Abstract
In this work, the authors performed X-ray imaging of mice using a colloid solution of Au nanoparticles that are coated with silica and subsequently surface-modified with poly(ethylene glycol) (PEG) (Au/SiO2/PEG). The silica-coating for Au nanoparticles and the amination for silica-coated particles were simultaneously performed in the presence of the Au nanoparticles, which were prepared by reducing Au ions (III) with sodium citrate in water at 80°C and by surface-modifying the Au nanoparticles with (3-aminopropyl)-trimethoxysilane (APMS), by a sol–gel process using tetraethylorthosilicate (TEOS), (3-aminopropyl)-triethoxysilane, water and sodium hydroxide (Au/SiO2-NH2). The surface modification of Au/SiO2-NH2 particles with PEG was performed by simply adding PEG with a functional group that reacts with an amino group to the Au/SiO2-NH2 particle colloid solution. A computed tomography value of the Au/SiO2/PEG colloid solution with an Au concentration of 0.129M was as high as 895 ± 8 HU. Mouse tissues were imaged following injection of the Au/SiO2/PEG particle colloid solution.
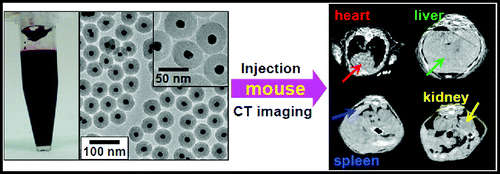
Introduction
Imaging techniques have become indispensable to medical diagnosis. An imaging technique using X-ray is one of the representative techniques for medical diagnosis.Citation1–Citation3 Contrast agents are useful to obtain clear X-ray images.Citation3 Solutions with dissolved iodine complexes are typical as commercially available X-ray contrast agents. Although they work well in imaging and have been used for many years, they encounter some problems. One problem is adverse events such as allergic reactions in patients who are provoked by iodine compounds.Citation4–Citation6 Thus, they cannot be used for such patients. The other problem is short residence time in living bodies, which has been noted by some researchers.Citation7,Citation8 Because iodine compounds are in the molecular size range, they are involved with body fluid flow in living bodies. As a result, they move fast and cannot stay in the living body for a long period of time, which implies that it is difficult to obtain clear and steady X-ray images using commercial X-ray contrast agents. To solve the problem of short residence time, contrast chemicals that contain or are composed of nanometre-size particle may be effective because the viscous resistance of a fluid acts on the particles and controls their flow.
In addition to iodine compounds, Au also increases the contrast of X-ray images because it can strongly absorb X-ray. From the viewpoints of absorption ability and particle formation, metallic Au nanoparticles have been examined towards X-ray contrast agents to image tissues in living bodies at the nanometre level.Citation9–Citation11 Metallic nanoparticles also have a problem regarding their toxicity.Citation12–Citation14 A promising method to solve the toxicity problem is to coat Au nanoparticles with inert materials for living bodies, i.e. to form particles with a core–shell structure, which is composed of a Au-particle core and an inert-material shell for living bodies. The shell eliminates the contact between the Au particles and the living bodies, which will reduce the toxicity of the Au particles. Various coating techniques of Au particles with silica, which is inert for living bodies, have been proposed in previous decades and recent years.Citation15,Citation16 Most techniques are based on a process of preparing Au nanoparticles, surface-modifying the Au nanoparticles to increase its affinity of the Au surface and silica source such as alkoxide groups, and producing silica shells through a sol–gel reaction of silicon alkoxide. Our research group has also developed a method to fabricate silica-coated Au nanoparticles (Au/SiO2).Citation17–Citation20
The living bodies recognise hydrophobic materials as foreign, which spoils their blood circulation or residence in the living bodies. PEGylation, which is an immobilisation process of the poly(ethylene glycol) (PEG) material, is often performed to make the surface hydrophilic.Citation21–Citation23 In our previous workCitation20, the Au/SiO2 particles were PEGylated for the same purpose. Although silica surface is hydrophilic among various solid materials, the PEGylation for the Au/SiO2 particles was performed to make their surface more hydrophilic. As a result, the PEGylation of Au/SiO2 particle surface had an effect of preventing the particles from being recognised as alien substances, which provided an increase in residence time of particles in the mouse. This result indicated that the PEGylation for the Au/SiO2 particles was effective to improve their performance in living bodies. A reaction between an amino group and the pyrrolidinyl groups of poly(oxy-1,2-ethanediyl),α-methyl-ω-[2-[(2,5-dioxo-1-pyrrolidinyl)oxy]-6-oxohexyloxy] (ME-050HS), which is a type of PEG, was used for the PEGylation. Before PEGylation, amination, which is a modification with amino groups, was performed on the silica surface of the Au/SiO2 particles for effective PEGylation: the amino groups were introduced on the surface by adding alkoxysilane with an aminoalkyl group to the Au/SiO2 particle colloid solution. Our method absolutely required this amination process for the PEGylation, which lengthened the procedures. In addition, because both amounts of amino groups and PEG molecules were not larger than expected, the method can be improved.
The present work proposes an alternative method to produce PEGylated Au/SiO2 (Au/SiO2/PEG) particles. The formation of silica shell and surface amination were performed almost simultaneously, which reduced the preparation time of the Au/SiO2/PEG particle colloid solution. The X-ray imaging ability of PEGylated Au/SiO2 (Au/SiO2/PEG) particles was also investigated in the present work: their X-ray absorption was measured, and the tissues of a mouse, into which the colloid solution of Au/SiO2/PEG nanoparticles was injected, were imaged.
Experimental
Materials
Hydrogen tetrachloroaurate (III) trihydrate (HAuCl4·3H2O) (Kanto Chemical, 98%) and trisodium citrate dihydrate (Na-cit) (Kanto Chemical, 99%) were used as an Au source and a reducing reagent to fabricate Au nanoparticles, respectively. The silane coupling agent to increase the affinity between the Au particle surface and the silica shell was (3-aminopropyl)trimethoxysilane (APMS) (Sigma-Aldrich, 97%). In silica coating, tetraethylorthosilicate (TEOS) (Kanto Chemical, 95%), NaOH aqueous solution (Kanto Chemical, 1M), (3-aminopropyl)triethoxysilane (APES) (Sigma-Aldrich, 98%) and ethanol (Kanto Chemical, 99.5%) were used as a silica source, a silica source with amino group, a catalyst for the sol–gel reaction of TEOS and a solvent, respectively. ME-050HS (NOF, Mw 55 000) was used to PEGylate the particle surface. All chemicals were used as received. Water was ion-exchanged and distilled using the Yamato WG-250 to use in all preparation.
Preparation
A colloid solution of Au nanoparticles was prepared by adding an Na-cit aqueous solution to an HAuCl4 aqueous solution under vigorous stirring at 80°C. The initial concentrations of Au and Na-cit were 2.4 × 10− 4 and 1.6 × 10− 3M, respectively. According to our previous work, a peak that was attributed to the surface plasmon resonance of Au nanoparticles was detected at 520.5 nm, the Au nanoparticles had an average size of 17.9 ± 1.3 nm. The Au nanoparticles were silica-coated using a sol–gel method as follows. To H2O/ethanol, the obtained Au nanoparticle colloid solution and an APMS/ethanol solution were sequentially added. After 15 min, a TEOS/ethanol solution was added to the colloid solution. After another 15 min, an NaOH aqueous solution was rapidly injected into the Au/TEOS/H2O/ethanol colloid solution to initiate the silica coating of Au particles. An APES/ethanol solution was added to the mixture to aminate Au/SiO2 particles (as-prepared Au/SiO2-NH2) 15 min after the initiation. The reaction temperature and time were 35°C and 24 h, respectively. The initial concentrations of Au, APMS, TEOS, H2O, NaOH and APES in the final reactant solution were adjusted to 4.3 × 10− 5, 2 × 10− 5, 5.0 × 10− 4, 10.7, 5.0 × 10− 4, 5 × 10− 5 − 2.5 × 10− 4 and M, respectively. The as-prepared Au/SiO2-NH2 particles were washed and concentrated by repeating the following process: centrifuge (18 000 rev min− 1, 30 min), removing the supernatant with decantation, adding and shaking with a vortex mixer three times (concentrated Au/SiO2-NH2 particles). The added solvents were ethanol for the first process and water for the second and third processes. To produce Au/SiO2/PEG particles, an ME-050HS aqueous solution was added to the concentrated Au/SiO2-NH2 particle colloid solution. An initial ME-050HS concentration was 1.0 × 10− 3M. The Au/SiO2/PEG particle colloid solution had an Au concentration of 0.129M, which assumed that the entire HAuCl4 was reduced to form Au nanoparticles and that no particle was lost during the washing process.
Characterization
The samples were characterised using visible (VIS) spectroscopy, transmittance electron microscopy (TEM), and electrophoretic light scattering (ELS). The VIS extinction of the particle colloid solution was measured using a Shimadzu UV-3101PC spectrophotometer. The TEM was performed using a JEOL JEM-2100 microscope that operated at 200 kV. The TEM samples were prepared by dropping and evaporating the nanoparticle suspensions on a colloid-coated copper grid. Dozens of particle diameters were measured to determine the volume averaged particle size. The zeta-potentials of the particles were measured using ELS to obtain information on the particle state. Electrophoretic light scattering was performed using a Brookhaven instrument Zeta-Plus. An HCl or NaOH aqueous solution was added to the solution to vary the solution pH for the ELS measurement.
CT images and CT values of the samples such as the Au/SiO2/PEG particle colloid solution and the mouse that was injected with the particle colloid solution were obtained using an Aloka La theta LCT-200 CT system, which was also used in our previous works.Citation19,Citation20 The samples were placed in a tube with a diameter of 3.7 cm and a length of 29.5 cm. The mouse was put under anaesthesia, and the colloid solution was injected into the mouse from its tail veins. The used mouse was an imprinting control region mouse with age 5–6 weeks. The images were taken as if the samples were cut into round slices. The CT values were estimated based on the CT values of − 1000 and 0 HU for air and water, respectively.
Results and discussion
Effect of the APES concentration on fabrication of Au/SiO2-NH2 particles
During the fabrication of Au/SiO2-NH2 particles, the APES was expected to react with OH groups on as-fabricated Au/SiO2 particles, as indicated in following schemes, which introduced amino groups on particle surface.1
2 shows photographs of Au/SiO2-NH2 particle colloid solutions to prepare at various APES concentrations. The colloid solutions were purple because of the SPR absorption of Au nanoparticles. No sediments were produced, which indicated that the Au/SiO2-NH2 particles were colloidally stable during the silica coating.
Figure 1. Photographs of Au/SiO2-NH2 particle colloid solutions that were prepared with APES concentrations of a 5 × 10− 5, b 1 × 10− 4, c 1.5 × 10− 4, d 2 × 10− 4 and e 2.5 × 10− 4M
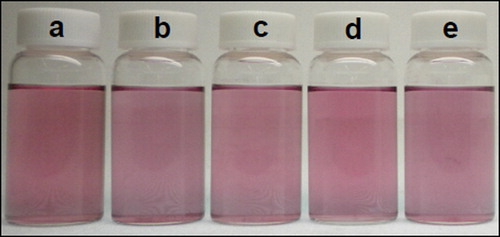
shows the UV-VIS extinction spectra of Au/SiO2-NH2 particle colloid solutions that were prepared with various APES concentrations. The band positions of the surface plasmon resonance were observed in a range of 532–539 nm appeared for all examined APES concentrations. All plasmon bands were red-shifted with respect to the uncoated Au nanoparticles (520.5 nm). According to a study on the optical properties of core–shell particles, the red shift of surface plasmon absorption occurs because of a local increase in refractive index around the Au particles when the silica shell forms. A similar mechanism was considered in the present work, which implied that the Au nanoparticles could be silica coated using the present method.
Figure 2. UV-VIS extinction spectra of Au/SiO2-NH2 particle colloid solutions, which were prepared with various APES concentrations. The black, red, blue, green and pink spectra correspond to samples a–e in Fig. 1, respectively
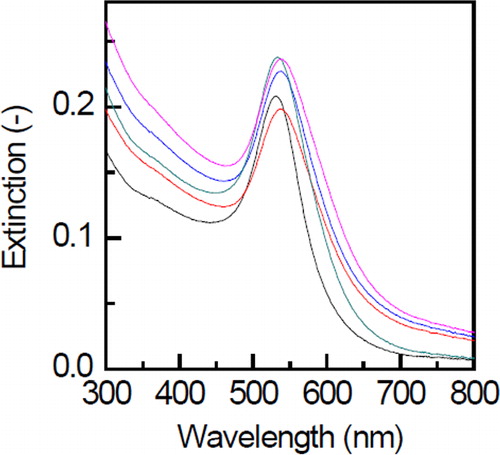
shows the transmittance electron microscopy (TEM) images of Au/SiO2-NH2 particles prepared at various APES concentrations. At two low APES concentrations of 5 × 10− 5 and 1 × 10− 4M, silica particles that contain Au nanoparticles as the cores appeared to aggregate through the gel network structure. These low APES concentrations provided the low ionic strength of the solution, where the double-layer repulsion between the Au/SiO2 particles and the silica nuclei was probably large. Consequently, most silica nuclei that were generated from APES were consumed to form a gel instead of a shell. A quasi-perfect core–shell structure was produced with no formation of gel network structure at 1.5 × 10− 4M. When the concentration increased to 2 × 10− 4 and 2.5 × 10− 4M, the Au nanoparticles were coated with silica. However, some particles contained multiple Au nanoparticles. The ionic strength of the colloid solution increased with increasing APES concentration, which decreased the thickness of the double layer on the particle surface. The decrease in thickness promoted the aggregation of Au/SiO2 particles. Then, a silica shell formed on the aggregate surface. As a result, the Au/SiO2-NH2 particles contained multiple Au cores.
Figure 3. Transmittance electron microscopy (TEM) images of Au/SiO2-NH2 particles. Insets are high magnification images. The samples were prepared at various APES concentrations, which were identical to those in Fig. 1
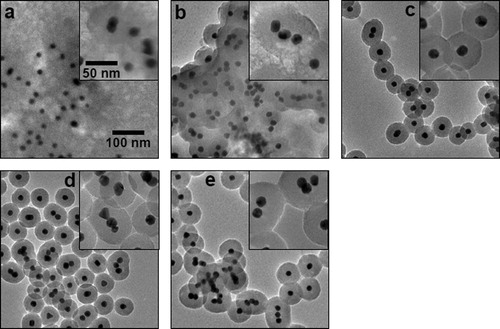
shows the zeta-potentials of Au/SiO2-NH2 particles that were prepared at various APES concentrations as a function of the pH of their solutions. In all examined particles, with increasing pH, the zeta-potentials with positive values decreased, passed through isoelectric points (IEP) to reveal negative values, and decreased further. The IEPs were 8.3, 9.8, 10.1, 9.6 and 10.6 for the AEPS concentrations of 5 × 10− 5, 1 × 10− 4, 1.5 × 10− 4, 2 × 10− 4 and 2.5 × 10− 4M, respectively. According to our previous work,Citation24 Au/SiO2 particles with no amino groups or particles that were prepared at the APES concentration of 0M had an IEP of 7.3. Accordingly, the IEP tended to increase with the increase in APES concentration in the range of 0–2.5 × 10− 4M. All examined Au/SiO2-NH2 particles had higher IEPs than Au/SiO2 particles. These higher IEPs were probably derived from amino groups in the silica shell because amino groups in many types of amines such as ammonia, alkylamine, and dialkylamine have acid dissociation constants of 9–10. Accordingly, these results supported that the amino groups were successfully introduced to the silica shell, and it was found that the amount of introduced amino groups increased with the increase in APES concentration.
Au/SiO2/PEG particles
During the PEGylation, the ME-050HS was expected to react with NH2 groups on the Au/SiO2-NH2 particles, as indicated in following schemes, which introduced the PEG on particle surface.3
4 shows a photograph of the Au/SiO2/PEG particle colloid solution, which was dark red. Although the particle colloid solution was concentrated to 1/2000 of the volume of the as-prepared Au nanoparticle colloid solution during the preparation, it was confirmed with naked eyes that the dark-red colloid solution contained no sediments, which indicated that the Au/SiO2/PEG particle colloid solution was colloidally stable at micrometre–meter order even at high concentration. However, characterisations such as dynamic light scattering, analysis based on Coulter principle and sedimentation velocity experiment using ultracentrifugation are required to conclude their colloidal stability in aqueous solution at nanometer order.
Figure 5. Images of Au/SiO2/PEG particles. Images a–c are photographs of their colloid solution, transmittance electron microscopy (TEM) image, and a CT image of their colloid solution, respectively
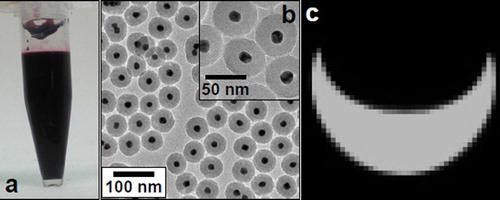
shows a transmittance electron microscopy (TEM) image of Au/SiO2/PEG particles. Most particles contained a single core of the Au nanoparticles. The Au/SiO2/PEG particles were fabricated by PEGylating the Au/SiO2-NH2 particles in , and the Au/SiO2/PEG particles appeared to have similar morphology to the Au/SiO2-NH2 particles. Accordingly, this observation indicated that the core–shell structures of particles were not damaged even with the PEGylation. Its particle size was 56.6 ± 2.9 nm.
X-ray imaging
shows a CT image of the Au/SiO2/PEG particle colloid solution. The image was clear with light contrast, and its CT value was as high as 895 ± 8 HU, which was converted with respect to the molar concentration of subject materials such as Au and I (converted CT value) to compare with the CT value of the commercial contrast agent: The converted CT value with respect to the Au concentration (0.129M) was 6.94 × 103 HU/M. According to our previous work, for Iopamiron 300, its converted CT value with respect to the iodine concentration is 4.76 × 103 HU M− 1.Citation20 The AgI/SiO2/PEG particle colloid solution had a larger converted CT value than Iopamiron 300. Similar to the present work, several previous works reported that Au nanoparticles or clusters had larger converted CT values than commercial iodine compound contrast agents.Citation25–Citation27 Because gold absorbs X-rays more strongly than iodine per atom because of its large atomic number, the Au/SiO2/PEG particle colloid solution had a large converted CT value. Accordingly, it was confirmed that the Au/SiO2/PEG particle colloid solution could function as an X-ray contrast agent for sensitivity.
shows X-ray images of the mouse before and after it was injected with the Au/SiO2/PEG particle colloid solution. Since it was difficult to clearly distinguish between contrasts in images of tissues before and after the injection, the CT values that were measured by the CT system were used for discussion. shows the CT values of various tissues as a function of time after the injection, where a value at 0 min indicated that it was before the injection. In contrast to the images, differences in the CT values were confirmed clearly between before and after the injection. For a kidney, the contrast was not remarkably changed, and its CT value was almost constant at approximately 50 HU. After the injection, the contrasts of the liver and the spleen appeared to be slightly lighter than before the injection. Their CT values, which were 89 and 68 HU before the injection, respectively, reached 112 and 121 HU at 720 min after the injection, respectively. In our previous study on the Au/SiO2/PEG particle colloid solution,Citation20 the CT values of the liver and the spleen tended to increase with an increase in time because the Au/SiO2/PEG particles were trapped and accumulated in the liver and the spleen. A similar tendency was obtained in the present work. For the heart, the injection also provided no remarkable change in contrast, which indicated that the trapping and accumulation of Au/SiO2/PEG particles occurred, and the particles were not circulated in the mouse. According to our previous work,Citation20 the circulation of Au/SiO2/PEG particles in a mouse was confirmed for Au/SiO2/PEG particles that were fabricated by separately performing two steps of production and surface-amination of Au/SiO2 particles. The present work simultaneously performed the production and surface-amination of Au/SiO2 particles to simplify the fabrication process. This difference probably resulted in the small amount of amino groups on the particles, which led to an incomplete PEGylation. As a result, the effect of PEGylation on the circulation did not appear, although the reason remains unclear.
Conclusions
Au/SiO2-NH2 particles that contain 17.9-nm Au cores were fabricated by simultaneously silica coating Au nanoparticles and introducing amino groups to the silica-coated particles during the silica coating. The colloid solution of 56.6 nm Au/SiO2/PEG particles was produced by making ME-050HS react with the amino groups that were introduced to the silica-coated particles. The Au/SiO2/PEG particle colloid solutions could absorb X-rays better than the commercial X-ray contrast agent: the Au/SiO2/PEG particle colloid solution had a higher CT value (with respect to the Au concentration of 6.94 × 103 HU M− 1) than the commercial X-ray contrast agent. The colloid solution injection into the mouse lightened the contrasts of its internal organs, i.e., increased their CT values. Accordingly, the Au/SiO2/PEG particle colloid solution was applicable as an X-ray contrast agent. Because this paper focused on the time dependence of the CT values of various tissues in the mouse, other subjects, such as the toxicity of particle colloid solutions and the precise mechanisms of amination and PEGylation, were not investigated. Further studies on these subjects are required as the next step towards practical use.
Conflicts of interest
The authors declare no conflicts of interest.
Acknowledgements
Authors express our thanks to Prof. T. Noguchi at the College of Science of Ibaraki University, Japan (current affiliation: Faculty of Arts and Science of Kyushu University, Japan) for his help with the TEM observation. This work was supported by a Grant-in-Aid for Scientific Research on Innovative Areas ‘Nanomedicine Molecular Science’ (No. 2306) from the Ministry of Education, Culture, Sports, Science, and Technology of Japan and by JSPS KAKENHI Grant Number 24310085.
References
- Tsuji K., Nakano K., Hayashi H., Hayashi K. and Ro C.-U.: Anal. Chem., 2008, 80, 4421–4454.
- Liu Y., Al K. and Lu L.: Accounts Chem. Res., 2012, 45, 1817–1827.
- Lusic H. and Grinstaff M. W.: Chem. Rev., 2013, 113, 1641–1666.
- Thomsen H. S.: Eur. J. Radiol., 2011, 80, 77–82.
- Ichikawa T., Motosugi U., Morisaka H., Sou H., Onohara K., Sano K. and Araki T.: Eur. J. Radiol., 2012, 81, 2450–2455.
- Kim K. H., Kim Y. S., Kuh S. U., Park H. S., Park J. Y., Chin D. K., Kim K. S. and Cho Y. E.: Spine J, 2013, 13, 564–571.
- Hallouard F., Briancon S., Anton N., Li X., Vandamme T. and Fessi H.: Eur. J. Pharm. Biopharm, 2013, 83, 54–62.
- Felix Q. Y., Yap Y., Yin L., Ma L., Zhou Q., Dobrucki L. W., Fan T. M., Gaba R. C. and Cheng J.: J. Am. Chem. Soc, 2013, 135, 13620–13623.
- Ghann W. E., Aras O., Fleiter T. and Daniel M.-C.: Langmuir, 2012, 28, 10398–10408.
- Mieszawska A. J., Mulder W. J. M., Fayad Z. A. and Cormode D. P.: Mol. Pharmaceut, 2013, 10, 831–847.
- Ross R. D., Cole L. E., Tilley J. M. R. and Roeder R. K.: Chem. Mater, 2014, 26, 1187–1194.
- Lasagna-Reeves C., Gonzalez-Romero D., Barria M. A., Olmedo I., Clos A., Ramanujam V. M. S., Urayama A., Vergara L., Kogan M. J. and Soto C.: Biochem. Biophy. Res. Commun, 2010, 393, 649–655.
- Park M. V. D. Z., Neigh A. M., Vermeulen J. P., de la Fonteyne L. J. J., Verharen H. W., Briedé J. J., van Loveren H. and de Jong W. H.: Biomater, 2011, 32, 9810–9817.
- Wang Y. and Li X.: Procedia Eng., 2012, 45, 1004–1009.
- Schulzendorf M., Cavelius C., Born P., Murray E. and Kraus T.: Langmuir, 2011, 27, 727–732.
- Torimoto T., Horibe H., Kameyama T., Okazaki K., Ikeda S., Matsumura M., Ishikawa A. and Ishihara H.: J. Phys. Chem. Lett., 2011, 2, 2057–2062.
- Kobayashi Y., Inose H., Nakagawa T., Gonda K., Takeda M., Ohuchi N. and Kasuya A.: J. Colloid Interface Sci., 2011, 358, 329–333.
- Kobayashi Y., Inose H., Nakagawa T., Gonda K., Takeda M., Ohuchi N. and Kasuya A.: Surf. Eng., 2012, 28, 129–133.
- Kobayashi Y., Inose H., Nakagawa T., Kubota Y., Gonda K. and Ohuchi N.: J. Nanostruct. Chem., 2013, 3, 62.
- Kobayashi Y., Inose H., Nagasu R., Nakagawa T., Kubota Y., Gonda K. and Ohuchi N.: Mater. Res. Innov., 2013, 17, 507–514.
- Qian W., Murakami M., Ichikawa Y. and Che Y.: J. Phys. Chem C, 2011, 115, 23293–23298.
- Howard M. D., Lu X., Rinehart J. J., Jay M. and Dziubla T. D.: Langmuir, 2012, 28, 12030–12037.
- Wilms V. S., Bauer H., Tonhauser C., Schilmann A.-M., Müller M.-C., Tremel W. and Frey H.: Biomacromolecules, 2013, 14, 193–199.
- Kobayashi Y., Nagasu R., Nakagawa T., Kubota Y., Gonda K. and Ohuchi N.: J Nanoparticle Res., 2014, 16, 2014–2551.
- Park J. A., Kim H., Kim J. H., Jeong S. W., Jung J. C., Lee G. H., Lee J., Chang Y. and Kim T. J.: Bioorg. Med. Chem. Lett., 2010, 20, 2287–2291.
- Peng C., Zheng L., Chen Q., Shen M., Guo R., Wang H., Cao X., Zhang G. and Shi X.: Biomater, 2012, 33, 1107–1119.
- Zhang A., Tu Y., Qin S., Li Y., Zhou J., Chen N., Lu Q. and Zhang B.: J. Colloid Interface Sci., 2012, 372, 239–244.