, Ryser P, Strässler S: ‘Segregated tunneling percolation model for transport nonuniversality’, Phys. Rev. B, 2003, 68B, (2), 024207.
- Ambrosetti G, Johner N, Grimaldi C, Maeder T, Ryser P, Danani A: ‘Electron tunneling in conductor-insulator composites with spherical fillers’, J. Appl. Phys., 2009, 106, 016103.
- Murhty K, Kumar A: ‘Failure mechanisms in bismuth ruthenate resistor systems’, J. Mater. Sci., 1990, 1, (2), 61–71.
- Adachi K, Kuno H: ‘Effect of glass composition on the electrical properties of thick-film resistors’, J. Am. Ceram. Soc., 2000, 83, (10), 2441–2448.
- Dziedzic A: ‘Thick film resistors with IrO2 and CaIrxTi1–xO3 − examples of chemically reactive and unreactive systems’, Microelectron. J., 1988, 19, (6), 24–42.
- Tankiewicz S, Morten B, Prudenziati M, Golonka LJ: ‘IrO2-based thick-film resistors’, J. Appl. Phys., 2002, 91, (7), 4261–4266.
- Ryden WD, Lawson AW, Sartain CC: ‘Temperature dependance of the resistivity of RuO2 and IrO2’, Phys. Lett. A, 1968, 26A, (5), 209–210.
- Longo JM, Raccah PM, Goodenough JB: ‘Pb2M2O7−x (M = Ru, Ir, Re) preparation and properties of oxygen deficient pyrochlores’, Mater. Res. Bull., 1969, 4, (3), 191–202.
- Ryden WD, Lawson AW, Sartain CC: ‘Electrical transport properties of IrO2 and RuO2’, Phys. Rev. B, 1970, 1B, (4), 1494–1500.
- Bouchard RJ, Gillson JL: ‘A new family of bismuth – precious metal pyrochlores’, Mater. Res. Bull., 1971, 6, (8), 669–680.
- Horowitz HS, Longo JM, Lewandowski JT: ‘New oxide pyrochlores: A2[B2−xAx]O7−y (A = Pb, Bi; B = Ru, Ir)’, Mater. Res. Bull., 1981, 16, (5), 489–496.
- Beyerlein RA, Horowitz HS, Longo JM, Leonowicz ME, Jorgensen JD, Rotella FJ: ‘Neutron diffraction investigation of ordered oxygen vacancies in the defect pyrochlores, Pb2Ru2O6·5 and PbTlNb2O6·5’, J. Solid State Chem., 1984, 51, (2), 253–265.
- Schuler M, Kemmler Sack S: ‘Systeme Bi2−xBxRu2O7−x (B = Mn, Co, Ni, Cu, Zn, Cd, Mg, Ca, Sr)’, J. Less-Common Met., 1984, 102, (1), 105–112.
- Dziedzic A, Golonka L: ‘Electrical properties of conductive materials used in thick film resistors’, J. Mater. Sci., 1988, 23, (9), 3151–3155.
- Yi K, Lee K, Chung K, Um W, Lee H, Song J, Lee I: ‘Conductive powder preparation and electrical properties of RuO2 thick film resistors’, J. Mater. Sci., 1997, 8, 247–251.
- Gurunathan K, Vyawahare NP, Phatak GJ, Amalnerkar DP: ‘Solution route synthesis and characterization of nanocrystalline Bi2Ru2O7 for usage in resistor paste application’, J. Mater. Sci., 2005, 16, (3), 159–168.
- Pagnaer J, Nelis D, Mondelaers D, Vanhoyland G, D’Haen J, van Bael MK, van den Rul H, Mullens J, van Poucke LC: ‘Synthesis of RuO2 and SrRuO3 powders by means of aqueous solution gel chemistry’, J. Eur. Ceram. Soc., 2004, 24, (6), 919–923.
- Goodenough JB: ‘Metallic oxides’, Prog. Solid State Chem. 1971, 5, (C), 145–399.
- Morten B, Masoero A, Prudenziati M, Manfredini T: ‘Evolution of ruthenate-based thick film cermet resistors’, J.Phys. D: Appl. Phys., 1994, 27, (10), 2227–2235.
- Rao M, Giramkar V, Phatak G, Amalnerkar D, Sastri K, Krishnamurthy V: ‘Microstructure and electrical properties of ‘pre-sintered bismuth ruthenate-glass composite’ based thick film paste for resistor application’, Int. J. Electron., 2005, 451–456.
- Prabhu A, Fuller G, Vest R: ‘Solubility of RuO2 in a Pb borosilicate glass’, J. Am. Ceram. Soc., 1974, 57, (9), 408–409.
- Palanisamy P, Sarma DHR, Vest RW: ‘Solubility of ruthenium dioxide in lead borosilicate glasses’, J. Am. Ceram. Soc., 1989, 72, (9), 1755–1756.
- Adachi K, Iida S, Hayashi K: ‘Ruthenium clusters in lead-borosilicate glass in thick film resistors’, J. Mater. Res., 1994, 9, (7), 1866–1878.
- Vionnet-Menot S, Maeder T, Grimaldi C, Jacq C, Ryser P: ‘Properties and stability of thick-film resistors with low processing temperatures − effect of composition and processing parameters’, Proc.15th Eur. Microelectronics and Packaging Conf., Brugge, Belgium, June 2005, IMAPS, P2.05, 571–576.
- Řehák B, Frumar M, Koudelka L: ‘Effect of atmosphere on the electrical conductivity of RuO2 and ruthenate layers’, J. Mater. Sci. Lett., 1984, 3, 1011–1014.
- Nakano T, Yamaguchi T: ‘Effect of O2 concentration in firing atmosphere on resistance of RuO2-glass thick-film resistors’, J. Am. Ceram. Soc., 1995, 78, (6), 1703–1704.
- Ryll T, Brunner A, Ellenbroek S, Bieberle Hütter A, Rupp JLM, Gauckler LJ: ‘Electrical conductivity and crystallization of amorphous bismuth ruthenate thin films deposited by spray pyrolysis’, Phys. Chem. Chem. Phys., 2010, 12, (42), 13933–13942.
- Prudenziati M, Morten B, Moro L, Olumekor L, Tombesi A: ‘Interactions between thick-film resistors and terminations: the role of bismuth’, J. Phys. D: Appl. Phys., 1986, 19, (2), 275–282.
- Hrovat M, Bernik S, Holc J, Kolar D: ‘Subsolidus phase equilibria in the RuO2–Bi2O3–ZnO and RuO2–Al2O3–ZnO systems’, J. Mater. Sci. Lett., 1996, 15, (4), 336–338.
- Hrovat M, Holc J, Kolar D: ‘Phase equilibria in RuO2–TiO2–Al2O3 and RuO2–TiO2–Bi2O3 systems’, J. Mater. Sci. Lett., 1993, 12, (23), 1858–1860.
- Bernik S, Hrovat M, Kolar D: ‘Phase equilibria in the RuO2–Bi2O3–CuO system’, J. Mater. Sci. Lett., 1991, 10, (1), 69–70.
- Hrovat M, Samardžia Z, Holc J, Kolar D: ‘Phase equilibria in the RuO2–Bi2O3–NiO and RuO2–Al2O3–NiO systems’, J. Mater. Sci. Lett., 1995, 14, (7), 506–507.
- Hrovat M, Bernik S, Kolar D: ‘Phase equilibria in the RuO2–PbO–Al2O3 and RuO2–PbO–CuO systems’, J. Mater. Sci. Lett., 1993, 12, (23), 1848–1850.
- Chen T, Djeu T, Li T: ‘Excess noise in nitrogen fired thick film resistors’, Int. J. Hybrid Microelectron., 1982, 5, (2), 92–97.
- Browne E, Walton B: ‘An air firing base metal resistor and conductor system for low-cost thick-film circuit manufacture’, Electrocompon. Sci. Technol., 1981, 8, (1–2), 61–65.
- Grebenkina V, Smolin M: ‘Thick-film cobalt molybdate-base resistors for high-voltage voltage dividers’, Powder Metall. Met. Ceram., 1994, 33, (1–2), 95–96.
- Akulova L, Vlasova M, Voropaeva I, Horpyakov O: ‘Behaviour of molybdenum diboride in resistive thick film’, J. Mater. Sci., 1992, 27, (12), 3293–3296.
- Achmatowicz S, Jakubowska M, Zwierkowska E, Primovitch M: ‘Low cost thick film resistive compositions without the use of noble metals’, Microelectron. Int., 1995, 12, (2), 23–25.
- Achmatowicz S, Jakubowska M, Zwierkowska E, Pitt K, Primovitch M: ‘A robust low cost thick film system for the consumer market’, J. Mater. Sci., 1999, 10, 487–490.
- Jakubowska M, Kalenik J, Miecznik A, Zwierkowska E: ‘Electrical and stability properties of molybdenum oxide based thick film resistors’, Proc. XXVII Int. Conf. of IMAPS Poland Chapter, Podlesice, Poland, September 2003, IMAPS, Vol. 27, 174–176.
- He L, Anderson JR, Franzen HF, Johnson DC: ‘Electrocatalysis of anodic oxygen transfer reactions: Bi3Ru3O11 electrodes in acidic media’, Chem. Mater., 1997, 9, (3), 715–722.
- Strobel R, Metz HJ, Pratsinis S: ‘Brilliant yellow, transparent pure, and SiO2-coated BiVO4 nanoparticles made in flames’, Chem. Mater., 2008, 20, (20), 6346–6351.
- Mehring M: ‘From molecules to bismuth oxide-based materials: Potential homo- and heterometallic precursors and model compounds’, Coordin. Chem. Rev., 2007, 251, (7–8), 974–1006.
- Venkatasubramanian R, Siivola E, Colpitts T, O’Quinn B: ‘Thin-film thermoelectric devices with high room-temperature figures of merit’, Nature, 2001, 413, 597–602.
- Leontsev SO, Eitel RE: ‘Progress in engineering high strain lead-free piezoelectric ceramics’, Sci. Technol. Adv. Mater., 2010, 11, (4), 044302.
- Jo W, Erdem E, Eichel RA, Glaum J, Granzow T, Damjanovic D, Rödel J: ‘Effect of Nb-donor and Fe-acceptor dopants in (Bi1/2Na1/2)TiO3−BaTiO3− (K0·5Na0·5)NbO3 lead-free piezoceramics’, J. Appl. Phys., 2010, 108, (1), 014110.
- Takahashi T, Iwahara H: ‘Oxide ion conductors based on bismuth sesquioxide’, Mater. Res. Bull., 1978, 13, (2), 1447–1453.
- Azad AM, Larose S, Akbar SA: ‘Bismuth oxide-based solid electrolytes for fuel cells’, J. Mater. Sci., 1994, 29, (16), 4135–4151.
- Shuk P, Wiemhöfer HD, Guth U, Göpel W, Greenblatt M: ‘Oxide ion conducting solid electrolytes based on Bi2O3’, Solid State Ion., 1996, 89, (3–4), 179–196.
- Sammes NM, Tompsett GA, Näfe H, Aldinger F: ‘Bismuth based oxide electrolytes − structure and ionic conductivity’, J. Eur. Ceram. Soc., 1999, 19, (10), 1801–1826.
- Brinkman K, Iijima T, Takamura H: ‘The oxygen permeation characteristics of Bi1 xSrxFeO3 mixed ionic and electronic conducting ceramics’, Solid State Ion., 2010, 181, (1–2), 53–58.
- Maeda H, Tanaka Y, Fukutomi M, Asano T, Togano K, Kumakura H, Uehara M, Ikeda S, Ogawa K, Horiuchi S, Matsui Y: ‘New high-Tc superconductors without rare earth element’, Physica C, 1988, 153–155C, (1), 602–607.
- Majewski P: ‘BiSrCaCuO high-Tc superconductors’, Adv. Mater., 1994, 6, (6), 460–469.
- Hinks DG: ‘The nature of superconductivity in Ba1−xKxBiO3’, MRS Bull., 1990, 15, 55–59.
- Kobayashi M, Ishii M, Harada K, Yamaga I: ‘Bismuth silicate Bi4Si3O12, a faster scintillator than bismuth germanate Bi4Ge3O12’, Nucl. Instrum. Methods Phys. Res. A, 1996, 372A, 45–50.
- Vaithianathan V, Claude A, Santhanaraghavan P, Ramasamy P: ‘On the energy and time resolution measurements of bismuth germanium silicon oxide (BGSO) crystal grown by Czochralski technique’, J. Cryst. Growth, 2005, 273, 481–488.
- Kwok CK, Vijay DP, Sheshu DB, Parikh NR, Hill EA: ‘Conducting oxide electrodes for ferroelectric films’, Integr. Ferroelectr., 1993, 3, (2), 121–130.
- Park BH, Kang BS, Bu SD, Noh TW, Lee J, Jo W: ‘Lanthanum substituted bismuth titanate for use in non-volatile memories’, Nature, 1999, 401, 682–684.
- Amanuma K, Hase T, Miyasaka Y: ‘Preparation and ferroelectric properties of SrBi2Ta2O9 thin films’, Appl. Phys. Lett., 1995, 66, (2), 221–223.
- Zhang ST, Sun B, Yang B, Chen YF, Liu ZG, Ming NB: ‘SrBi4Ti4O15 thin films of Ti containing bismuth-layered-ferroelectrics prepared by pulsed laser deposition’, Mater. Lett., 2001, 47, (6), 334–338.
- Anon: ‘Minerals yearbook’; 2010 Reston, VA, USA, US Geological Survey.
- Yang X, Liu H, Zhao H, Zhang X, Liang X, Xiang W: ‘Fabrication and third order optical nonlinearities of Na2O–B2O3–SiO2 glasses containing metallic Bi, Bi2O3 and Bi2S3 crystals’, Mater. Chem. Phys., 2011, 192, (1–2), 121–129.