Abstract
In this work, the authors present experimental results of tunable amplified spontaneous emission (ASE) and random lasing (RL) emission wavelength, which mechanism is based on Rhodamine 6G (Rh6G) aggregates formation. Dye concentration controlled light amplification has been applied for RL and ASE on Rhodamine-doped biopolymeric layers like modified deoxyribonucleic acid (DNA) or starch. Additionally, measurements of ASE from water suspension with and without phosphatidylcholine (PC) lipids, confirmed Rhodamine aggregates role on different wavelength emission of ASE and RL. There have been acquired wide tuning of lasing equal to 35 nm observed for different studied biological systems.
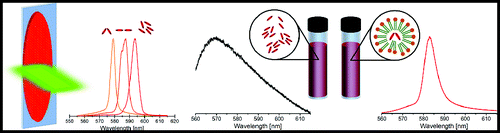
Introduction
Rhodamine 6G is one of the most efficient fluorescent dye, on which studies are continuing without interruption from the middle of past century.Citation1–Citation5 From mid-60s, there is an interest in light amplification, stimulated emission or lasing from Rhodamine 6G (Rh6G) including principles of mechanisms of light emission taking place in this particular dye like aggregation, triplet emission or quenching.Citation2,Citation4,Citation6–Citation12 Rhodamine proved to be very efficient compound in flash-lamp induced dye lasing for tunable continuous-wavelength lasers successfully used until now, and accounted as a classic representation of dye lasers.Citation13,Citation14
Another feature of Rhodamine is related to dye aggregation process, whereby fluorescence emission is shifting to the longer wavelengths from 550 nm emission for monomer, through low intensity J-type aggregates with fluorescence emission around 575 nm, H-type dimers quenching fluorescence up to higher order aggregates, which emit light at around 600 nm.Citation15 Changes in fluorescence spectra because of aggregation process and involvement of molecular aggregates in the emission spectra was also postulated by Brand et al. and Bujdak et al., where J- and H-aggregates dimer rearrangement has been measured leading to description of aggregation kinetics.Citation16,Citation17 Shift in fluorescence emission was also seen in sol–gel Rh6G/TiO2 thin films.Citation18 In this case, larger aggregates were responsible for a fluorescence emission equal to 650 nm off the central wavelength.
In this work, the authors show that by changing the dye concentration they are able to tune the amplified spontaneous emission (ASE) and random lasing (RL) emission wavelength because of the presence of above described different types of aggregates.
Random lasing term stands for generation of lasing action based on spontaneously formed nano- and micro-sized optical resonators, where light is being multiple scattered with gain on dispersing medium. High efficiency of light amplification based on Rh6G has been successfully introduced into RL topic. From the beginning of XXI century, Rhodamine becomes to be a compound responsible for gain in RL sufficient to study distributed systems, studies on TiO2 nanoparticles resonators, fiber RL, and many more.Citation19–Citation25 Some recent works concerning Rh6G are showing further interest in RL especially in optimization of the acquired process in systems coupled with CdSe nanoparticles, showing enhanced RL process, as well as in Rh6G-doped silica gels pumped as one- and two-photon process.Citation26,Citation27 The other works are concerning Rhodamine-doped biologically derivative systems for enhance fluorescence observation.Citation28 Ease of handling, water and organic solvent solubility, high–quality factor are responsible for such popularity of Rh6G in the light amplification systems. Acquiring tunable lasing action is important for laser applications. Excitation of materials with tunable frequency laser beams is important for the studies based on properties possible to be utilizable only with intense light irradiation as it is with laser beams. Despite that the RL has found just few applications. Improvement in the controlling of this phenomenon by tuning emission wavelength can have significant impact on the future applications. Here, the authors show that using the dye properties and an adequate laser cavity design, it is possible to tune the emission wavelength of random lasers.
Experimental methods
Owing to the well-known Rh6G ability of aggregates formation polymeric layers containing variable Rhodamine concentration were prepared. In particular, a deoxyribonucleic acid (DNA)-based bio laser was demonstrated. Salmon tastes ds-DNA purchased from Sigma-Aldrich has been used in all experiments. Approximate size of used DNA was 1.3·106 Da 2000 bp, melting at the temperature 87.5°C. Deoxyribonucleic acid was stored in lyophilized form at 2°C. Deoxyribonucleic acid was functionalized with the cetyltrimethylammonium chloride surfactant (DNA-CTMA) following the modified Heckman's protocol.Citation29 If DNA alone is water soluble only, the DNA-CTMA complex can be solubilized in organic solvents. Lasing experiments were performed on thin films obtained by the drop casting technique with a constant DNA-CTMA concentration equal to 1% (w/w) to the butanol solution and varying Rh6G concentration equal to 0.5, 1.0 and 1.5% (w/w) according to the polymeric matrix weight. Thin layers of DNA-CTMA-Rh6G were dried in saturated vapor atmosphere of solvent at room temperature. Extended formation of polymeric layer resulted in good optical quality of the samples with measured by atomic force microscopy surface roughness in the range of 100 nm.
Samples based on starch biopolymer were prepared according to the procedure presented in our previous work describing the usage of starch in RL.Citation30 Starch was weighted and dispersed in higher temperature, above the gelatinization point. Then, an appropriate amount of Rhodamine was added to get the expected concentration. Samples were prepared from water suspensions and dried at room temperature too. The observed surface roughness was significantly higher than observed for DNA-CTMA matrices alone and was equal to about 200 nm. All prepared samples had comparable thickness designated of 15 μm.
Finally, the formation of Rh6G aggregates was also considered for water-based solutions. For that purpose, a sample containing Rh6G solubilized in water and the other one containing Rh6G-doped phosphatidylcholine (PC) liposomes dispersed in water were prepared. Liposomes are structures built of amphiphilic lipids forming a phospholipid bilayer and can be actively packed inside with dye or any other compound. Rhodamine 6G can be e.g. introduced into a liposomal formulation.Citation31 Lewis et al. have shown that Rh6G can act as an indicator in phospholipid-based polymeric biomaterials, for example, by coating biomedical equipment.Citation32 An increase of fluorescence intensity was observed from PC coated materials. It means that Rh6G is preferentially binding to the phosphorylcholine group like that in PC liposomes. Moreover, Liu et al. have reported that phospholipid films assisted Rh6G aggregates formation lead to both H- and J-types of aggregates formation.Citation33
In our case, the sample containing Rh6G only, was simply solubilized in water in the appropriate concentration equal to c = 10− 4 (w/w). The other PC liposomes containing samples were prepared according to the following procedure: PC lipids were weighted and dissolved in chloroform. After the solubilization, the samples were left in the ambient atmosphere to evaporate chloroform. On the surface of the glass vial, a PC lipid thin film was formed. Water solution of Rhodamine with weight concentration equal to c = 10− 4 (w/w) was added to the dried lipid film to gather weight concentration of PC equal to c = 10− 2 (w/w) and that of Rh6G equal c = 10− 4 (w/w). Then, the lipids containing sample was shaken to form multi-lamellar vesicles (MLV), a common procedure in liposomes formation. Applied procedure is typical for polydisperive liposomes acquiring of size ranging 0.2–20 μm. Exact size distribution was not measured, because only amphiphilic conditions wanted to be applied for Rh6G.Citation34 Both samples: Rh6G and Rh6G-doped PC liposomes were studied for ASE in 1 cm thick glass cuvette.
The ASE as well as the RL was studied on the optically pumped system based on the Nd:YAG pulsed nanosecond laser. To diminish the standard deviation of the energy pulses, crossed polarizer and half-wave plate were used for a precise energy manipulation. Amplified spontaneous emission was measured from the single spot of 3 mm diameter in function of energy density. The RL was induced with stripe-like shape beam formed by a beam expander and cylindrical lens of varying lengths from 2 up to 7 mm and width equal to 0.5 mm. The optical electric field was polarized perpendicular to the stripe longer axis. Amplified spontaneous emission as well as RL was measured with Shamrock SR-163 fiber spectrometer with 0.1 nm resolution. To decrease the statistical error in samples characterization, all measurements were repeated at least 10 times.
Results and discussion
ASE from Rh6G-doped DNA-CTMA layers
Amplified spontaneous emission gathered from the Rh6G-doped DNA-CTMA polymeric layer in function of Rhodamine concentration is presented in . The observed shift of the maximum intensity emission wavelength was of ca. 15 nm. It started from λ0.5% = 579.1 nm measured for the first sample of 0.5% (w/w) Rhodamine concentration up to λ1.5% = 593.5 nm for 1.5% (w/w). The shift direction was to the longer wavelengths with increasing concentration what is comparable with the Martinez et al. results of fluorescence emission shift because of the Rh6G aggregation.Citation15 The calculated energy threshold of ASE was found to be ca. ρASE ≈ 3.6 ± 0.4 mJ cm− 2. Aggregation process unless lack of control during sample preparation is responsible for the monotonic change in the emission wavelength. The aggregates population shifts between H-type, J-type and higher order aggregates, and the change in their distribution is responsible for the observed continuous shift of the emission spectrum.
Figure 1. Amplified spontaneous emission (ASE) spectrum for the Rhodamine 6G (Rh6G)-doped deoxyribonucleic acid was functionalized with the cetyltrimethylammonium chloride surfactant (DNA-CTMA) biopolymer layer. The Rh6G dye concentrations are: 0.5% (black), 1.0% (red), and 1.5% (green). The measured energy density threshold for ASE is equal to 3.6 ± 0.4 mJ cm− 2
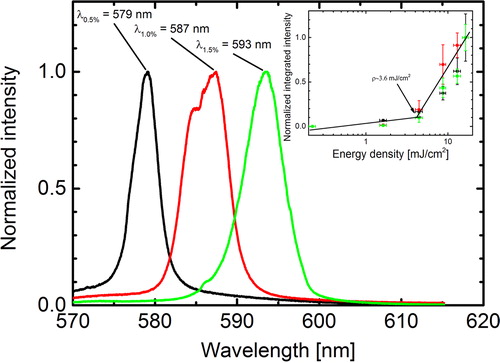
RL from Rh6G-doped DNA-CTMA layers
The previously observed tunable light amplification based on Rh6G aggregates in DNA-CTMA biopolymer motivated us to study the RL generation. To fulfil the RL requirements samples with a larger luminophore concentration, ranging from 1.0 up to 5.0% (w/w), Rh6G/DNA-CTMA were prepared. Quasi one-dimensional pumping system determined by pumping beam shape was responsible for the light propagation along the longer axis, perpendicular to the electric field polarization direction. The observed phenomenon was easily distinguished from the previously observed ASE. Lasing was observed as random numerous high intensity lasing modes appearance in the spectrum as shown in . The observed maximum emission wavelength was ranging from λ1.0% = 579.6 nm up to λ5.0% = 614.8 nm for the highest Rh6G concentration. It gives about Δλ = 35 nm RL emission tuning range. Different modes present in emission spectrum were easy to distinguish, being however close in frequency, what could be the result of surface roughness introducing multiple scattering to the system. Lasing threshold for all the samples was calculated basing on integrated lasing intensity in function of incident energy density. Calculated lasing threshold was found to be ρRL ≈ 6.5 mJ cm− 2.
Figure 2. Random lasing (RL) from Rhodamine 6G (Rh6G)-doped deoxyribonucleic acid was functionalized with the cetyltrimethylammonium chloride surfactant (DNA-CTMA) biopolymeric layer with increasing Rhodamine concentration 1.0% (red), 2.0% (green), and 5.0% (blue). Inset shows the calculated lasing energy density threshold equal to 6.5 mJ cm− 2
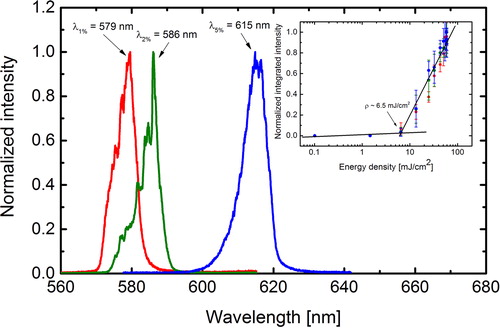
Another parameter describing the RL medium is the mean size of optical resonators responsible for generation of acquired lasing spectra. For that, the Fourier transform analysis of lasing spectra in k-vector scale was performed, allowing to determine the size of lasing cavity. From the calculated Fourier transform, the distance between repetitively occurring lasing modes was determined1 where D is the resonator diameter, m is the order of modes harmonics and n is the refractive index.
The mean Fourier transform of lasing spectrum above its threshold does not show specific pattern characteristic for repetitive lasing modes. The optical fiber spectrometer used in the experiment with resolution equal to δλ = 0.1 nm allows to determine the length of optical resonators with the lowest measurements limit equal to 10 μm. The Fourier transform analysis did not give a clear optical resonator size. Thus, most likely, the optical resonators originate from the surface roughness or smaller size aggregates.
Starch-based tunable RL
The obtaining of tunable RL action from Rhodamine aggregates was confirmed using different biological matrices. Recently, it was shown that starch can act as an biopolymeric matrix for RL action, with a significant increase of photostability comparing to the other biopolymeric matrices, what is caused by low oxygen permeability through starch layer.Citation27 Here, the authors show that also in this novel lasing matrix, the dye concentration plays an important role in tuning of the RL emission wavelength. For the sake of comparison, Rhodamine-doped starch layers were prepared, varying the dye concentration from 0.5 up to 2.0% (w/w). Similarly as with DNA-CTMA thin films, the RL was also observed. The starch-based films exhibit a higher surface roughness than DNA-CTMA, what could result in light localization on the surface roughness and generation of well-resolved lasing modes in contrary to the DNA-CTMA-based system. Surface roughness was induced by sol–gel transition during starch drying and structuring of starch granules with mean molecular mass of ∼105 Da. The maximum lasing emission was changing from 570 up to 605 nm when varying the Rh6G content from 0.5 to 2.0% (w/w), respectively. It gives a 35 nm laser wavelength tuning, as shown in . The width of emission profile in starch samples was found to be broader than observed in DNA-CTMA-based samples. In contrary to the results shown previously, changes in the emission profile widths were observed as well as changes in lasing thresholds for different Rhodamine concentration. The calculated lasing thresholds ρ were decreasing with increasing Rh6G concentration taking the following values: ρ0.5% = 5.5 mJ cm− 2; ρ1.0% = 4.5 mJ cm− 2;ρ2.0% = 3.5mJcm− 2. These results let us suppose that the aggregates type distribution is affecting the lasing threshold. A narrow light amplification profile, like observed in DNA-CTMA-based samples, is responsible for existence of single type aggregates. Highly dispersed samples, e.g. Rh6G-doped starch layers, exhibit a broader lasing emission profile.
Figure 3. Random lasing (RL) emission spectra from Rhodamine 6G (Rh6G):starch biopolymeric matrices for increasing amount of Rh6G. The measurements were performed with pumping energy equal to ρ = 7.5 mJ cm− 2. Inset shows the calculated lasing energy density thresholds for different dye concentrations, equal to: ρ0.5% = 5.5 mJ cm− 2; ρ1.0% = 4.5 mJ cm− 2 and ρ2.0% = 3.5 mJ cm− 2, respectively
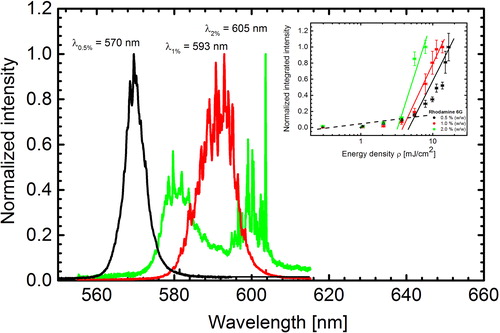
PC lipids assisted Rhodamine 6G aggregates formation
Rhodamine water solution and Rhodamine-doped PC liposomes water suspension were both tested for getting ASE. The main goal of this experiment was set to answer the question on light amplification possibility in saturated Rh6G solution without application of an external optical resonator. Solution containing only Rhodamine at concentration 10− 4 (w/w) with respect to water, excited with Nd:YAG pulsed laser exhibits only fluorescence with the maximum emission wavelength of ca. 565 nm, as seen in . The other sample containing PC multi-lamellar liposomes and Rh6G shows ASE at λmax = 583 nm wavelength. In the case of Rh6G-doped PC suspension, kept in 10 mm glass cuvette, ASE signal was observed with threshold of 10 mJ cm− 2 of energy density. In contrary, in Rh6G water solution, only a linear increase of fluorescence in function of energy density was seen. It appears that the presence of the lipids in the system is crucial for ASE taking place. The self-assembly of multi-lamellar liposomes from PC in water environment is a spontaneous process driven by hydrophobic interaction. Such stable in time structures are built from lipid bilayers embedded one in another one. Such systems are also polydispersive. Phosphatidylcholine dispersed in water solution forms multi-layer liposomes of random size, what can have positive effect on the stabilization of Rh6G aggregates because of a possible dye entrapment inside micelles and liposomes. However, Rhodamine is a water soluble dye and it is supposed that PC acts as a surfactant, stabilizing the structure. Assistance of PC lipids in Rh6G aggregation is driven by the hydrophobic interaction, which is reflected in dye properties like higher solubility of Rhodamine in organic solvents than in water.Citation28 Compartments formed in multi-lamellar liposomes are putting Rh6G molecules in closer proximity to each other. Lower molecules distance gives larger probability of stimulated and ASE, especially when dye is located at the lipid membrane interfaces.
Conclusions
In the present study, the authors have shown the possibility to obtain the tunable RL action exploiting the aggregation process present in used Rh6G luminophore. The biologically derived matrices, doped with Rh6G, exhibit ASE with its visible shift to the higher wavelengths. The observed shift is because of the presence of aggregates in the studied thin film and depends on their size understood as the dye concentration. The quasi one-dimensional optical pumping area forces the light propagation along the direction perpendicular to the polarization vector. Random lasing was observed on both the R6G-doped DNA-CTMA and starch-based systems, where feedback was acquired in the layer thickness, and multiple light scattering was generated with layer surface roughness and possible occurrence of Rh6G higher order aggregates. Lasing frequency tuning was clearly visible in both cases. However, composition of emitted laser modes was different. The authors suppose that it results from the surface roughness and spontaneously formed optical resonators. A confirmation of aggregates formation playing a crucial role in the light amplification process was done with water suspension containing PC lipids, doped with Rh6G luminophore. A shift of ASE with respect to the fluorescence emission spectrum was also observed. Such a shift is characteristic for an aggregation process.
Acknowledgments
This work was financially supported by the Polish National Science Centre (UMO-2013/11/N/ST4/01488) and Wroclaw University of Technology. The authors would like to acknowledge the Romanian Ministry of Education, Research, Youth and Sports support through the UEFISCDI organism, under Contract Number 3/2012, Code Project PN-II-PT-PCCA-2011-3.1-0316.
References
- Koizumi M. and Mataga N.: J. Am. Chem. Soc., 1953, 75, (2), 483–484.
- Crozet P. and Meyer Y.: C R Acad. Sci. B Phys., 1970, 271, (14), 718.
- Ketsle G. A., Levshin L. V., Slavnova T. D. and Chibisov A. K.: Dokl. Akad. Nauk. SSSR, 1971, 201, (1), 60.
- Pappalar R., Samelson H. and Lempicki A.: IEEE J. Quantum Electron., Qe, 1970, 6, (11), 716.
- Sagi S. R. and Rao G. G.: Fresenius’ Z. Anal. Chem., 1962, 188, (3), 164–168.
- Baranova E. G.: Opt. Spectrosc. (USSR), 1965, 18, (3), 230.
- Aristov A. V. and Maslyuko Y. S.: Opt. Spectrosc. (USSR), 1969, 27, (5), 468.
- Aristov A. V., Kozlovsk D. A. and Cherkaso A. S.: Opt. Spectrosc. USSR, 1970, 29, (2), 221.
- Yamashit M. and Kashiwag H.: J. Chem. Phys., 1973, 59, (4), 2156–2157.
- Schafer F. P. and Ringwels L.: Z. Naturforsch. A, A, 1973, 28, (5), 792–793.
- Yamashit M. and Kashiwag H.: J. Phys. Chem. US, 1974, 78, (20), 2006–2009.
- Galanin M. D. and Chizhiko E. A.: Izv. An SSSR Fiz., 1972, 36, (5), 941.
- Chis I. D., Julea T. N. and Popescu I. M.: Rev. Roum. Phys., 1974, 19, (9), 1005.
- Ferguson A. I., Dunn M. H. and Maitland A.: Opt. Commun., 1976, 19, (1), 10–13.
- Martinez V. M., Arbeloa F. L., Prieto J. B. and Arbeloa I. L.: J. Phys. Chem. B, 2005, 109, (15), 7443–7450.
- Toptygin D., Packard B. Z. and Brand L.: Chem. Phys. Lett., 1997, 277, (5–6), 430–435.
- Lofaj M., Valent I. and Bujdak J.: Cent. Eur. J. Chem., 2013, 11, (10), 1606–1619.
- Lewkowicz A., Bojarski P., Synak A., Grobelna B., Akopova I., Gryczynski I. and Kulak L.: J. Phys. Chem. C, 2012, 116, (22), 12304–12311.
- Tikhonov E., Yashchuk V. P., Prygodjuk O. and Bezrodny V.: Sol. St. Phen., 2004, 99–100, 77–82.
- Shin H. W., Cho S. Y., Choi K. H., Oh S. L. and Kim Y. R.: Appl. Phys. Lett., 2006, 88, (26)
- de Matos C. J. S., Menezes L. D. S., Brito-Silva A. M., Gamez M. A. M., Gomes A. S. L. and De Araujo C. B.: Phys. Rev. Lett., 2007, 99, (15)
- Garcia-Revilla S., Sola I., Balda R., Roso L., Levy D., Zayat M. and Fernandez J.: Opt. Compon. Mater. VII, 2010, 7598.
- Fernandez J., Garcia-Revilla S. and Balda R.: NATO Sci. Peace Sec. B, 2011, 321–342.
- Cerdan L., Costela A. and Garcia-Moreno I.: Org. Electron., 2012, 13, (8), 1463–1469.
- Yang L. L., Feng G. Y., Yao K., Yi J. Y., Luo W. Q. and Zhou S. H.: Integr. Ferroelectr., 2012, 138, 9–15.
- Augustine A. K., Radhakrishnan P., Nampoori V. P. N. and Kailasnath M.: Laser Phys. Lett., 2015, 12, 2.
- Garcia-Ramiro B., Illarramendi M. A., Garcia-Revilla S., Balda R., Levy D., Zayat M. and Fernandez J.: Appl. Phys. B, 2014, 117, 4.
- Boni M., Nastasa V., Andrei1 I. R., Staicu A. and Pascu M. L.: Biomicrofluidics, 2015, 9, 014126.
- Heckman E. M., Hagen J. A., Yaney P. P., Grote J. G. and Hopkins F. K.: Appl. Phys. Lett., 2005, 87, 21.
- Cyprych K., Sznitko L. and Mysliwiec J.: Org. Electron., 2014, 15, (10), 2218–2222.
- Rezler E. M., Khan D. R., Lauer-Fields J., Cudic M., Baronas-Lowell D. and Fields G. B.: J. Am. Chem. Soc., 2007, 129, (16), 4961–4972.
- Wang J. H., Bartlett J. D., Dunn A. C., Small S., Willis S. L., Driver M. J. and Lewis A. L.: J. Microsc. Oxford, 2005, 217, 216–224.
- Liu T. T., Yu A. C., Luo G. B., Zhao X. S., Ying L. M., Huang Y. Y. and Huang C. H.: Acta Phys. Chim. Sin., 2000, 16, (1), 49–54.
- Cohen B. E. and Bangham A. D.: Nature, 236, 173–174.