Abstract
Zinc oxide (ZnO)–polycarbonate (PC) nanocomposite films were prepared by blade coating method by using pre-synthesized spherical ZnO nanoparticles of 15–20 nm size and pre-dissolved polycarbonate. The blend was homogenized by sonication and the films were dried in an oven at moderate temperature. Various films with different % loading of ZnO nanoparticles in PC were prepared and their effect on antibacterial properties was studied. It is observed that increasing wt-% loading of ZnO nanoparticles in PC leads to enhanced antibacterial properties against Staphylococcus aureus (S. aureus) and Escherichia coli (E. coli). The freshly prepared ZnO nanoparticles were thoroughly characterized by XRD, SEM, UV-visible and photoluminescence spectroscopy before their use in preparing nanocomposite films. Contact angle measurement of the films was studied to ascertain their hydrophobic behavior. Such nanocomposite films have potential in various packaging applications because of their effective antibacterial and UV-blocking properties and hydrophobic nature.
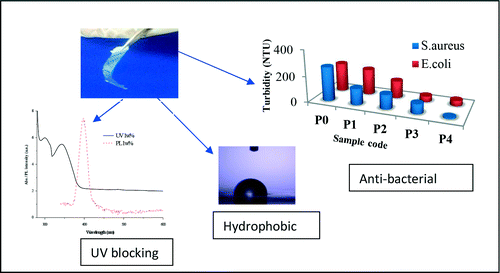
Introduction
Most of the recent antibacterial agents are chemically assembled natural compounds.Citation1 In general, the antibacterial agents could be classified as either bactericidal (kill bacteria) or bacteriostatic (quenching bacterial growth). Antibacterial activity of a compound is governed by locally killing bacteria or slows down their growth, without being in general toxic to surrounding tissue. The major drawbacks for conventional antimicrobial agents are the development of multiple drug resistance and also adverse side effects. Drug resistance enforces high-dose administration of antibiotics, often generating intolerable toxicity, which has prompted the development of alternative strategies to treat bacterial diseases.Citation2 Nanotechnology has provided a new dimension in the area of antibacterial activity. In particular, the ability to prepare highly ordered nanoparticles of any size and shape have led to the development of new biocidal/antimicrobial agents. The use of various nanoparticles (NPs) as an effective antibacterial agent is highlighted in various reportsCitation3–Citation10 along with. Especially, different antimicrobial NPs have proven their effectiveness for treating infectious diseases, including antibiotic-resistant ones, in vitro as well as in animals.Citation11
Bio-nanotechnology is the integration between biotechnology and nanotechnology for developing biosynthetic and environmental friendly technology for the synthesis of nanomaterial.Citation12 Zinc oxide (ZnO) is non-toxic, II–VI semiconductor material with reportedly good antibacterial activity. Owing to noble properties such as high refractive index, high thermal conductivity, binding energy, antibacterial and UV-protection of ZnO, it could be used in various materials and products, including medicine, cosmetics, varistors, solar cells, rubber and concrete, and foods.Citation13 It is an inorganic white powder, which is insoluble in water. Zinc oxide nanoparticles have been shown to be useful antibacterial and antifungal agents when used as a surface coating on materials and can be a very useful material for bio-nanotechnology. The antimicrobial activity of ZnO nanoparticles is well known as the larger surface area of nanoparticles can result in a greater degree of interaction with bacterial cell walls. Among inorganic materials, metal and metal oxides have elicited a great deal of interest for use as antimicrobial agents because of their durability, selectivity and resistant to heat. One of the primary reasons these inorganic materials (metal and metal oxide like ZnO) have attracted so much attention is because of their ability to withstand intensive processing conditions.Citation14,Citation15 They have selective toxicity to bacteria but have minimal effect on human cells.Citation16 Zinc oxide belongs to a group of metal oxides with photo-oxidizing and photo-catalytic ability against chemical and biological species.Citation17,Citation18 Zinc is an essential element and ZnO nanoparticles are considered to be non-toxic and toxicity studies have shown that zinc ion do not cause any damage to the DNA of human cells.Citation19 The antibacterial activity of ZnO nanoparticles is because of their electrostatic interaction with the cell surface.Citation20
Protective packaging is one of the most important requirement of modern society as most human being depend on readily available packaged food (raw or precooked) to ease out their workload at home after having spent demanding work hours thus food industry success depends upon the quality of packaging employed to wrap the food and food ingredients. Antibacterial films can protect the food surfaces because of presence of ZnO nanoparticles and therefore the shelf life of the packaged food enhances at the same time keeping the product healthy. Lately, chitosan and pullulan based films and coatings have shown great potential for food packaging because of their antimicrobial ability.Citation21,Citation22 Arora and Padua have studied starch/ZnO–carboxymethylcellulose sodium nanocomposite for their promising applications in food packaging industry.Citation23 Similarly, research group of Haciu et al. have studied ZnO with agar-based polymer films for food packaging application.Citation24
However, polymer-based nanocomposite offers the possibility to contain diverse properties that are impossible within a single material. Polycarbonate is an amorphous engineering thermoplastic with good processibility, which can be recycled. Thus, ZnO–PC nanocomposite could be a potential candidate for various applications including food packaging. It was reported that 0.5% ZnO nanoparticles in polycarbonate shows higher load-carrying capacity than polycarbonate alone, reducing both the wear rate and the friction coefficient with respect to polycarbonate.Citation25 Nanotechnology has opened up several avenues for ZnO-based nanocomposites for modern day applications such as packaging technology. However, one of the major issue that remains is the synthesis and thorough analysis before testing any application. In this regard, choice of method for preparation of ZnO nanoparticles has become crucial, e.g. if one needs to use the ZnO nanoparticles for organic purposes then the synthesis has to be performed accordingly, to create hydrophobic surfaces. However, for its use in biological systems and for other aqueous medium based applications, the surfaces need to be hydrophilic in nature. To impart hydrophobic coating by use of ZnO–PC nanocomposite might be a lucrative area, which will offer great commercial potential.
Thus, the authors have adopted a method to synthesize ZnO nanoparticles with PVP as capping agent to obtain spherical shaped below 20 nm sized particles. The nanocomposite based on ZnO nanoparticles and polycarbonate has been scarcely documented previously, despite polycarbonate being a useful material for food industry. In this article, the authors describe a simple one-step method for preparation of a nanocomposite of polycarbonate with ZnO and highlight the antibacterial properties of so-prepared composite films against gram-positive and gram-negative bacteria. The present study more precisely was carried out with an objective to analyze the antimicrobial properties of ZnO–PC nanocomposite against some common food borne pathogens such as Staphylococcus aureus (S. aureus) and Escherichia coli (E. coli).
Experimental and characterization
All chemicals were purchased commercially and were used as received. Zinc nitrate hexahydrate, Zn(NO3)2, 6H2O (99%) was purchased from Thomas Baker, India. Polyvinylpyrrolidone, PVP (M.W 40 000) was purchased from Loba Chemicals, India. Polycarbonate (Lexan™) was procured from Sabic Industries. The absorption spectra of the as-prepared ZnO and PC–ZnO were measured by same technique. Photoluminescence (PL) of various stages of reaction as well as of final products was recorded using Eclipse fluorescence spectrophotometer (Agilent Technologies, Santa Clara, CA, US) at excitation wavelength of 325 and 350 nm. X-ray diffractions patterns were measured by using Cu–Kα (λ = 1.5406Å) radiation having tube voltage with 40 mA current on Mini Flex Rigaku X-ray diffractrometer, recorded between 2θ, 10°–90°. Thus, hexagonal crystal phase of ZnO nanoparticles was established by XRD. Morphology was examined directly by Scanning electron microscopy (SEM) and 20 kV acceleration voltage were used for the analysis. Particle size distribution was measured in DMF solution in the range of 0–100 nm using Sympatech (France) particle size analyzer at a Laser wavelength of 632 nm.
Synthesis of ZnO nanoparticles
Zinc oxide nanoparticles were synthesized as per the reported procedure with slight modification.Citation26 In the present method, the synthesis was performed in aqueous medium by preparing the alkali solution of zinc nitrate by dissolving 0.5M zinc nitrate [Zn(NO3)2.6H2O] and 1M NaOH, in 100 mL distilled water. The solution was continuously stirred and heated at 75°C maintaining the pH 12. Polyvinylpyrrolidone (PVP, 0.2 g) was added to create a capping on the surface of the precursor and the reaction mixture was kept for 5–6 h at 75°C to obtain white suspension of ZnO nanoparticles. The particles were separated from the supernatant dispersion by sedimentation. The supernatant solution was discarded and remaining suspension was washed several times with distilled water and ethanol. The particles were then collected by centrifugation at 5000 rpm for 15–30 min. The white precipitate obtained was dried at 50–75°C and was annealed at 400°C for 2 h in a muffle furnace for formation of highly nanocrystalline ZnO nanoparticles. The sample was characterized by various characterization techniques before loading in polycarbonate matrix.
Preparation of ZnO–PC composite thin `film
As-synthesized ZnO nanoparticles were suspended in chloroform and were blended with pre-dissolved polycarbonate (M.W. 22 000) in chloroform. The amount of ZnO nanoparticles was varied to form various compositions solutions (0.05, 0.2, 0.5 and 1 wt-%) with respect to polycarbonate. The mixture was sonicated for ∼15 min, and the solution was poured onto a borosilicate glass slide. The poured solution was blade coated manually. The film dried almost instantly because of volatile nature of chloroform. The dried films were peeled off after keeping in warm (about 50–60°C) condition for about an hour.
Measurement of antibacterial properties
In vitro antimicrobial activity of the films was tested against gram-positive bacteria S. aureus and gram-negative bacteria E. coli by turbidimetric method.Citation27 Suspensions of S. aureus and E. coli were prepared from fresh colonies after overnight incubation. Equally sized films were placed in tubes with 10 mL LB (Luria Bertini) broth. An aliquot of the bacterial suspension was added to each tube containing films to obtain dilutions of 106 cfu ml− 1. Tubes were then incubated at 37°C in a shaking incubator. Bacterial growth in terms of turbidity was measured post 24 h using Systronics Turbidity Meter 135.
Results and discussion
The as-prepared ZnO nanoparticles were characterized by conventional tools, e.g. UV-visible spectroscopy, XRD and SEM to observe and confirm the formation of phase pure ZnO nanoparticles. It was observed that all the characterization data matched well with the reported data. In-fact, the UV-visible spectra indicated a very sharp cutoff of the PC–ZnO films thereby implying excellent distribution of particles in the film.Citation28,Citation29 Absorption values of about 340 nm signified an excellent size control with large blue shift in the band-gap energy of ZnO (bulk band-gap; Eg = 3.37 eV). Optical absorption measurement of the composite films as well as the film of polycarbonate was measured in the range of 300–800 nm. shows the UV-visible spectra of the film samples indicating absorption bands at 340 nm for ZnO nanoparticles as against ∼370 nm of bulk ZnO. Along with the band because of ZnO nanoparticles, additional band at 300 nm because of polymer was also observed and was well compared with the value obtained for polycarbonate film alone. The absorption band was pronounced for the sample with 0.05 wt-% loading as compared to the other samples. Such strong absorbance was a result of higher thickness of the 0.05 wt-% ZnO-loaded film as compared to 0.5 and 1 wt-% ZnO-loaded films. The blue shift in absorption wavelength is generally related to small particle size in nanostructures.Citation30,Citation31
Figure 1. a UV-visible spectra of polycarbonate (PC)–zinc oxide (ZnO) nanocomposite film with varied ZnO loading and b UV-visible and photoluminescence (PL) (λ(ex) @350 nm) spectra of 1 wt-% ZnO–PC film
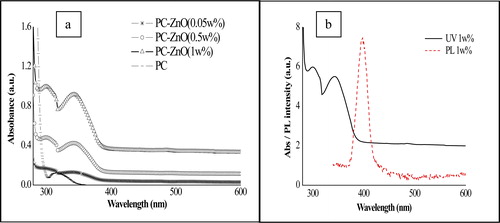
A blue shift also implies increased band-gap energy of the semiconducting materials. Similarly, PL measurement of the film containing 1 wt-% ZnO in polycarbonate () revealed the emission band at ∼402 nm at excitation energy of 320 nm. The band width in PL is found to be ∼50 nm, which was reasonably narrow from ZnO particles dimension point of view thus indicating good distribution of the particle in the matrix. The good UV absorption with relatively low wt-% of ZnO nanoparticles and a homogeneous particles distribution as understood by optical properties could be useful information for applying these composite for food technology related applications.
The crystalline nature and phase studies were also studied by obtaining the diffraction pattern from XRD measurement of ZnO nanoparticles. The obtained XRD spectrum shown in matched well with the diffraction pattern of the bulk ZnO and confirmed the formation of ZnO nanoparticles. All peaks for relevant crystal planes were observed and the particles size as calculated from Debye–Scherrer equation was estimated to be between 15 and 20 nm. The peaks can be assigned to various crystal planes of hexagonal crystal structure of ZnO. Peaks at planes (100), (002), (101), (102), (110), (103), (200), (112) and (201) were observed. Additionally, some minor peaks were also observed beyond 2θ 70°, indicating excellent crystallinity of the sample. Zinc oxide–PC samples (here, 1 wt-% ZnO loading) however showed peaks with reduced intensities, which is obvious because of presence of organic material around the particles leading to loss of crystallinity.
Figure 2. XRD spectra of as-synthesized zinc oxide (ZnO) nanoparticles and its comparison with ZnO– polycarbonate (PC) nanocomposite film and PC film
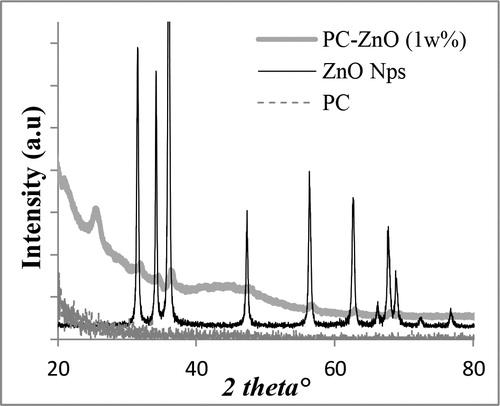
TGA analysis was carried out under nitrogen atmosphere to avoid further oxidation during decomposition stage thereby avoiding formation of any byproduct, which otherwise will lead to false analysis. The wt-% loss as indicated in was found to be more than 95% where it was observed that the composite residue was higher than the polymer alone. Both the samples undergo two-stage decomposition profile at 370 and 520°C. It is also estimated that the decomposition temperature of the composite is about 25°C higher than the PC alone when the loading of ZnO nanoparticles was 1 wt-% and ∼12°C higher for 0.2 wt-% ZnO loading. Such enhanced decomposition temperature or thermal stability can be considered as a useful property for application of this composite material in packaging technology.
Figure 3. TGA profile of polycarbonate (PC) and PC–zinc oxide (ZnO) nanocomposite film; P4 with 1 wt-% and P2 with 0.2 wt-% of ZnO nanoparticles
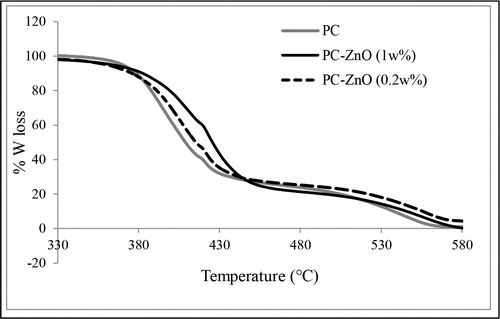
The various films of ZnO nanoparticles loaded polycarbonate (0.2–1 wt-%) exhibited significant antimicrobial activity against both gram-positive (S. aureus) as well as gram-negative (E. coli) bacteria as shown in . However, there was no significant effect shown by polycarbonate film alone which highlights the role of ZnO nanoparticles as an effective antimicrobial agent. The bactericidal activity of metal oxide embedded polymer composites was attributed to their surface-destructive interactions with the cell membranes.Citation32,Citation33 As shown in , increase in the ZnO content showed decrease in turbidity of media post 24 h incubation. There was no significant effect shown by PC (P0) film alone. Thus, antimicrobial activity of ZnO/PC composite films was solely dependent on the concentration of ZnO nanoparticles. It is well known that ZnO nanoparticles complex with thiol (–SH) and such other functionalities of key enzymes, nucleic acid and cellular organelles in the cytoplasm disrupts essential cellular processes of microbial cell.Citation34 Moreover, ZnO nanoparticles stimulate oxidative stress via generation of reactive oxygen species (ROS) in the bacterial cells that arrests cell growth and bring about cell lysis.Citation35
Table 1. Polycarbonate (PC)–zinc oxide (ZnO) nanocomposite films showing antibacterial effect for gram-positive (S. aureus) and gram-negative (E. coli) bacteria
Figure 4. a Antibacterial activity of zinc oxide (ZnO) nanoparticles against S. aureus and E. coli and b related graphical comparison of turbidity as measure of cell viability, c photograph of a typical ZnO–polycarbonate (PC) film
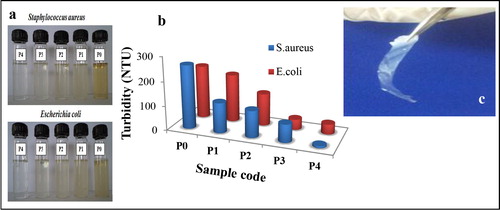
In the comparative analysis (), antibacterial activity of ZnO nanoparticles against gram-positive (S. aureus) bacteria was more than gram-negative (E. coli) bacteria. This difference in antimicrobial spectrum of films was attributed to the morphological variation in bacterial cells.Citation36 Porous and charged peptidoglycan surface of gram-positive bacteria make them more vulnerable to ZnO nanoparticles. However, gram-negative bacteria possess thin peptidoglycan layer protected by less permeable phospholipids bilayer from inside, which increases the bacterial resistance thus are less affected by small ZnO particles.Citation37 Thus, ZnO–PC nanocomposite films shows better, antibacterial property of ZnO–PC against gram-positive bacteria as compared to gram-negative bacteria. The overall antimicrobial mechanism of ZnO–PC nanocomposite is presented in .
SEM image of the as-prepared ZnO nanoparticles () indicates spherical-shaped aggregates of ZnO nanoparticles. Although some clustering was visible, the ZnO nanoparticles were properly re-dispersed in chloroform before loading. As a result, ZnO nanoparticles were nicely embedded and showed uniform distribution of tiny particles in the PC matrix (). Particle size distribution profile of the ZnO nanoparticle is shown in for a suspension that was prepared in chloroform. Dynamic light scattering technique was used for analysis with Laser frequency of 632 nm. The measurement shows that the particles are distributed between 2 and 30 nm with majority being in the range of 15–20 nm. The observed particle size distribution correlated well with the XRD findings.
Figure 6. SEM of a as-synthesized zinc oxide (ZnO) nanoparticles, b polycarbonate (PC)–ZnO film with 1 wt-% of ZnO, (scale bar is 1 μm in both the images) and c particle size distribution profile of as-synthesized ZnO nanoparticles measured by DLS technique. Below, contact angle measurement images of PC and PC–ZnO films d PC film, e 0.2 wt-% ZnO, f 0.5 wt-% ZnO and g 1 wt-% ZnO
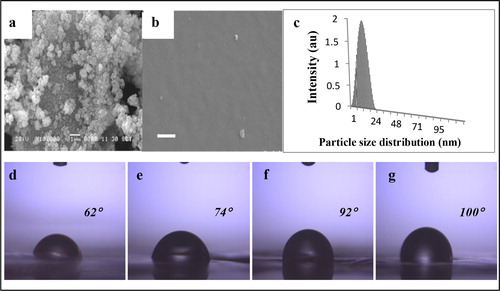
Contact angle measurements of the film were conducted by dropping water droplet on the PC (d) and as-prepared ZnO–PC nanocomposite films (). It was clearly visible that the hydrophobic character of the films increased with the increase in wt-% loading of ZnO nanoparticles in PC. Polycarbonate film without any loading of ZnO nanoparticles showed the expected value for contact angle, i.e. 62°. However, the contact angle was systematically increased to 74°, 83°, 92° and 100° for 0.05 wt-% (not shown), 0.2, 0.5 and 1 wt-% loading of ZnO nanoparticles, respectively. Such increased hydrophobicity could be adequate for self-cleaning properties as ZnO nanoparticles can also actively work as a photo-catalyst. Thus, the as-prepared ZnO–PC nanocomposite films could also be potentially used for self-cleaning applications. However, substantial work is still needed to claim its suitability, such as further increase in ZnO nanoparticles loading to induce further hydrophobicity, its effect on physical properties and transparency of the films.
Conclusion
Zinc oxide–PC nanocomposite films with varied ZnO concentrations were casted by solution-based blade coating method resulted in homogeneous size distribution of ZnO nanoparticles in the PC matrix. The optical properties of the films confirmed the increased band-gap energy of ZnO because of small size as well as to homogeneous nature of the particles within the films. The films were analyzed by various modern characterization techniques such as XRD, UV-visible, photoluminescence spectroscopy, SEM and TGA. All the analysis showed good correlation and improvement in respective properties. The films can be considered potential materials for food packaging application and thus their antibacterial properties, UV-blocking properties and hydrophobicity were tested. Additionally, the results indicated good UV-blocking capability even at low wt-% loading of ZnO nanoparticles along with enhanced bacteriostatic action against S. aureus and E. coli with increase in ZnO loading in the nanocomposite films. As the PC alone does not show any antibacterial activity, the observed antimicrobial activity of ZnO–PC composite films was solely dependent on the concentration of ZnO nanoparticles. It was also observed that the hydrophobic character of the nanocomposite films is systematically enchanced with increasing ZnO wt-% loading in PC. Thus, from the above results, the ZnO–PC nanocomposite films can be considered as potential packaging material.
Conflicts of Interest
Authors declare no conflict of interest.
Acknowledgement
The authors are thankful to Vice-chancellor, DIAT for encouragement and permissions. PKK thanks Director NMRL for research grant under CARS scheme of DRDO. Vividha Dhapte is thankful to Principal, Poona College of Pharmacy for research support.
References
- Von Nussbaum F., Brands M., Hinzen B., Weigand S. and Häbich D.: Angew. Chem. Int. Ed., 2006, 45, 5072–5129.
- Baker-Austin C., Wright M. S., Stepanauskas R. and McArthur J. V.: Trends Microbiol., 2006, 14, 176–182.
- Tiller J. C., Liao C. J., Lewis K. and Klibanov A. M.: Proc. Natl. Acad. Sci. U.S.A., 2001, 98, 5981–5985.
- Lin J., Qiu S., Lewis K. and Klibanov A. M.: Biotechnol. Prog., 2002, 18, 1082–1086.
- Sawai J.: J. Microbiol. Methods, 2003, 54, 177–182.
- Sondi I. and Salopak-Sondi B.: J. Colloid Interface Sci., 2004, 275, 177–182.
- Stoimenov P. K., Klinger R. L., Marchin G. L. and Klabunde K. J.: Langmuir, 2002, 18, 6679–6686.
- Sunada K., Kikuchi Y., Hashimoto K. and Fujishima A.: Environ. Sci. Technol., 1998, 32, 726–728.
- Rosi N. L. and Mirkin C. A.: Chem. Rev., 2005, 105, 1547–1562.
- Ma D., Guan J., Normandin F., Denommee S., Enright G., Veres T. and Simard B.: Chem. Mater., 2006, 18, 1920–1927.
- Huh A. J. and Kwon Y. J.: J. Control. Release, 2011, 156, 128–145.
- Sobha K., Surendranath K. and Meena V.: Biotechnol. Mol. Biol. Rev., 2010, 5, 1.
- Abhulimen U.: Mater. Res. Soc. Symp. Proc., 2005, 1, 27.
- Carri'on F. J., Sanes J. and Bermúdez M.-D.: Wear, 2007, 262, 1504–1510.
- Fu L., Liu Z., Liu Y., Han B., Hu P., Cao L. and Zhu D.: Adv. Mater., 2005, 17, 217–221.
- Makhluf S., Dror R., Nitzan Y., Abramovich Y., Jelinek R. and Gedanken A.: Adv. Funct. Mater., 2005, 15, 1708–1715.
- Reddy K. M., Feris K., Bell J., Wingett D. G., Hanley C. and Punnoose A.: Appl. Phys. Lett., 2007, 90, 213902–213903.
- Szabó T., Németh J. and Dékány I.: Colloids Surf. A Physicochem. Eng. Asp., 2003, 230, 23–35.
- Abramov O. V., Gedanken A., Koltypin Y., Perkas N., Perelshtein I., Joyce E. and Mason T. J.: Surf. Coat. Technol., 2009, 204, 718–722.
- Yamada H., Suzuki K. and Koizumi S.: J. Toxicol. Sci., 2007, 32, 193–196.
- Dutta K., Tripathi S., Mehrotra G. K. and Dutta J.: Food Chem., 2009, 114, 1173–1182.
- Farris S., Unalan I. U., Introzzi L., Fuentes-Alventosa J. M. and Cozzolino C. A.: J. Appl. Polym. Sci., 2014, 131, 40539.
- Arora A. and Padua G. W.: J. Food Sci., 2010, 75, 43–49.
- Haciu D., Saner S., Türdoğru O. and Ünal U.: Front. Sci., 2013, 3, (3), 96–101.
- Sharma D., Rajput J., Kaith B. S., Kaur M. and Sharma S.: Thin Solid Films, 2010, 519, 1224–1229.
- Guo L., Yang S. H., Yang C. L., Yu P., Wang J. N., Ge W. K. and Wong G. K. L.: Chem. Mater., 2000, 12, 2268–2274.
- European Commission Official Publication, Eur. Pharmacopoeia, 2005, 5, 191.
- Giersig M., Pastoriza-Santos I. and Liz-Marzan L. M.: J. Mater. Chem., 2004, 14, 607–610.
- Khanna P. K., Singh N., Kulkarni D., Deshmukh S., Charan S. and Adhyapak P. V.: Mater. Lett., 2007, 61, 3366–3370.
- Khanna P. K., More P., Bharate B. G. and Viswanath A. K.: J. Lumin., 2010, 130, 18–23.
- Khanna P. K., Singh N., Charan S., Lonkar S., Reddy A. S., Patil Y. and Viswanath A. K.: Mater. Chem. Phys., 2006, 97, 288–292.
- Dhapte V., Kadam S., Pokharkar V., Khanna P. K. and Dhapte V.: ISRN Inorg. Chem., 2014, 8, 170919–170927.
- Qian L., Xiao H., Zhao G. and He B.: ACS Appl. Mater. Interfaces, 2011, 3, (6), 1895–1901.
- Love S., Maurer-Jones M., Thompson J., Lin Y. and Haynes C.: Annu. Rev. Anal. Chem., 2012, 5, 181–205.
- Wahab R., Kim Y. S., Mishra A., Yun S. I. and Shin H. S.: Nanoscale Res. Lett., 2010, 5, (10), 1675–1681.
- Xie Y., He Y., Irwin P. L., Jin T. and Shi X.: Appl. Environ. Microbiol., 2011, 77, (7), 2325–2331.
- Tortora G., Funke B. and Case C.: ‘Microbiology – an introduction’, 9th edn; 2009, San Francisco, CA, Pearson, Benjamin Cummings.