Abstract
New technologies have recently been developed to control the expression of human genes in their native genomic context by engineering synthetic transcription factors that can be targeted to any DNA sequence. The ability to precisely regulate any gene as it occurs naturally in the genome provides a means to address a variety of diseases and disorders. This approach also circumvents some of the traditional challenges of gene therapy. In this editorial, we review the technologies that have enabled targeted human gene activation, including the engineering of transcription factors based on zinc finger proteins, transcription activator-like effectors and the CRISPR/Cas9 system. Additionally, we highlight examples in which these methods have been developed for therapeutic applications and discuss challenges and opportunities.
1. Introduction
For more than 40 years, gene therapy has been proposed as an approach to cure genetic diseases by adding functional copies of genes to the cells of patients with defined genetic mutations. However, this field has been limited by the available technologies for adding extra genetic material to human genomes. More recently, several new technologies have emerged for manipulating genes in their native chromosomal context, including editing of gene sequences and regulating target gene expression. In this editorial, we specifically discuss therapeutic applications of activating human genes for treating genetic diseases and enhancing regenerative medicine strategies.
2. Technologies for targeted gene regulation
In order to control the expression of specific genes, it is necessary to develop molecular tools that precisely recognize DNA sequences in the context of the 3 billion base pairs of the human genome. Over the past 20 years, scientists have developed several methods for re-engineering naturally occurring proteins to recognize any desired genomic target ().
Figure 1. Technologies for engineering programmable DNA-binding proteins, including (A) zinc finger proteins, (B) TALEs and (C) CRISPR/Cas9. (Top) Representative crystal structures of a (A) zinc finger protein (PDB 1P47) or (B) TALE (PDB 3UGM) fused to the p65 transcriptional activation domain (PDB 2RAM) or (C) Cas9 (green) bound to a gRNA (blue) and the corresponding DNA target site (brown) (PDB 4OO8). (Bottom) Zinc finger proteins and TALEs recognize their target sequences through non-covalent protein-DNA interactions with 3 bp or 1 bp per repeat domain, respectively. The CRISPR system consists of a complex of the gRNA and the Cas9 protein that recognizes its genomic target sequence by complementary base pairing of the gRNA (spheres) to the chromosomal DNA.
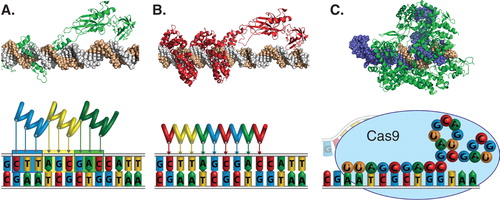
2.1 Zinc finger transcription factors
The first such tools developed for targeted gene activation were based on engineered zinc finger proteins. Zinc finger proteins are the most common class of DNA-binding proteins across all domains of life and recognize specific target sequences through non-covalent interactions of each zinc finger motif with three or four base pairs of DNA. Individual domains can be linked in series to generate arrays that recognize extended sequences (). Many zinc finger domains that target different sequences have been engineered by rational design or high-throughput selection. Because of the modular nature of the zinc finger arrays, it is possible to exchange zinc finger domains to reprogram the binding specificity of the protein to new sequences. The new targeted DNA-binding proteins can be genetically fused to various actuator domains to generate specific outputs. Fusion to potent transcriptional activation domains, such as the monomer or oligomer of the VP16 acidic transactivator, is commonly used to enhance transcription of target genes (). The first synthetic transcription factors engineered to activate the expression of endogenous human genes were designed based on this approach Citation[1]. Despite many successes of developing particular zinc finger proteins for different applications, some of which are discussed in more detail below, the protein engineering challenges of this approach have thus far prevented widespread adoption of the technology.
2.2 Transcription activator-like effectors
More recently, a new class of modular DNA-binding proteins was discovered based on the transcription activator-like effector (TALE) proteins of the plant bacterial pathogen Xanthomonas. Similar to zinc finger proteins, individual domains are linked together in series to determine DNA-binding specificity. But unlike zinc fingers, each modular repeat recognizes only a single base pair of DNA (). The frequency of successfully engineering functional TALE proteins targeted to new sequences is very high. However, the magnitude of gene activation by these proteins at native chromosomal loci was relatively modest Citation[2,3]. Subsequently, we and others demonstrated that multiple TALE activators targeted to single promoters act synergistically to induce high expression levels of the target genes and their protein products Citation[4,5].
2.3 CRISPR/Cas9
Over the past year, a third technology has emerged for facile targeting of specific genomic loci that is based on the CRISPR/CRISPR-associated (Cas) adaptive immunity system in bacteria. In its engineered form, this system comprises a Cas9 endonuclease and a single-stranded guide RNA (gRNA) that forms a complex with Cas9 and targets specific genomic sites based on complementary pairing of a 20 base pair ‘protospacer’ sequence (). In contrast to zinc finger proteins and TALEs, which require design and assembly of new proteins for each new target site, the CRISPR system requires only the exchange of the 20 base pair protospacer within the gRNA expression cassette. Therefore, this system is particularly simple to use and has quickly been implemented for diverse studies. In order to adapt the Cas9 nuclease for targeted gene activation, we and others have fused transcriptional activation domains to a Cas9 mutant in which the nuclease activity has been abolished Citation[6-11]. This results in a programmable RNA-guided gene activation system that can be used to control diverse human genes Citation[6-11] but does not require protein engineering expertise.
3. Human gene activation for treating genetic disease
Hundreds of genetic diseases are caused by the mutation of a single gene. The majority of gene therapy efforts are focused on developing curative strategies based on the addition of functional copies of the affected gene or correction of the mutant gene with genome editing technologies. However, many of these genetic defects can also be compensated for by activation of other endogenous genes with similar function (). For example, sickle cell disease is caused by mutation to the β-globin gene. This mutation can be compensated for by re-activation of the γ-globin gene that has similar function to β-globin but is only expressed during fetal development and is epigenetically silenced during adult life. As a potential therapy for sickle cell disease, γ-globin has been successfully activated by synthetic zinc finger transcription factors Citation[12]. In a similar set of studies, zinc finger transcription factors have been used to upregulate the expression of utrophin, which can compensate for the loss of dystrophin expression that occurs in Duchenne muscular dystrophy Citation[13]. TALE-based activators targeted to the frataxin gene have also been used to increase expression that is downregulated in cells from Friedreich’s ataxia patients due to a trinucleotide repeat expansion in the first intron Citation[14].
Table 1. Examples of therapeutic applications of engineered transcriptional activators.
In addition to monogenic hereditary diseases, other complex genetic diseases, such as cancer and pathologic neovascularization, can potentially be treated by activation of single genes. For example, the tumor suppressor maspin is epigenetically silenced in several types of epithelial tumors. Re-activation of maspin with zinc finger transcription factors reduced tumor cell invasion in vitro and tumor xenograft growth in vivo Citation[15]. In another study, zinc finger transcription factors that activated the expression of the proapoptotic factor Bax induced cell death selectively in cancer cells with inactivation of the tumor suppressor p53 but not in cells with normal p53 Citation[16].
Pathological neovascularization occurs during the course of several diseases and often results from an imbalance between stimulators and inhibitors of angiogenesis. Although many therapeutic efforts focus on inhibiting the angiogenic factor VEGF, inducing expression of the anti-angiogenic factor pigment endothelium-derived factor (PEDF) using zinc finger transcription factors provides a protective response in choroidal neovascularization due to inhibition of multiple proangiogenic growth factors simultaneously Citation[17].
4. Human gene activation for regenerative medicine
Beyond compensation for genetic defects, precise control of endogenous gene expression can provide a means for enhancing tissue regeneration and directing cell lineage specification (). For example, upregulation of multiple VEGF isoforms expressed from the endogenous gene by zinc finger transcription factors stimulated improved angiogenesis and wound healing relative to overexpression of a single VEGF isoform, presumably by more accurately recapitulating natural VEGF signaling Citation[18]. This approach was later explored in Phase II clinical trials for treating both diabetic neuropathy and amyotrophic lateral sclerosis.
One of the most notable applications of modulating cellular gene expression has been the development of genetic reprogramming to redirect cell lineage specification, including the reprogramming of induced pluripotent stem cells (iPSCs) and direct conversion between cell phenotypes. The genetic reprogramming of cell fate may ultimately form the basis of patient-specific cell therapies. These strategies rely on the forced expression of master transcription factors that govern lineage-specific gene regulatory networks. Typically, complete reprogramming is defined as the irreversible activation of the endogenous promoters that control these master transcription factors that occurs as part of the global reprogramming of the lineage-specific gene regulatory network. In attempts to increase the frequency, speed or robustness of reprogramming, recent studies have replaced lineage-specific master transcription factors with synthetic transcription factors targeted to the promoters that control the genes encoding the master factor. For example, TALE transcription factors targeted to the mouse Oct-4 promoter in neural stem cells induced the expression of both Oct-4 and its downstream target Nanog, although only in the presence of DNA methyltransferases or histone deacetylases inhibitors Citation[19]. Subsequently, another study showed that by targeting TALE transcriptional activators to the mouse Oct-4 enhancers, in contrast to the promoter, these synthetic transcription factors could substitute for Oct-4 overexpression in the reprogramming of mouse embryonic fibroblasts to iPSCs Citation[20]. Similar results were achieved by targeting Nanog enhancers in epiblast stem cells.
5. Expert opinion
As described above, the potential for activation of endogenous human genes in the development of innovative therapeutics is immense. Although a few examples have been demonstrated with the early technologies for engineering synthetic transcription factors, these approaches can be expanded to address a diverse range of diseases and conditions. Furthermore, the recent developments in engineering synthetic transcription factors will rapidly advance the pace of success in this field. For example, the ability to engineer new transcription factors by simply exchanging short 20 bp targeting sequences of the CRISPR/Cas system will facilitate the parallel testing of many new synthetic gene activators without the need for protein engineering expertise Citation[6-11]. Similarly, the demonstration of synergistic gene activation by multiple transcription factors has provided insights for achieving results that are significantly more robust than previous methods with single factors Citation[4,5].
However, several challenges remain. It is not clear how to best deliver many factors to activate a single gene to achieve synergistic effects, particularly when working with the highly recombinogenic TALE-encoding DNA sequences. Additionally, exogenous regulation of the synthetic transcription factors may be critical to ensuring safety and efficacy, particularly for applications requiring dynamic control of gene expression. Systems that provide chemical control, or more recently light-based control with optogenetic methods, are examples of advances being made in this area. Enzymatic domains that provide more specific or precise manipulation of genomic regulation, such as epigenetic modifiers of histone marks and DNA methylation, will also enable new strategies for gene regulation. Other important remaining challenges to achieving clinical efficacy include determining optimal delivery methods, characterizing the specificity of gene regulation, assessing immunogenicity of the synthetic proteins involved in each system and improving the robustness of the effects generated by the synthetic factors.
The significant advances made in the last year in technologies for activating human genes are already rapidly accelerating the pace at which these methods are applied to the preclinical development of human therapeutics. Although significant challenges still exist, parallel advances in the fields of gene therapy, immunology, genetics and genomics are providing solutions for overcoming these obstacles. Consequently, it will not be surprising if promising therapeutic candidates based on targeted gene activation emerge in the near future. At a minimum, we expect that the technologies will enable the discovery of novel targets that could then be addressed by more conventional strategies.
Declaration of interest
This work was supported by a US National Institutes of Health (NIH) Director’s New Innovator Award (DP2OD008586), National Science Foundation (NSF) Faculty Early Career Development (CAREER) Award (CBET-1151035), NIH R01DA036865, NIH R03AR061042 and an American Heart Association Scientist Development Grant (10SDG3060033). The authors are inventors on patent applications related to genome engineering. CAG is a consultant in the area of genome engineering.
Acknowledgements
We apologize to authors of relevant works that were not cited here due to space restrictions. We also thank the lab members and collaborators who contributed to our efforts in this area.
Bibliography
- Beerli RR, Dreier B, Barbas CF III. Positive and negative regulation of endogenous genes by designed transcription factors. Proc Natl Acad Sci USA 2000;97:1495-500
- Zhang F, Cong L, Lodato S, et al. Efficient construction of sequence-specific TAL effectors for modulating mammalian transcription. Nat Biotechnol 2011;29:149-53
- Miller JC, Tan S, Qiao G, et al. A TALE nuclease architecture for efficient genome editing. Nat Biotechnol 2011;29:143-8
- Perez-Pinera P, Ousterout DG, Brunger JM, et al. Synergistic and tunable human gene activation by combinations of synthetic transcription factors. Nat Methods 2013;10:239-42
- Maeder ML, Linder SJ, Reyon D, et al. Robust, synergistic regulation of human gene expression using TALE activators. Nat Methods 2013;10:243-5
- Gilbert LA, Larson MH, Morsut L, et al. CRISPR-mediated modular RNA-guided regulation of transcription in eukaryotes. Cell 2013;154:442-51
- Perez-Pinera P, Kocak DD, Vockley CM, et al. RNA-guided gene activation by CRISPR-Cas9-based transcription factors. Nat Methods 2013;10:973-6
- Maeder ML, Linder SJ, Cascio VM, et al. CRISPR RNA-guided activation of endogenous human genes. Nat Methods 2013;10:977-9
- Mali P, Aach J, Stranges PB, et al. CAS9 transcriptional activators for target specificity screening and paired nickases for cooperative genome engineering. Nat Biotechnol 2013;31:833-8
- Cheng AW, Wang H, Yang H, et al. Multiplexed activation of endogenous genes by CRISPR-on, an RNA-guided transcriptional activator system. Cell Res 2013;23:1163-71
- Farzadfard F, Perli SD, Lu TK. Tunable and multifunctional eukaryotic transcription factors based on CRISPR/Cas. ACS Synth Biol 2013;2:604-13
- Graslund T, Li X, Magnenat L, et al. Exploring strategies for the design of artificial transcription factors: targeting sites proximal to known regulatory regions for the induction of gamma-globin expression and the treatment of sickle cell disease. J Biol Chem 2005;280:3707-14
- Di Certo MG, Corbi N, Strimpakos G, et al. The artificial gene Jazz, a transcriptional regulator of utrophin, corrects the dystrophic pathology in mdx mice. Hum Mol Genet 2010;19:752-60
- Chapdelaine P, Coulombe Z, Chikh A, et al. A potential new therapeutic approach for Friedreich ataxia: induction of Frataxin expression with TALE proteins. Mol Ther Nucleic Acids 2013;2:e119
- Beltran A, Parikh S, Liu Y, et al. Re-activation of a dormant tumor suppressor gene maspin by designed transcription factors. Oncogene 2007;26:2791-8
- Falke D, Fisher M, Ye D, Juliano RL. Design of artificial transcription factors to selectively regulate the pro-apoptotic bax gene. Nucleic Acids Res 2003;31:e10
- Yokoi K, Zhang HS, Kachi S, et al. Gene transfer of an engineered zinc finger protein enhances the anti-angiogenic defense system. Mol Ther 2007;15:1917-23
- Rebar EJ, Huang Y, Hickey R, et al. Induction of angiogenesis in a mouse model using engineered transcription factors. Nat Med 2002;8:1427-32
- Bultmann S, Morbitzer R, Schmidt CS, et al. Targeted transcriptional activation of silent oct4 pluripotency gene by combining designer TALEs and inhibition of epigenetic modifiers. Nucleic Acids Res 2012;40:5368-77
- Gao X, Yang J, Tsang JC, et al. Reprogramming to pluripotency using designer TALE transcription factors targeting enhancers. Stem Cell Reports 2013;1:183-97