Abstract
Sobetirome binds selectively to the main hepatic form of thyroid hormone (TH) receptor, TRβ1, compared to TRα1, which is principally responsible for thyrotoxic effects on heart, muscle and bone. Sobetirome also preferentially accumulates in liver. It was originally envisaged that sobetirome could be used to stimulate hepatic pathways that lower cholesterol without harmful side effects and might be used in conjunction with statins. Indeed, sobetirome progressed through preclinical animal studies and Phase I human clinical trials with excellent results and without obvious harmful side effects. Despite the fact that cardiovascular disease remains a major cause of mortality and that new therapies are desperately needed, it is unlikely that sobetirome will progress in further human clinical trials in the near future. The emergence of alternative cholesterol-lowering therapeutics may render selective thyromimetics redundant. Further, fears of thyrotoxic effects in the heart and emergence of cartilage defects in dogs after long-term use of eprotirome, a similar though not identical compound, has reduced enthusiasm for this strategy. We argue that it is nevertheless important to explore uses of sobetirome in humans; more treatment strategies would help patients with hard-to-treat dyslipidemias. Sobetirome may also have additional applications in orphan indications and short-term controlled weight loss.
1. Introduction. Cardiovascular disease: a need for new drugs
Despite recent advances, cardiovascular disease remains the leading cause of death [Citation1]. Dyslipidemia, elevation of serum cholesterol and/or triglycerides, triggers atherosclerosis, ischemic heart disease, stroke and peripheral artery disease. Statins inhibit 3-hydroxy-3-methyl-glutaryl-CoA reductase, which catalyzes the rate-limiting step of the cholesterol synthetic pathway in liver [Citation2]. This, in turn, reduces hepatic cholesterol, leading to activation of low-density lipoprotein (LDL) receptor gene expression and increased reabsorption of LDL cholesterol. While statins have striking impacts on dyslipidemias and cardiovascular disease, there remains a need for new cholesterol-lowering drugs. Desired cholesterol reductions are not always achieved with statins. This may be due, in part, to genetic variations in dyslipidemias; for example, homozygous familial hypercholesterolemia (FH), caused by inherited defects in LDL receptor genes, causes severe statin-resistant hyperlipidemia and statin sensitivity differs among patients with heterozygous disease. Additionally, statins are not always tolerated, especially at high doses. Side effects include elevated serum liver enzymes, muscle pain, rhabdomyolysis, cognitive effects and increased risk of Type 2 diabetes [Citation2].
New options for management of dyslipidemias are emerging () [Citation3]. Niacin and ezetimibe, the latter of which inhibits absorption of cholesterol in the small intestine, have long been known to reduce serum cholesterol but also cause side effects that limit compliance. Newer therapeutics include monoclonal antibodies that inhibit proprotein convertase subtilisin/kexin type (PCSK)9 and block PCSK9-dependent LDL receptor degradation, thereby increasing LDL receptor levels. Three PCSK9 inhibitors are in final stages of Phase III human clinical trials and yield spectacular cholesterol reductions without obvious side effects. Moreover, one of these, evolocumab, reduced heart attack, stroke and heart failure rates by 50% over the course of a one year trial. An ATP citrate lyase (ACL) inhibitor (ETC-1002) reduces cholesterol levels by blocking supply of substrate for cholesterol biosynthesis and yielded significant cholesterol reductions without obvious side effects in trials that have lasted up to 12 weeks, with and without statins. In light of requirements for cholesterol-lowering therapies, it is nevertheless interesting to consider selective thyromimetics, developed as cholesterol therapeutics but largely discontinued for this purpose.
Figure 1. Schematic: Influences of cholesterol-lowering therapeutics on hepatic and extrahepatic cholesterol metabolism. The figure is a simplified depiction of cholesterol metabolism and flux in hepatocytes. Gray circles represent metabolites, rectangles represent proteins (purple = enzymes, blue = other proteins), triangles represent lipoprotein particles. Large pale gray arrows represent directions of cholesterol flux and metabolism. Small arrows at side of proteins represent changes in activity, either through alterations in protein levels or enzyme activity. Thyromimetics enhance low-density lipoprotein receptor (LDLR) expression, increase cholesterol to bile acid conversion by inducing expression of the cholesterol-processing enzyme cholesterol 7α-hydroxylase (Cyp7A1) and inhibit expression of the transcription factor sterol response element-binding protein (SREBP1c), which directs genes involved in triglyceride (TG) synthesis. Statins inhibit HMG-Co reductase with secondary effects on LDLR expression. Proprotein convertase subtilisin/kexin type 9 (PCSK9) inhibitors block PCSK9-dependent degradation of LDLR, thereby increasing LDLR levels. The ATP citrate lyase (ACL) inhibitor blocks cholesterol synthesis.
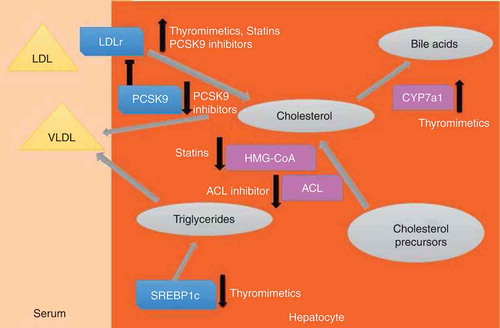
2. Thyromimetics as cholesterol-lowering therapeutics
2.1 Manipulation of thyroid hormone to reduce cholesterol
Rationale for selective modulation of thyroid hormone (TH) signaling has been reviewed extensively [Citation4-Citation6]. Excess TH leads to elevated heart rate, arrhythmias, bone and muscle catabolism and mood disturbances, but also reduces serum cholesterol and body fat. There are two TH receptors (TRs α and β) that are members of the nuclear hormone receptor family and encoded by different genes. Evidence from TR knockout mice, humans with TRβ mutations causing TH resistance and, latterly, patients with TRα mutations suggests that TRα is responsible for some harmful TH effects on heart, muscle and bone, whereas TRβ regulates serum cholesterol. Thus, TRβ-selective ligands could be useful for control of serum cholesterol. It is also desirable to target thyromimetics to liver, which mediates cholesterol synthesis and processing, while sparing tissues in which THs exert harmful effects. In this regard, cell-specific transporters regulate TH uptake and efflux and these proteins, along with intracellular TH-modifying enzymes, regulate TH availability at the pre-receptor level [Citation7]. Mechanisms of uptake and efflux of synthetic TRβ-directed analogs are not clear and sobetirome and related compounds are not substrates for intracellular deiodinases. Perhaps selective thyromimetics could exploit differential uptake/processing mechanisms to safely control cholesterol via effects on delivery of analogs to TRβ in appropriate tissues. However, it is also conceivable that synthetic thyromimetics could interfere with these processes in hard to predict ways that might lead to harmful side effects.
TRs influence multiple steps of hepatic cholesterol processing (). While TRs, like statins and PCSK9 inhibitors, induce LDL receptor, TR also induces cholesterol 7α-hydroxylase (Cyp7a1), which mediates the rate-limiting step of cholesterol to bile acid conversion and inhibits expression of sterol response element-binding protein (SREBP)1c, which regulates genes involved in hepatic triglyceride synthesis and export. Thus, thyromimetics could treat dyslipidemias that are resistant to therapeutics that work by increasing LDL receptor, such as statins, and could complement other cholesterol-lowering therapies with different mechanisms.
2.2 Sobetirome
Sobetirome (GC-1) was the first of a new class of thyromimetics that emerged in the 1990s [Citation4-Citation6]. GC-1 binds preferentially to TRβ versus TRα and accumulates selectively in liver. Sobetirome has been tested extensively in preclinical animal models, including rodents and primates, and reached Phase Ib human clinical trials. There was significant reduction in LDL cholesterol in all species and reductions in serum triglycerides and lipoprotein a [Lp(a)], linked to atherogenesis, in primates. Therapeutic doses of sobetirome did not elicit signs of thyrotoxicity, except for weak suppression of the hypothalamic pituitary (H-P-T) axis. Notably, sobetirome reduced LDL cholesterol in a mouse model of homozygous FH [Citation8], indicating that cholesterol-lowering actions do not absolutely require LDL receptor.
2.3 Eprotirome
Actions of sobetirome should be evaluated in light of studies of similar compounds, including eprotirome (KB2115) [Citation4]. Eprotirome is a thyromimetic that is highly liver selective and weakly TRβ selective and also reduces LDL cholesterol, TGs and Lp(a) in animals (). Eprotirome reduced total and LDL cholesterol by up to 40% in 14-day treatments of hypercholesterolemic human subjects and stimulated bile acid synthesis [Citation9], indicative of Cyp7a1 induction. A further 12-week trial of humans on statins revealed large reductions in LDL cholesterol, Lp(a) and triglycerides [Citation10]. Similar results were obtained in a 6-week study of patients with heterozygous FH and a 12-week study of patients with primary hypercholesterolemia [Citation11,Citation12]. There were modest transient elevations in liver enzymes in the latter two studies and no effects on heart, markers of bone catabolism or muscle strength although short treatment durations may mean that deleterious effects could emerge over longer times. Serum thyrotropin and triiodothyronine (T3), the active form of TH, were unaltered but there was a modest decrease in the parental TH, thyroxine (T4). This effect was attributed by authors to decreases in serum T4-binding globulin, which occur in hyperthyroidism, and hepatic induction of deiodinase 1, which converts T4 to T3 [Citation12]. This idea is unproven and other possibilities include conversion of T4 to glucorinidated or sulfated forms. It is interesting to note that one highly liver-selective member of a series of 1-benzyl-4-aminoindole-based TH analogs that partly resemble GC-1 (SKL-13784) reduces endogenous T4 levels without evidence for escape from this tissue [Citation13]. Further, this SKL-13784 effect requires lower doses than required for lipid lowering. This implies that T4 lowering involves hepatic actions that differ mechanistically from lipid-lowering actions.
Table 1. Comparison of actions of sobetirome and eprotirome.
Unfortunately, 12 – 15 month eprotirome treatments resulted in cartilage defects in dogs. This was unexpected; there is no evidence that TH disturbances cause similar effects in humans and no obvious explanation for how a highly liver-specific thyromimetic with only modest effects on serum TH disrupts cartilage [Citation12,Citation13]. It is unclear whether this effect is related to TRs or off-target effects and whether effects are drug or drug class specific. Nevertheless, this effect is concerning; THs exert multiple influences on skeleton and euthyroidism is crucial for normal skeletal development [Citation14]. Additionally, THs regulate articular cartilage maintenance and influence the etiology of osteoarthritis [Citation14]. It is therefore important to understand mechanisms of cartilage disrupting effects and to test long-term effects of all thyromimetics on skeleton and joints.
2.4 Alternative effects of sobetirome
Sobetirome displays additional useful actions. Sobetirome achieved orphan drug status for X-linked adrenoleukodystrophy [Citation15], caused by mutations in a membrane lipid transporter (ABCD1) and leading to toxic very long-chain fatty acid (VLCFA) accumulation in all cells and disease in the CNS and adrenal gland. Since THs induce a related gene (ABCD2), sobetirome could distribute to the CNS and induce ABCD2 to increase VLCFA uptake to mitigate disease effects. Sobetirome also safely reduces body fat via induction of thermogenesis [Citation4,Citation16]. These effects are accompanied by reduced blood glucose in mouse models of Type 2 diabetes and liver fat clearance [Citation11]. Mechanisms are likely extrahepatic actions not shared with the liver-selective compounds (). Finally, sobetirome displays pro-angiogenic actions that stem from non-genomic effects and, if reproducible in clinical settings, could be useful in patients with blood vessel disease [Citation17].
3. Conclusion
Sobetirome and related compounds are effective therapeutics which reduce LDL cholesterol with few side effects and sobetirome displays the potential to manage weight loss.
4. Expert opinion
It is unlikely that sobetirome will move further in human trials in the near future. Emergence of new cholesterol-lowering drugs could render the thyromimetics redundant. Fears of cardiovascular side effects, whether well founded or not, mean that large clinical trials will be needed to prove safety and discovery of eprotirome effects on dog cartilage has reduced enthusiasm. It may become important to reconsider sobetirome if PCSK9 and ACL inhibitors display unexpected side effects. There could also be applications of sobetirome and other thyromimetics in dyslipidemias that are resistant to strategies that increase LDL receptor, including homozygous FH. Here, it would only be possible to use sobetirome long term if concerns about cartilage disruption prove unfounded.
There is no evidence that short uses of sobetirome lead to harmful side effects and this could be useful. Clinical guidelines for patients in need of organ transplants rule out individuals who fail to meet weight limits. Here, managed weight loss, where patients are monitored for excessive increases in body temperature and other harmful actions, could literally become lifesaving. Exploration of actions of sobetirome in this area is highly warranted.
Declaration of interest
The authors were supported by the National Institutes of Health (NIH) under award number RC4 DK090849. The authors have no other relevant affiliations or financial involvement with any organization or entity with a financial interest in or financial conflict with the subject matter or materials discussed in the manuscript apart from those disclosed.
Notes
Bibliography
- FastStats. Available from: http://www.cdc.gov/nchs/fastats/leading-causes-of-death.htm
- Pisaniello AD, Scherer DJ, Kataoka Y, Nicholls SJ. Ongoing challenges for pharmacotherapy for dyslipidemia. Expert Opin Pharmacother 2015;16:347-56
- Ahn CH, Choi SH. New drugs for treating dyslipidemia: beyond statins. Diabetes Metab J 2015;39:87-94
References 2 and 3 are recent reviews of emerging therapies for dyslipidemia.
- Baxter JD, Webb P. Thyroid hormone mimetics: potential applications in atherosclerosis, obesity and type 2 diabetes. Nat Rev 2009;8:308-20
- Scanlan TS. Sobetirome: a case history of bench-to-clinic drug discovery and development. Heart Fail Rev 2010;15:177-82
State of the art review on Sobetirome action from the creator of the compound.
- Tancevski I, Demetz E, Eller P. Sobetirome: a selective thyromimetic for the treatment of dyslipidemia. Recent Pat Cardiovasc Drug Discov 2011;6:16-19
Good recent review on Sobetirome.
- Bernal J, Guadaño-Ferraz A, Morte B . Thyroid hormone transporters-functions and clinical implications. Nat Rev Endocrinol 2015;11:406-17
- Lin JZ, MartagÓn AJ , Hsueh WA, et al. Thyroid hormone receptor agonists reduce serum cholesterol independent of the LDL receptor. Endocrinology 2012;153:6136-44
Highlights the importance of non-low density lipoprotein (LDL) receptor dependent pathways in thyroid hormone (TH)-dependent cholesterol reabsorption; key for TH receptor (TR)-based therapies.
- Berkenstam A , Kristensen J, MellstrÖm K, et al. The thyroid hormone mimetic compound KB2115 lowers plasma LDL cholesterol and stimulates bile acid synthesis without cardiac effects in humans. Proc Natl Acad Sci USA 2008;105:663-7
- Ladenson PW, Kristensen JD, Ridgway EC, et al. Use of the thyroid hormone analogue eprotirome in statin-treated dyslipidemia. N Engl J Med 2010;362:906-16
- Sjouke B, Langslet G, Ceska R, et al. Eprotirome in patients with familial hypercholesterolaemia (the AKKA trial): a randomised, double-blind, placebo-controlled phase 3 study. Lancet Diabetes Endocrinol 2014;2:455-63
- Angelin B, Kristensen JD, Eriksson M, et al. Reductions in serum levels of LDL cholesterol, apolipoprotein B, triglycerides and lipoprotein(a) in hypercholesterolaemic patients treated with the liver-selective thyroid hormone receptor agonist eprotirome. J Intern Med 2015;277:331-42
- Takahashi N, Asano Y, Maeda K, Watanabe N. In vivo evaluation of 1-benzyl-4-aminoindole-based thyroid hormone receptor beta agonists: importance of liver selectivity in drug discovery. Biol Pharm Bull 2014;37:1103-8
- Williams GR. Thyroid hormone actions in cartilage and bone. Eur Thyroid J 2013;2:3-13
- Scanlan T, Hartley M, Placzek A, Righi M. Use of Sobetirome in the Treatment of X-linked Adrenoleukodystrophy. WO178892; 2014
- MartagÓn AJ , Lin JZ, Cimini SL, et al. The amelioration of hepatic steatosis by thyroid hormone receptor agonists is insufficient to restore insulin sensitivity in Ob/Ob mice. PLoS One 2015;10:e0122987
- Mousa SA, Lin HY, Tang HY, et al. Modulation of angiogenesis by thyroid hormone and hormone analogues: implications for cancer management. Angiogenesis 2014;17:463-9