Abstract
Acute radiation exposure induces apoptosis of tissues in the hematopoietic, digestive, cutaneous, cardiovascular and nervous systems; extensive apoptosis of these tissues ultimately leads to acute radiation syndrome. A novel strategy for developing radiation countermeasures has been to imitate the genetic mechanisms acquired by radiation-resistant tumors. Two mechanisms that underlie this ability of tumor cells are the p53 and NF-κB pathways. The loss of p53 function results in the inactivation of pro-apoptotic control mechanisms, while constitutive activation of NF-κB results in the up-regulation of anti-apoptotic genes. Various Toll-like receptor ligands are capable of up regulating the NF-κB pathway, which increases radio-resistance and reduces radiation-induced apoptosis in various tissues. Several Toll-like receptor ligands have been patented and are currently under development as radiation countermeasures for acute radiation syndrome. Ongoing studies suggest that a few of these attractive agents are progressing well along the US FDA approval pathway to become radiation countermeasures.
1. Introduction
Due to the increasing prevalence of radioactive material, incidences involving radiation exposure, either intended or unintended, are an undeniable possibility with potentially catastrophic consequences Citation[1]. Such concerns have justified substantial efforts to develop medically effective radiation countermeasures for ARS Citation[2,3]. Although such efforts were initiated > 6 decades ago, only one radiation countermeasure, granulocyte colony-stimulating factor (G-CSF), has recently been approved by the US FDA for ARS Citation[4,5]. Countermeasures can be categorized based on their administration in relation to the time of radiation exposure. Radioprotectors, also called prophylactic agents, must be administered before radiation exposure to prevent injury by acting prior to and during the initial radiochemical events. Radiation mitigators are administered after irradiation but before the appearance of overt evidence of injury to accelerate recovery. Radiation therapeutics or treatments are the agents given after symptoms manifest to stimulate repair or regeneration of organ and tissue functions Citation[2].
High-dose whole-body irradiation induces inflammation, massive apoptosis, necrosis, and susceptibility to infection. All three leukocyte populations (lymphocytes, monocytes, and granulocytes) fall within a few days after exposure, reaching their respective nadirs in several days. Other blood cell populations (e.g., erythrocytes, platelets) decline slowly over a several-week-long period due to reduced hematopoietic activity with the exception of granulocytes. Cellular determinants of the adaptive immune response are significantly more sensitive than the majority of components of innate immunity (e.g., macrophage populations and natural killer cells) with the exception of granulocytes. However, significant decline in neutrophils (neutropenia) increases the risk and severity of infections Citation[6,7].
Effective protection from irradiation requires the defense of existing mature immune cells and the rescue of precursor and stromal cells as both are essential for regeneration and recovery. Relative sensitivity also varies greatly among different tissue components Citation[8]. Mature B- and T-cells are the most sensitive to irradiation and will undergo apoptosis after exposure to < 1 Gy, whereas hematopoietic precursors are significantly more resistant and can regenerate the immune system even after exposure to 10 Gy in mice. Bone marrow stroma is the most resistant component of immune system, which can survive exposure to level as high as 15 – 17 Gy, although functional alterations can be observed at lower doses.
2. Biological responses to radiation
Exposure to ionizing radiation produces breaks and cross-links in the DNA, and alters proteins, cell membranes, and other macromolecular structures. Ionizing radiation also induces secondary damage to the cellular components by creating free radicals and reactive oxygen species (ROS). Several pathways protect and restore the integrity of the DNA. For example, antioxidant chemicals and enzymes scavenge the free radicals and ROS in addition to reducing the levels of oxidized proteins and lipids. Cellular checkpoint systems detect DNA defects and delay cell cycle progression until the damage is repaired, the cell commits to permanent growth arrest, or the cell initiates programmed death (apoptosis).
At the organismal level, the immediate effects of low- and moderate-intensity radiation are largely caused by cell death, necrosis, or by the bystander effect. These effects cause radiation-induced inflammation Citation[9-11]. At high doses, lethality is caused by the hematopoietic and gastrointestinal radiation syndromes (H-ARS and GIS, respectively). H-ARS is the loss of blood cells and their progenitors, which makes it impossible to regenerate the blood and lymphoid systems. This process leads to death from hemorrhage, anemia and infection. GIS is caused by massive cell death in the intestinal epithelium, predominantly in the small intestine, followed by disintegration of the intestinal wall and death from bacteremia and sepsis. Another important syndrome of radiation exposure is cutaneous syndrome. Exposure of the human skin to ionizing radiation > 3 Gy results in a distinct clinical representation, characterized by a transient and faint erythema after a few hours, and then followed by severe erythema, blistering, and necrosis.
3. Role of NF-κB in radiation-induced apoptosis
The important radioprotective strategy is to activate the NF-κB pathway. The protective role of NF-κB is mediated by the transcriptional activation of genes coding for proteins that block major apoptotic pathways Citation[12]. The NF-κB pathway makes an attractive target because it is activated by several naturally occurring factors that are considered radioprotectants. Sources of NF-κB activators include tumor cells and microbial parasites of mammals, since both employ inhibition of apoptosis for survival in the host. The idea of using NF-κB pathway induction to inhibit apoptosis was initially tested using Salmonella typhimurium flagellin, which activates NF-κB through Toll-like receptor 5 (TLR5) () Citation[13]. NF-κB regulates anti-apoptotic genes especially the TNF receptor-associated factor 1 and 2 (TRAF1 and TRAF2) and, therefore, checks the activities of the caspase enzyme family, which are central to most apoptotic processes. Bacterial lipoproteins are ligands for TLR2 which also activate NF-κB. The immunomodulatory properties of lipoproteins and their effects on hematopoietic cytokines make small synthetic lipopeptides excellent candidates as radiation countermeasures. Consequently, several patents have been filed for different TLR ligands for development as candidate radiation countermeasures () Citation[14-26].
Figure 1. Schematic representation of TLR-ligand-mediated NF-κB activation. TLRs bind microbial PAMPs leading to the activation of NF-κB and IRF pathways. Activation of these pathways is mediated by the two key adaptor molecules MyD88 and TRIF. NF-κB enters the nucleus where it ‘turns on’ the expression of specific genes resulting in inflammatory, immune, or cell survival response. Key biological effects of NF-κB activation include the prevention of apoptosis. A set of endosomal TLRs recognize nucleic acids derived from viruses and endogenous nucleic acids.
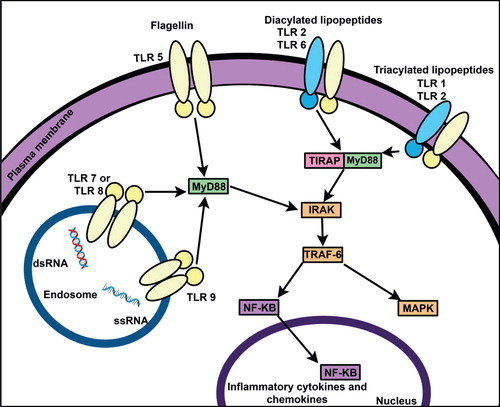
Table 1. Promising TLR ligands under development as radiation countermeasures.
4. Toll-like receptors and activation of NF-κB
TLRs are the key sensor elements of innate immunity and are evolutionary conserved receptors. They are homologues of the Drosophila Toll protein which is important for the defense against microbial infection. TLRs recognize highly conserved structural motifs known as pathogen-associated molecular patterns (PAMPs) (exclusively expressed by microbial pathogens) or damage/danger-associated molecular patterns (DAMPs) (endogenous molecules released from necrotic or dying cells). Stimulation of TLRs, by PAMPs and DAMPs, initiates signaling cascades which lead to the activation of transcription factors, such as NF-κB (). TLR signaling results in a variety of cellular responses including the production of interferons, pro-inflammatory cytokines, and effector cytokines that direct the adaptive immune response. A total of 10 human and 12 murine TLRs have been well characterized ().
Table 2. Well characterized TLRs of mouse and human.
Consistent with their function of immunocyte activation, TLRs are expressed in spleen and peripheral blood leukocytes. Other TLR-specific patterns are expressed in other lymphoid organs and subsets of leukocytes as well as other tissues and organs. TLR ligands are appealing as potential radioprotectors since they are characteristic of large groups of pathogens, and cannot be easily mutated. Unlike cytokines, many PAMPs have little effect besides activating TLRs and thus are unlikely to produce side effects. Many PAMPs (and DAMPs) are constantly present in humans. Several TLR ligands are under different stages of the development as radiation countermeasures ().
Table 3. Promising TLR ligands under development as radiation countermeasures for ARS.
5. Development of TLR ligands as radiation countermeasures
Flagellin of Salmonella typhimurium is a stable protein and natural activator of NF-κB which has been shown to protect mice from lethal doses of total-body irradiation. A truncated version of this protein has been developed (CBLB502) which retains the radioprotective efficacy and stability while lacking its immunogenic properties. CBLB502 has been found to be effective against both H-ARS and GIS in mice and nonhuman primates (NHPs), both as a radioprotector and also as a radiomitigator Citation[27,28]. These attributes make CBLB502 uniquely useful as a radiation countermeasure for civilian and military applications. CBLB502 has received FDA investigational new drug status and is currently in clinical development. A human safety study indicated that it was well tolerated in humans and the biomarker results correspond to data from animal models Citation[28].
A shortened version of a naturally occurring N-terminal lipopeptide from Mycoplasma arginine, R-Pam2-CGETDK (S-[(2R)-2,3-bis(palmitoyloxy)propyl]-cysteinyl-GETDK) (CBLB613), is water soluble, activates TLR2/TLR6-dependent NF-κB production, and demonstrates significant radioprotective efficacy in murine model of ARS Citation[29]. Mycoplasma arginine is a symbiotic asymptomatic microflora of mammals, commonly present in humans and is expected to be safe. A synthetic mimetic of diacylated mycoplasma lipopeptides (Pam2-CSKKKK, agonists of TLR2, CBLB612) has also been shown to have radioprotective and radiomitigative efficacy in murine model of ARS Citation[30]. The TLR agonists are activators of anti-apoptosis pathways and their role in carcinogenesis deserves investigation. Although the role of TLRs in cancer is not well understood, results suggest a dual role of TLRs in cancer: high doses of TLR agonists appear to have an anti-cancer effect whereas low doses of TLR agonists promote cancer growth Citation[31].
6. Expert opinion
Currently, G-CSF is the only one FDA-approved countermeasure to mitigate ARS-related morbidity and mortality. It has been procured for Strategic National Stockpile for use in a radiological emergency. However, the adverse consequences of G-CSF administration need to be taken into consideration. For examples, G-CSF administration after cytotoxic agents that damage bone marrow stem cells exacerbates long-term stem cell damage through excessive differentiation stimulation Citation[5]. Additional concerns stem from its role in exacerbating delayed lung damage in an animal model of ARS. Finally, in a recent study, G-CSF failed to demonstrate radiomitigative efficacy in the NHP model. This failure may be due to the lack of supportive care Citation[5]. It is important to note that the European standard for the evaluation and treatment of ARS recommends the use of cytokines 14 – 21 d post-exposure to promote hematological reconstruction, and there are several studies in which G-CSF has been used for the treatment of radiation accident victims with beneficial effects Citation[5,32,33].
Fortunately, there are several promising radiation countermeasures for ARS at different stages of development Citation[2]. In the recent past, several patents have been filed for the use of TLR ligands as radiation countermeasures, specifically as anti-apoptotic agents. Among these products, CBLB502 (truncated flagellin) and lipopeptides of mycoplasma origin are promising agents. CBLB502 is the leading candidate currently under development, as this agent has been evaluated as a radioprotector as well as a radiomitigator with promising results in NHPs. A good laboratory practice compliant study with CBLB502 has also been conducted in NHPs. In addition, this agent has undergone clinical trial for toxicity, pharmacokinetics, and biomarkers in healthy volunteers. Its existing efficacy, safety data, and animal-to-human dose conversion are enough to proceed with a pre-emergency use authorization (EUA) application to reduce the risk of death following radiation exposure. In our opinion, CBLB502 holds the most promise for the future due to its limited side effects and studies indicate it is likely to be a safe and effective agent when approved.
CBLB612 and CBLB613 are at early stages of development and their promising efficacy in a murine model has been reported Citation[29,30]. However these peptides, along with CBLB502, are derived from human pathogens/symbionts (Salmonella and Mycoplasma), pre-existing immunity in the host may be an issue. Efforts are, therefore, being made to identify hyperthermophilic flagellin derivatives to which the human immune system has not yet been exposed. These derivatives are also stable, have a relatively low molecular weight and can be incorporated with de-immunizing deletions and mutations.
Acknowledgments
The opinions or assertions contained herein are the professional views of the authors and are not necessarily those of the Department of Defense, USA. The authors gratefully acknowledge the research support from various funding agencies of US Department of Defense. The mention of specific therapeutic agents does not constitute endorsement by the U.S. Department of Defense, and trade names are used only for the purpose of clarification. We apologize to those having contributed substantially to the topics discussed herein that we were unable to cite due to space constraints.
Declaration of interest
The authors have no relevant affiliations or financial involvement with any organization or entity with a financial interest in or financial conflict with the subject matter or materials discussed in the manuscript. This includes employment, consultancies, honoraria, stock ownership or options, expert testimony, grants or patents received or pending, or royalties.
Notes
Bibliography
- Carter AB, May MM, Perry WJ. The day after: action following a nuclear blast in a U.S. city. Washington Quarterly 2007;30:19-32
- Singh VK, Newman VL, Romaine PL, et al. Radiation countermeasure agents: an update (2011 - 2014). Expert Opin Ther Pat 2014;24:1229-55
- Singh VK, Romaine PL, Newman VL. Biologics as countermeasures for acute radiation syndrome: where are we now? Expert Opin Biol Ther 2015;15:465-71
- U.S. Food and Drug Administration. FDA approves Neupogen® for treatment of patients with radiation-induced myelosuppression following a radiological/nuclear incident. 2015. Available from: http://www.fda.gov/EmergencyPreparedness/Counterterrorism/MedicalCountermeasures/AboutMCMi/ucm443245.htm [Last accessed 17 April 2015]
- Singh VK, Newman VL, Seed TM. Colony-stimulating factors for the treatment of the hematopoietic component of the acute radiation syndrome (H-ARS): a review. Cytokine 2015;71:22-37
- Gridley DS, Pecaut MJ, Dutta-Roy R, Nelson GA. Dose and dose rate effects of whole-body proton irradiation on leukocyte populations and lymphoid organs: part I. Immunol Lett 2002;80:55-66
- Pecaut MJ, Gridley DS, Smith AL, Nelson GA. Dose and dose rate effects of whole-body proton-irradiation on lymphocyte blastogenesis and hematological variables: part II. Immunol Lett 2002;80:67-73
- Singh VK, Newman VL, Berg AN, MacVittie TJ. Animal models for acute radiation syndrome drug discovery. Expert Opin Drug Discov 2015;10:497-517
- Hall EJ, Giaccia AJ. Radiobiology for the Radiobiologist. 7th edition. Lippincott Williams and Wilkins; Philadelphia, PA; 2012
- Lataillade JJ, Doucet C, Bey E, et al. New approach to radiation burn treatment by dosimetry-guided surgery combined with autologous mesenchymal stem cell therapy. Regen Med 2007;2:785-94
- Muller K, Meineke V. Radiation-induced alterations in cytokine production by skin cells. Exp Hematol 2007;35:96-104
- Karin M, Lin A. NF-kappaB at the crossroads of life and death. Nat Immun 2002;3:221-7
- Hayashi F, Smith KD, Ozinsky A, et al. The innate immune response to bacterial flagellin is mediated by Toll-like receptor 5. Nature 2001;410:1099-103
- Gudkov A. Modulating apoptosis. US7638485B2, US8106005, US8784840, US20060275255, US20100056454, US20100120687, US20150072931; 2009
- Gudkov A. Method for reducing the effects of chemotheraphy using flagellin related polypeptides. WO2009102818. US8580321, US20090246303, US20130324462; 2009
- Gudkov A, DiDonato J, Krivolkrysenko V. Flagellin related polypeptides and uses thereof. EP01838340A1, EP02460534A1, IL184140D0, IL227910D0, JP2008525472A, JP5285278B2, US08007812B2, US08287882B2, US08932609B2, US20090011982A1, US20110319344A1, US20110319595A1, US20140294900A1, WO2006069198A1; 2014
- Gudkov A. Methods of protecting against radiation using flagellin. CA2547869A1, CA2547869C, CN1913915A, CN1913915B, DE602004029500D1, EP1706133A2, EP1706133B1, WO2005056042A3, WO2005056055A2, WO2005056055A3, WO2005057218A2, WO2005057218A3; 2005
- Gudkov A, Burdelya L. Compositions and methods comprising Toll-like receptor (TLR) stimulating agents for prophylaxis and therapy for damage to dermal epithelium. WO2013151994, EP2833876A1; 2013
- Mogelsvang S, Gelber C. Peptides and methods of using same. US20140378372A1, CA2859777A1, EP2802338A2, US8975224, US20130274187, WO2013106273A2, WO2013106273A3; 2014
- Mogelsvang S, Gelber C. Peptides and methods of using same. WO 2013106273 A2 20130718, WO 2013106273 A3 20131219, AU 2013208293 A1 20140626, CA 2859777 A1 20130718, EP 2802338 A2, 20141119, IL 233481 D0 20140831, JP 2015504073 A 20150205, MX 2014007799 A 20141024, US 2013274187 A1 20131017, US 2014378372. A1 20141225, US 2015133375 A1 20150514, US 8975224 B2 20150310; 2015
- Shakhov A, Strom E. Methods for increasing and mobilizaing hematopoietic stem cells. WO 2008086426 A2 20080717, WO 2008086426 A3 20081218, WO 2008086426 A8 20090730, AU 2008204836 A1 20080717, BR PI0806557, A2 20140415, CA 2675032 A1 20080717, CN 101631850 A 20100120, CN 101631850 B 20131204, EA 018983 B1 20131230, EA 200900806, A1 20100830, EP 2115124 A2 20091111, EP 2115124 A4 20120530, HK 1134835 A1 20140808, IL 199766 A 20150129, JP 2010515748 A, 20100513, JP 5389666 B2 20140115, KR 101493474 B1 20150216, KR 20090108703 A 20091016, MX 2009007391 A 20090813, NZ 578928 A, 20130328, NZ 603805 A 20140328, SG 177959 A1 20120228, US 2010055077 A1 20100304, US 2014045747 A1 20140213, ZA 200905378 A 20100526; 2012
- Shakhov A, Strom E. Methods for increasing and mobilizing hematopoietic stem cells. CA2675032A1, CN101631850A, CN101631850B, EP2115124A2, EP2115124A4, US20100055077, WO2008086426A2, WO2008086426A3, WO2008086426A8; 2014
- Shakhov A, Gudkov A. Methods of protecting against apoptosis using lipopeptides. US8008260B2, CA2612102A1, CN101242852A, CN101242852B, EP1904084A2, EP1904084A4, EP1904084B1, EP2554181A2, EP2554181A3, EP2554181B1, US8524668, US9006183, US20090214467, US20110237507, US20140045750, WO2006138238A2, WO2006138238A3; 2011b
- Shakhov A, Gudkov A. Methods of protecting against apoptosis using lipopeptides. AU2006259630A1, AU2006259630B2, BRPI0611586A2, CA2612102A1, CN101242852A, CN101242852B, EA014644B1, EA200702510A1, EP01904084A2, EP01904084B1, EP02554181A2, EP02554181B1, ES2421447T3, HK1123495A1, IL188091A, IL188091D0, JP2008543847A, JP5000644B2, KR20080030566A, KR20140041874A, MX2007015834A, NZ565063A, PT1904084E, US08524668B2, US09006183B2, US20090214467A1, US20110237507A1, US20140045750A1, WO2006138238A2, ZA200800126A; 2011a
- Gleiberman A, Burdelya L, Gudkov A. Use of Toll-like receptor agonist for treating cancer. EP2663367A4, CA2824438A1, CN103476458A, EP2663367A1, US20140248260, WO2012097012A1; 2014
- Gleiberman A, Burdelya L, Gudkov A. Use of toll-like receptor agonist for treating cancer. WO 2012097012 A1 20120719, AU 2012205681 A1 20130718, CA 2824438 A1 20120719,CL 2013002001 A1 20140328, CN 103476458 A 20131225, CO 6781506 A2 20131031, EA 201390843 A1 20131230, EP 2663367 A1 20131120, EP 2663367 A4 20140806, IL 227308 D0 20130930, JP 2014502973 A 20140206, KR 20140030132 A 20140311, MX 2013007967 A 20130821, SG 191830 A1 20130830, US 2014248260 A1 20140904; 2014
- Burdelya LG, Krivokrysenko VI, Tallant TC, et al. An agonist of Toll-like receptor 5 has radioprotective activity in mouse and primate models. Science 2008;320:226-30
- Krivokrysenko VI, Shakhov AN, Singh VK, et al. Identification of granulocyte colony-stimulating factor and interleukin-6 as candidate biomarkers of CBLB502 efficacy as a medical radiation countermeasure. J Pharmacol Exp Ther 2012;343:497-508
- Singh VK, Ducey EJ, Fatanmi OO, et al. CBLB613: A TLR 2/6 agonist, natural lipopeptide of Mycoplasma arginini, as a novel radiation countermeasure. Radiat Res 2012;177:628-42
- Shakhov AN, Singh VK, Bone F, et al. Prevention and mitigation of acute radiation syndrome in mice by synthetic lipopeptide agonists of Toll-like receptor 2 (TLR2). PLoS One 2012;7:e33044
- Kluwe J, Mencin A, Schwabe RF. Toll-like receptors, wound healing, and carcinogenesis. J Mol Med (Berl) 2009;87:125-38
- Waselenko JK, MacVittie TJ, Blakely WF, et al. Medical management of the acute radiation syndrome: recommendations of the Strategic National Stockpile Radiation Working Group. Ann Intern Med 2004;140:1037-51
- Gourmelon P, Benderitter M, Bertho JM, et al. European consensus on the medical management of acute radiation syndrome and analysis of the radiation accidents in Belgium and Senegal. Health Phys 2010;98:825-32