Abstract
Iron, a source of oxidative stress, plays a major role in the pathology of neurodegenerative disease. In Alzheimer's disease, the hippocampus is vulnerable to oxidative stress, leading to impairment in memory formation. In our previous study, a brain oxidative reaction was induced after intraperitoneal injection of ferric nitrilotriacetate (Fe-NTA). However, since only a small amount of iron reached the brain in the previous study, Fe-NTA was administered into the hippocampus using an osmotic pump in this study.
After continuous injection of Fe-NTA for 2 weeks, a high level of apoptotic change was induced in the hippocampus, in accordance with the iron localization. After injection for 4 weeks, the hippocampus was totally destroyed. A small amount of iron infiltrated into the cerebral cortex and the striatum, and deposition was observed at the choroid plexus and ependymal cells. However, no apoptotic reaction or clear tissue injury was observed in these areas. In addition, muscarinic acetylcholine receptors (M1, M2, and M4) were decreased in both the cortex and hippocampus while it increased in the striatum. Thus, the hippocampus is likely vulnerable to oxidative stress from Fe-NTA, and the oxidative stress is considered to bring the disturbance in the muscarinic acetylcholine receptors.
Introduction
In the pathology of neurodegenerative diseases such as Alzheimer's disease (AD) and Parkinson's disease, oxidative stress plays a major role. Plausible sources of oxidative stress in the AD brain include beta-amyloid deposition, activated microglia, senescent mitochondria, and neural sequestration of redox-active iron above levels encountered in the course of normal aging.Citation1 Although iron is essential in the brain, excessive free iron generates oxidative stress, leading to neuronal diseases linked to aging.Citation2 In addition, recent studies revealed that a disturbance in brain iron homeostasis and dysfunction in catalase for scavenging hydrogen peroxide (H2O2) contribute to the pathogenesis of neurodegenerative diseases,Citation3,Citation4 suggesting that iron metabolism and a balance of redox reactions regarding iron may be pivotal in inducing oxidative stress in the brain.
Clinical symptoms of AD in the early stage are mild cognitive dysfunction and memory deficits, indicating that hippocampal synaptic transmission and plasticity are impaired because the hippocampus is indispensable for memory formation.Citation5 Using transgenic animals relevant to AD, numerous plaques in the hippocampus have been observed,Citation6,Citation7 and excitatory synaptic transmission in the hippocampus was impaired,Citation8 leading to the conclusion that the hippocampus may be vulnerable to oxidative stress linked to pathological changes in AD.
Ferric nitrilotriacetate (Fe-NTA) is an iron chelate that can induce strong oxidative stress via its unique characteristics.Citation9 The oxidative stress caused by intermittent injection of Fe-NTA causes diabetes as well as kidney carcinoma.Citation10,Citation11 Recently, we demonstrated that brain oxidative changes were induced after intraperitoneal (i.p.) injection of Fe-NTA in rats.Citation12 In that study, the level of heme oxygenase (HO)-1 mRNA was raised, and lipid peroxidation was exaggerated, as well as changes in muscarinic acetylcholine receptors (mAChRs) in the brain. However, since iron accumulating in the brain was sparse, the effects of Fe-NTA in the brain are considered much weaker compared to those in peripheral organs such as the liver and kidneys after peripheral injection of Fe-NTA. In this study, a low dose and small amount of Fe-NTA was injected directly into the hippocampus for several weeks using an osmotic pump, and the direct effect of Fe-NTA on the hippocampus was observed. In addition, we evaluated the changes of mAChRs, which were affected by i.p. injection of Fe-NTA in our previous report.
Methods
Animal treatment
Male DDY mice were obtained from Shimizu Laboratory Supplies (Kyoto, Japan) at 8 weeks of age. The animals were housed in groups at 25°C with food and water available ad libitum. All experimental procedures were approved by the Okayama University Animal Care Committee.
Fe-NTA solution was prepared by the method of Awai et al.Citation11 Osmotic minipumps (Model 2004, Alzet, Cupertino, CA, USA), which deliver their contents at a rate of 0.25 µl/hour for up to 4 weeks, were each connected by a polyethylene catheter to a stainless-steel cannula (Brain Infusion Kit 3, Alzet) and loaded Fe-NTA solution (Fe 0.1 mg/ml) for 2 or 4 weeks. The concentration of Fe-NTA solution was determined from a preliminary experiment, in which we compared Fe concentrations of 1, 0.5, or 0.1 mg/ml, resulting in a survival rate of more than 80% in groups administered Fe 0.1 mg/ml of Fe-NTA solution, which was selected. The concentration was 10 times lower than that used for ip injection, and the total amount of Fe was 16.8 µg over 4 weeks.
Mice were anesthetized by ip injection of pentobarbital (50 mg/kg), and their skulls were carefully exposed. The Brain Infusion Kit was stereotaxically implanted into the right hippocampus. The stereotaxic coordinate used was 1.5 mm posterior to the bregma, 1.5 mm lateral to the midline, and 2.0 mm from the surface of the skull, using a flat skull position.Citation13 The kit was attached to the skull with dental cement and connected to the osmotic minipump, which was filled with normal saline solution (NSS) or Fe-NTA solution, prepared as described above. The pump was implanted under the skin of the mouse's back, and an antibiotic (ampicilline 50 mg/kg, Sigma, St Louis, MO, USA) was injected subcutaneously.
In order to compare the oxidative injury caused by Fe-NTA with other free radical inducers, we used H2O2 because it has been widely used as a reactive oxygen species. We administered 0.01% of H2O2 for 2 weeks in the same manner as Fe-NTA injection.
HE staining
After 2 or 4 weeks of injection, the mice were anesthetized again and perfused with NSS and 4% of paraformaldehyde in phosphate buffer, pH 7.6. The brains were removed and postfixed overnight in a fixative. After fixation, the brains were embedded in paraffin and cut into 8 µm sections, which were adhered to poly-l-lysine-coated slides by heating them at 60°C for 15–20 minutes. After deparaffinization and rehydration, the tissue was stained with HE solution.
Iron histochemistry
The brain sections were deparaffinized and immersed in a potassium ferrocyanide mixture (Perl's solution containing 1 part of 10% aqueous, potassium ferrocyanide and a half part of 10% hydrochloric acid), and rinsed. The stain was emphasized by incubation for 20 minutes in 0.5% of diaminobenzidine (DAB) in 0.1 M phosphate-buffered saline (PBS) and 15 minutes in the same medium including 0.005% of H2O2, followed by rinsing in water. After that, sections were counterstained with toluisine blue.
TUNEL staining
Apoptotic cells were stained using a MEBSTAIN Apoptosis Kit II (MBL, Nagoya, Japan). After deparaffinization, sections were rinsed with PBS, followed by a treatment with proteinase K for 30 minutes at 37°C. After washing, they were incubated with TdT solution containing TdT and biotin-conjugated dUTP for 60 minutes at 37°C, and then with TB buffer for 15 minutes at room temperature. They were washed again, treated with blocking solution, incubated with avidin-fluorescein isothiocyanate solution for 30 minutes at 37°C, followed by washing with PBS.
Realtime reverse transcriptase polymerase chain reaction and dot blot analysis
Twelve mice were used for reverse transcriptase polymerase chain reaction (RT-PCR), and eight out of them were used for dot blot analysis. Half of them were used for injection of Fe-NTA solution as described above, and rest of them were for NSS. After administration for 2 weeks, each mouse was perfused with NSS, and the brains were removed. The contralateral cortex, hippocampus and striatum were removed and immersed in RNA later (Qiagen, Hilden, Germany) for RT-PCR, and the ipsilateral hippocampus was removed and frozen in liquid nitrate for dot blot analysis.Citation14
Using samples for RT-PCR, total RNA was extracted with phenol. Elimination of genomic DNA and reverse transcription were performed with QuantiTect (Qiagen, Hilden, Germany). Primers used for the amplification of cDNA were summarized in . Quantitative real-time PCR for M1, M2, and M4 muscarinic receptors was performed using Miniopticon (Bio-rad, Hercules, CA, USA) and SYBR Premix Taq II (Takara, Tokyo, Japan). The reactions were incubated at 95°C for 3 minutes, followed by a cycling protocol consisting of two stages: 10 seconds at 95°C and 30 seconds at 60°C. After 40 cycles of PCR, melting curve analysis was routinely performed. The target cDNA copy number was normalized to that of beta-actin amplified from the same cDNA sample.
Table 1. Mouse gene-specific PCR primers
The cell lysates (2 µg), obtained from the samples for dot blot, were blotted onto a membrane. The membrane was incubated with a mouse anti-4-HNE monoclonal antibody (1:2000; GE Healthcare, Piscataway, NJ, USA) for 1 hour at room temperature. The membrane was treated with chemiluminescent reagents (GE Healthcare), and the signals were detected using x-ray film. After washing the membrane with immunopure IgG elution buffer (Pierce, Rockford, IL, USA), beta-actin monoclonal antibody (1:2000, clone AC-74; Sigma), the secondary antibody, and the detection agents described above were administered. Dot densities were quantified with NIH image software and the expression level of 4-HNE was normalized with that of beta-actin. Data were analyzed by Mann–Whitney test. Significance was taken as P < 0.05.
Results
Tissue staining after injection for 2 weeks
HE staining
After continuous injection of Fe-NTA for 2 weeks, the hippocampus was surrounded with inflammatory cells, and severe atrophy of the hippocampus was observed on the ipsilateral side of Fe-NTA injection (A). In the contralateral hippocampus, there was no significant change (B).
Figure 1. Photomicrographs of HE (A, B), Peal's (C, D), and TUNEL staining (E) after continuous injection of Fe-NTA for 2 weeks. Note that robust inflammation was induced in and around the hippocampus. An apoptotic change was induced in the hippocampus, but not in the vicinity. (Original magnification ×40.)
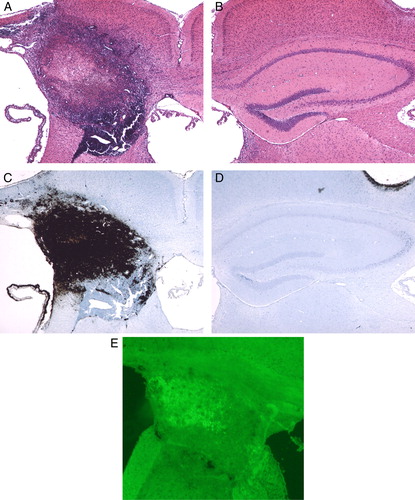
Perl staining
Deposition of high-level iron was observed mainly on the hippocampal tissue. The iron deposition reached the corpus callosum, the cerebral cortex, and a part of the fimbria of hippocampus. Although iron infiltrated into the cortex and the fimbria, it did not go over the corpus callosum (C). In the contralateral hippocampus, no deposition of iron was observed (D).
TUNEL staining
Positive cells were observed, mainly corresponding to iron localization in the hippocampus. Although iron was observed on part the cerebral cortex and the fimbria of the hippocampus, there were no cells positive for apoptotic changes (E).
Tissue staining after injection for 4 weeks
HE staining
The hippocampal structure was totally destroyed, and it was replaced with a mass of inflammatory cells. However, there were far fewer structural changes in the vicinity of the hippocampal area, such as the striatum, corpus callosum, and cerebral cortex (A and B).
Figure 2. Photomicrographs of HE (A, B, C), Peal's (D, E, F), and TUNEL staining (G, H) after continuous injection of Fe-NTA for 4 weeks. The hippocampus was totally destroyed and replaced by inflammatory cells (A, B). High-level iron accumulated at a marginal area of the hippocampal space, and lower level iron was observed on the choroid plexus and ependymal cells of the third ventricle (D, E). Localization of TUNEL-positive cells corresponded to that of a higher level of iron in the hippocampal space (G, H). (Original magnification ×40 for B, C, E, F, H, and ×100 for A, D, G.)
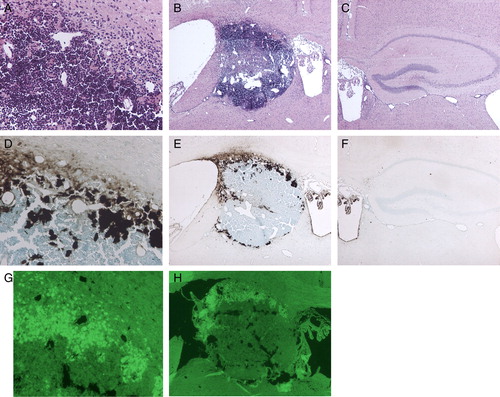
Perl staining
Although iron deposited at a marginal area of the hippocampal space, the iron did not injure the striatum or the cortex. Also, iron deposition was observed on the both choroid plexus and ependymal cells of the third ventricle, and morphological changes were limited in and around both areas. Although iron filtrated into the cerebral cortex, it did not invade into the brain parenchyma (D and E).
TUNEL staining
Positive signals were observed corresponding to iron distribution at a marginal area of the hippocampal space. However, in other neighboring areas, such as the cortex, striatum, corpus callosum, choroid plexus, and ependymal cells, no cells positive for apoptosis were observed (G and H).
In the contralateral hippocampus, there was no significant change (C and F).
Dot blot analysis
After continuous injection of Fe-NTA for 2 weeks, the level of 4-HNE in the hippocampus was significantly higher than that after NSS injection ().
Changes after continuous injection of H2O2
H2O2, injected at the same point as Fe-NTA, induced apoptotic changes in cells in the striatum as well as the hippocampus, while the damage in the hippocampus was much less than that after Fe-NTA injection (A–C). D is a high magnification picture of white rectangular area of C. The concentration of the TUNEL-positive cells in the striatum was at almost the same level as that in the hippocampus after injection of H2O2 (D).
Figure 4. Photomicrographs of HE (A, B) and TUNEL staining (C, D) after continuous injection of H2O2 for 2 weeks. (D) is a high magnification picture of white rectangular area of Fig. (C). H2O2 induced apoptotic changes in cells in the striatum as well as the hippocampus, while the damage in the hippocampus was much less than that after Fe-NTA injection. (Original magnification; ×40 for A, B, C, and ×100 for D.)
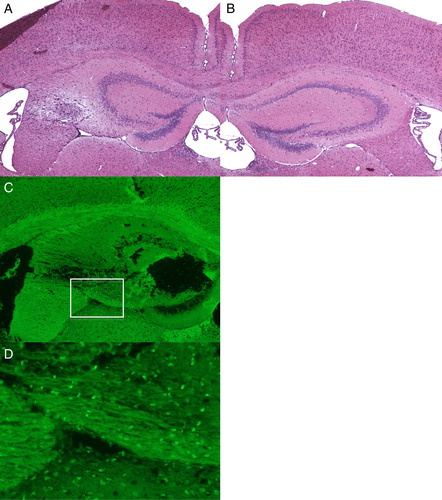
Real-time RT-PCR
In both the cortex and hippocampus, levels of all three kinds of mAChRs were decreased after the Fe-NTA injection significantly (A–F). Especially, M1 mAChR was deteriorated with the Fe-NTA, of which the average level was 17.7 % of NSS group (D). On the contrary, each average of three kinds of mAChRs increased in the striatum (G–I), and there were significant differences in M1 and M4 (G and I).
Figure 5. Changes in levels of M1, M2, and M4 mAChRs in the cortex, hippocampus, and striatum. In both the cortex and hippocampus, levels of all three kinds of mAChRs were decreased after the Fe-NTA injection significantly (A–F). Especially, M1 mAChR was deteriorated with the Fe-NTA, of which the average level was 17.7% of NSS group (D). On the contrary, each average of three kinds of mAChRs increased in the striatum (G–I), and there were significant differences in M1 and M4 (G, I).
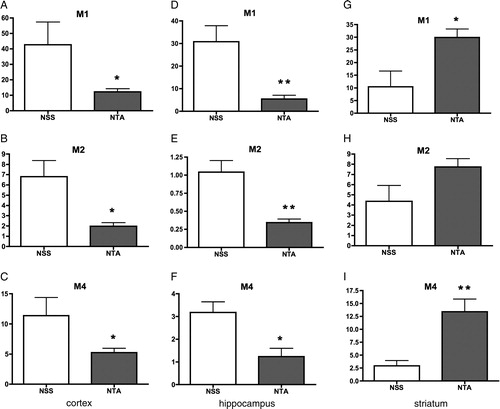
Discussion
In this study, the hippocampus was totally destroyed with continuous injection of Fe-NTA for up to 4 weeks, and a large quantity of iron deposition was observed in the hippocampal area. Overall, distribution of ferric iron corresponded to localization of TUNEL-positive cells on the hippocampal tissue. The level of 4-HNE in the hippocampus was raised, indicating that an oxidative change was induced in the hippocampus. Oxidative stress from iron is known to induce a neuronal loss and an apoptotic changes in the hippocampus.Citation15,Citation16 In our previous report, the iron deposition was sparse, and the apoptotic change was not observed although oxidative change was induced.Citation12 Therefore, it is considered that oxidative mechanisms causing apoptosis were evoked by the iron from Fe-NTA injected into the brain in our study.
Previously, we reported changes in brain mAChRs after ip injection of Fe-NTA.Citation12 In this experiment, the changes appeared clearly by the direct injection of Fe-NTA into the brain. Surprisingly, the reaction of the mAChRs of the striatum was contrary to those of both the cortex and hippocampus. Before the experiment, it was speculated that all kinds of mAChRs were deteriorated with oxidative stress, such as a decrease in muscarinic recptor binding in elderly people.Citation17 In addition, mAChRs in both the cortex and hippocampus are known to have an important role in brain function.Citation18 However, its role in the striatum is unclear. The increase in the striatum mAChRs may be compensatory reaction to the disturbance in other parts of the brains such as the cortex and hippocampus. In any case, further experiments are necessary to clarify this mechanism.
In AD, significant neuronal loss is observed in the hippocampal CA1 region.Citation19 In older mice, significant oxidative changes were induced in the CA1 region,Citation20 suggesting that pathological changes in the hippocampus are involved in neurodegenerative diseases. In other oxidative conditions, selective vulnerability has been reported in the hippocampus,Citation21 and in the CA1 region.Citation22 In our study, although the regional selective vulnerability to the oxidative stress from H2O2 was not observed, the hippocampus may be markedly vulnerable to oxidative stress from Fe-NTA. This result is in accordance with the previous study, in which iron accumulation was shown to be an important contributor toward the oxidative damage of AD.Citation23,Citation24
References
- Schipper HM. Heme oxygenase-1 in Alzheimer disease: a tribute to Moussa Youdim. J Neural Transm 2010;118:381–7.
- Levenson CW, Tassabehji NM. Iron and ageing: an introduction to iron regulatory mechanisms. Ageing Res Rev 2004;3:251–63.
- Altamura S, Muckenthaler MU. Iron toxicity in diseases of aging: Alzheimer's disease, Parkinson's disease and atherosclerosis. J Alzheimers Dis 2009;16:879–95.
- Rival T, Page RM, Chandraratna DS, Sendall TJ, Ryder E, Liu B. Fenton chemistry and oxidative stress mediate the toxicity of the beta-amyloid peptide in a Drosophila model of Alzheimer's disease. Eur J Neurosci 2009;29:1335–47.
- Seabrook GR, Rosahl TW. Transgenic animals relevant to Alzheimer's disease. Neuropharmacology 1999;38:1–17.
- Jolas T, Zhang XS, Zhang Q, Wong G, Del Vecchio R, Gold L. Long-term potentiation is increased in the CA1 area of the hippocampus of APP(swe/ind) CRND8 mice. Neurobiol Dis 2002;11:394–409.
- Strazielle C, Sturchler-Pierrat C, Staufenbiel M, Lalonde R. Regional brain cytochrome oxidase activity in beta-amyloid precursor protein transgenic mice with the Swedish mutation. Neuroscience 2003;118:1151–63.
- Fitzjohn SM, Doherty AJ, Collingridge GL. The use of the hippocampal slice preparation in the study of Alzheimer's disease. Eur J Pharmacol 2008;585:50–9.
- Kawabata T, Awai M, Kohno M. Generation of active oxygen species by iron nitrilotriacetate (Fe-NTA). Acta Med Okayama 1986;40:163–73.
- Li JL, Okada S, Hamazaki S, Ebina Y, Midorikawa O. Subacute nephrotoxicity and induction of renal cell carcinoma in mice treated with ferric nitrilotriacetate. Cancer Res 1987;47:1867–9.
- Awai M, Narasaki M, Yamanoi Y, Seno S. Induction of diabetes in animals by parenteral administration of ferric nitrilotriacetate. A model of experimental hemochromatosis. Am J Pathol 1979;95:663–73.
- Nakatsuka I, Maeda S, Andoh T, Hayashi Y, Mizuno R, Higuchi H. Oxidative changes in the rat brain by intraperitoneal injection of ferric nitrilotriacetate. Redox Rep 2009;14:109–14.
- Dolev I, Michaelson DM. A nontransgenic mouse model shows inducible amyloid-beta (Abeta) peptide deposition and elucidates the role of apolipoprotein E in the amyloid cascade. Proc Natl Acad Sci USA 2004;101:13909–14.
- Glowinski J, Iversen LL. Regional studies of catecholamines in the rat brain. I. The disposition of [3H]norepinephrine, [3H]dopamine and [3H]dopa in various regions of the brain. J Neurochem 1966;13:655–69.
- Bostanci MO, Bas O, Bagirici F. Alpha-tocopherol decreases iron-induced hippocampal and nigral neuron loss. Cell Mol Neurobiol 2010;30:389–94.
- Liu R, Liu W, Doctrow SR, Baudry M. Iron toxicity in organotypic cultures of hippocampal slices: role of reactive oxygen species. J Neurochem 2003;85:492–502.
- White P, Hiley CR, Goodhardt MJ, Carrasco LH, Keet JP, Williams IE. Neocortical cholinergic neurons in elderly people. Lancet 1977;1:668–71.
- Volpicelli LA, Levey AI. Muscarinic acetylcholine receptor subtypes in cerebral cortex and hippocampus. Prog Brain Res 2004;145:59–66.
- West MJ, Coleman PD, Flood DG, Troncoso JC. Differences in the pattern of hippocampal neuronal loss in normal ageing and Alzheimer's disease. Lancet 1994;344:769–72.
- Hayakawa N, Yokoyama H, Kato H, Araki T. Age-related alterations of oxidative stress markers in the mouse hippocampal CA1 sector. Exp Mol Pathol 2008;85:135–40.
- Haces ML, Montiel T, Massieu L. Selective vulnerability of brain regions to oxidative stress in a non-coma model of insulin-induced hypoglycemia. Neuroscience 2010;165:28–38.
- Wang X, Zaidi A, Pal R, Garrett AS, Braceras R, Chen XW. Genomic and biochemical approaches in the discovery of mechanisms for selective neuronal vulnerability to oxidative stress. BMC Neurosci 2009;10:12.
- Smith MA, Harris PL, Sayre LM, Perry G. Iron accumulation in Alzheimer disease is a source of redox-generated free radicals. Proc Natl Acad Sci USA 1997;94:9866–8.
- Sayre LM, Perry G, Harris PL, Liu Y, Schubert KA, Smith MA. In situ oxidative catalysis by neurofibrillary tangles and senile plaques in Alzheimer's disease: a central role for bound transition metals. J Neurochem 2000;74:270–9.