Abstract
Liposomes are one of the effective drug delivery systems that are developed based on the nanotechnology concept. Liposomal formulation is the first nanomedicine approved by the US FDA for clinical application. Recently, the marketed liposomes and stealth liposomes have made impact for cancer therapy. In addition, a few receptor-targeted liposome products have been in different phases of clinical trials, which are yet to be marketed. In the present editorial, the advantages of vitamin E TPGS-coated liposomes over the currently available PEG-coated liposomes will be described and their great potentials for nanotheranostics for cancer imaging and therapy will be covered.
1. Introduction
Liposomes are one of the effective drug delivery systems that are developed based on the nanotechnology concept Citation[1]. Biocompatibility, biodegradability, fewer toxicity, nanosize and surface modifications comprise the utility of liposomes for effective drug delivery. Liposomal formulation is the first nanomedicine approved by the United States Food and Drug Administration (US FDA) for clinical application. Liposomes are vesicles consist of single or multiple concentric lipid bilayers (called lamellae), which were first prepared in the 1960s. Liposomes can be seen as the simplest artificial biological cells, which have great potential applications in drug delivery, gene therapy, vaccine delivery and molecular imaging Citation[1,2].
Liposomes may vary in size, most being 200 nm or less, which can be termed as ‘nanoliposomes' Citation[1]. Nanosized liposomes can be made of various natural lipids, and usually composed of phospholipids/cholesterol to encapsulate various active water-soluble drug in the hydrophilic core and/or insoluble drug in the hydrophobic membrane for targeted and controlled delivery of therapeutic agents for the enhancement of ADME (adsorption, distribution, metabolism and excretion) process to maximize efficacy with fewer adverse effects. Passive and active targeting can also be realized by enhanced permeability and retention (EPR) and targeting ligand conjugation. However, the major drawbacks of conventional liposomes are instability, insufficient drug loading, faster drug release and shorter circulation time in the blood. The liposomes will be eliminated by macrophages of the immune system. Therefore, the landmark in liposome development at the end of 1980s was the invention of stealth liposomes, that is, long circulating liposomes by PEGylation to increase the stability of the liposomes in the blood. PEGylation was originally defined as conjugation of a bioactive molecule or polymer to polyethylene glycol (PEG) to enhance its solubility, permeability and stability. PEGylation has now become one of the most sophisticated technologies in the pharmaceutical applications. It was suggested that by its excluded volume effect, the long PEG chain coated on the liposome surface prevents the adsorption of plasma protein on to the liposome surface and, as a result, effectively reduces the liposome aggregation in plasma. PEGylation is now extended to various nanocarriers such as micelles, liposomes and polymeric nanoparticles. PEGylation can avoid quick recognition and elimination of liposomes by the immune system and thus prolong the circulation of liposomes in the body. Also, stealth liposomes can passively accumulate in solid tumors due to their inherently leaky vasculature and defective lymphatic drainage Citation[3].
Recently, the marketed liposomes and stealth liposomes have made impact for cancer therapy. For example, Doxil®, Caelyx® and Myocet® are nanosized liposomes (encapsulated with doxorubicin in their aqueous core) which have been used for the treatments of Kaposi's sarcoma, ovarian cancer and multiple myeloma. In addition, a few receptor-targeted liposome products have been in different phases of clinical trials, which are yet to be marketed Citation[2]. An alternative form of development of PEGylation is surface modification or coating of liposomes with d-α-tocopheryl PEG 1000 succinate (vitamin E TPGS) Citation[3].
In the present editorial, the advantages of vitamin E TPGS-coated liposomes over the PEG-coated liposomes fabricated in the authors' lab (both in vitro and in vivo level) will be compared and their great potential success in pre-clinical level as nanotheranostics (e.g., bioluminescence evaluation) for the future cancer imaging and treatment will be covered.
2. Vitamin E TPGS-coated liposomes and their theranostic potential
Vitamin E TPGS or simply TPGS is a PEGylated vitamin E, which has greatly improved the pharmaceutical properties of vitamin E and thus has been widely applied in the food and drug industry. TPGS, prepared from the esterification of d-α-tocopheryl acid succinate and PEG 1000, is an amphiphilic vitamin E, quite stable under normal conditions without hydrolysis. Owing to its hydrophilic–liphophilic balance (HLB) value being between 15 and 19, TPGS has excellent water solubility and it is effective emulsifier which can emulsify hydrophobic molecules. The co-administration of TPGS has been shown to enhance the solubility, inhibit P-glycoprotein-mediated multi-drug resistance and increase the oral bioavailability of anti-cancer drugs Citation[4]. The authors have reported TPGS–doxorubicin conjugate as a novel prodrug which enhanced the therapeutic potential and reduced the systemic side effects of the drug. Additionally, they have studied TPGS as an emulsifier in the preparation of poly(d,l-lactide-co-glycolide) (PLGA) nanoparticles, and as a component of new biodegradable copolymer polylactide-TPGS (PLA-TPGS) for nanoparticle formulation of anti-cancer drugs Citation[5].
The pharmacokinetic results of TPGS-coated liposomes in rats (pre-clinical study) revealed that liposomes (based on the concentration of doxorubicin in plasma) have proved 24 h longer circulation time than PEG-coated liposomes Citation[6]. Further, the authors have prepared conventional, PEG- and TPGS-coated liposomes for comparison. The cellular uptake and in vitro cytotoxicity of the different liposomes were assessed on brain cancer cells. Results of TPGS-coated liposomes showed great advantages for in vitro cellular uptake and cytotoxicity than PEG-coated liposomes Citation[3]. Field emission transmission electron microscopy (FETEM) images also confirmed the presence of PEG and TPGS coating on the liposome surfaces (). In another study, the pharmacokinetic results of Herceptin receptor-targeted TPGS-coated liposomes in rats showed 21 h longer circulation time (based on the concentration of docetaxel in plasma) and better relative availability in comparison with PEG-coated liposomes (unpublished data).
Figure 1. Field-emission transmission electron microscope (FETEM) image of (A) an individual non-coated liposome in 50 nm scale, (B) an individual PEG-coated liposome also in 50 nm scale and (C) an individual TPGS-coated liposome in 200 nm scale.
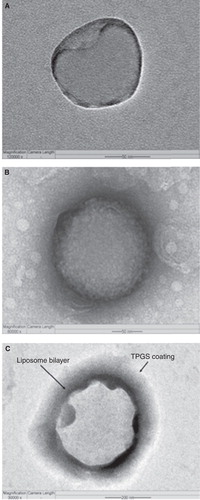
Theranostic liposomes are generally designed to facilitate simultaneous diagnosis and therapy of cancer Citation[7]. The nanosized imaging agent such as iron oxide, quantum dots and gold nanoparticles can be entrapped within the hydrophobic core or linked covalently to the surface of the theranostic liposomes and the anti-cancer agent can be either encapsulated in the core or embedded in the lipophilic bilayer shell. They are also called as ‘liposome–nanoparticle hybrids'. These theranostic liposomes can then be further conjugated with molecular probe for targeting. Such multifunctional liposomes may circulate for prolonged periods in the blood, evading host defenses, and gradually release drug by targeting and simultaneously facilitate in vitro or in vivo imaging. Combination of diagnosis with therapeutic intervention is more specific clinical protocols to individuals and likely to offer better prognosis and monitoring of disease. Currently, quantum dots have become a popular imaging agent used in the multifunctional nanoparticles as they have significant advantages over organic dyes. Furthermore, quantum dots emit highly intense signals, and they are photostable Citation[7,8]. For example, Yang et al. developed folate receptor-targeted liposomes with quantum dots loaded, and their targeted cellular imaging properties are reported for the future cancer imaging applications Citation[9].
Recently, Wen et al. evaluated brain targeting theranostic liposomes loaded with quantum dots and apomorphine. The formulation was evaluated for in vitro brain endothelial cell uptake and in vivo bioimaging. In vitro cellular uptake study confirmed the delivery of liposomes through receptor-mediated endocytosis routes. The fluorescence derived from quantum dots was visualized during bioimaging. A significant distribution was observed for theranostic liposomes compared with free quantum dots. The brain uptake was increased after the quantum dots were incorporated into liposomes. The fluorescence in the brain observed up to 1 h for the liposomal group. However, free quantum dots were rapidly eliminated from the brain and retained more in liver up to 35 min Citation[10]. This study suggested a feasibility of bioimaging in animal models using theranostic liposomes for cancer diagnosis and treatment during their pre-clinical development.
Grange et al. evaluated the combination of magnetic resonance imaging (MRI) and therapy of doxorubicin-loaded liposomes in a Kaposi's sarcoma model in vivo. MRI was studied to track the liposome tissue distribution and also to monitor drug release Citation[11]. In another study, Kostarelos and collaborators described a theranostic liposome loaded with cytotoxic drug (doxorubicin). They incorporated quantum dots into lipid layers, which made them dispersed in physiological conditions. Successful drug loading was confirmed by observation of doxorubicin crystals inside the hybrids. The cryo-transmission electron microscopy (cryo-TEM) and atomic force microscopy (AFM) of theranostic liposomes confirmed the incorporation of quantum dots in the liposomes bilayer. The theranostic liposomes were taken up by cells and were able to release drug intracellularly, as evidenced by a significant enhancement in the cytotoxicity Citation[8,12].
In another study, the authors have developed TPGS-coated, folic acid-conjugated theranostic liposomes (liposome-quantum dots hybrids) for targeted co-delivery of quantum dots and docetaxel Citation[13]. Liposomes are coated by vitamin E TPGS for long-circulation effects as well as for enhanced cellular uptake. The structural characterization by FETEM confirmed the incorporation of quantum dots in the liposome bilayer (). Fluorescent quantum dots-loaded multifunctional liposomes were studied for qualitative cellular uptake by folate receptor expressing MCF-7 cells. The red fluorescence in the MCF-7 cells, which corresponds to the targeting multifunctional liposomes was higher and showed more red stain in cytoplasm than that of the non-targeting liposomes. It confirmed the targeted cellular uptake of the multifunctional liposomes. In vitro cytotoxicity of folate receptor expressing MCF-7 cells was assessed and the IC50 value was assessed to evaluate the in vitro therapeutic effects of the multifunctional liposomes with or without targeting function. After 24 h incubation, IC50 for each of the three types of docetaxel formulation, liposome formulations versus Taxotere® were determined from the cytotoxicity data to be 1.56 ± 0.19, 0.23 ± 0.05 versus 9.54 ± 0.76 μg/ml, respectively, which imply that the drug formulated in the non-targeting liposomes and the targeting liposomes could be 83.64 and 97.58% more efficient than Taxotere after 24 h treatment, respectively Citation[13].
3. Conclusions
In this editorial, the development of theranostic liposomes (possibility of loading wide variety of diagnostic nanoparticles along with anti-cancer drugs) in combination with TPGS coating and their pre-clinical success were discussed. Also, the authors discussed the feasibility of bioluminescent (imaging) evaluation in animal models using theranostic liposomes for cancer diagnosis and treatment. In addition, theranostic liposomes can be modified with different targeting ligands on the surface to have binding specificity for cancer cell receptors for theranostic applications. Next generations of theranostic liposomes such as liposome–nanoparticle hybrids including stimulus responsive functions (e.g., pH, temperature, magnetism and ultrasound) for triggering drug release at targeted and controlled manner, have to be developed. In future, theranostic technology has to be integrated into successful platforms such as polymeric nanoparticles, gold nanomaterials, carbon nanotubes, silica nanoparticles and magnetic nanoparticles Citation[14].
4. Expert opinion
The research efforts are further needed to explore this concept based on the available clinical platform of liposomes in combination with novel diagnostic nanoparticles, toward the development of versatile and smart theranostic applications. The challenges in cancer chemotherapy are early cancer diagnosis, imaging tumor angiogenesis, tracking tumor metastasis, tracking intracellular delivery of anti-cancer drugs and monitoring of tumor therapy. Quantum dots-loaded theranostic liposomes could be potential carrier to address some of these issues in near future. Also, biocompatible and safer surface-coating material (may be TPGS) which could prevent the release of toxic heavy metals from the inorganic core of quantum dots is desirable for clinical use. Theranostic liposomes containing quantum dots with the capacity of multimodality imaging (i.e., combination of MRI and fluorescence) could be advantageous for theranostic purposes Citation[5]. The use of heavy metal-based quantum dots as theranostic probes for human use is a major concern at present. It is a challenge to study the long-term toxic effects of quantum dots in animal systems that could potentially mimic its toxicity in human use Citation[15]. In future, fabrication of biocompatible, non-immunogenic, ultra-small size (< 5.0 nm) quantum dots for liposomes loading that would be excreted through a renal clearance mechanism is highly awaited.
Declaration of interest
MS Muthu acknowledges the Department of Science and Technology (DST), New Delhi, India, for the BOYSCAST Fellowship (SR/BY/L-41/2009) and research project under Fast Track Scheme for young scientists (SERB/FT/LS-132/2011).
Notes
Bibliography
- Muthu MS, Feng SS. Nanopharmacology of liposomes developed for cancer therapy. Nanomedicine (Lond.) 2010;5:1017-19
- Muthu MS, Singh S. Targeted nanomedicines: effective treatment modalities for cancer, AIDS and brain disorders. Nanomedicine (Lond) 2009;4:105-18
- Muthu MS, Kulkarni SA, Xiong J, Vitamin E TPGS coated liposomes enhanced cellular uptake and cytotoxicity of docetaxel in brain cancer cells. Int J Pharm 2011;42:332-40
- Feng SS. New-concept chemotherapy by nanoparticles of biodegradable polymers: where are we now? Nanomedicine (Lond) 2006;1:297-309
- Zhang Z, Tan S, Feng SS. Vitamin E TPGS as a molecular biomaterial for drug delivery. Biomaterials 2012;33:4889-906
- Wang AJ, Wang PL, Lu SJ. Long circulating liposome. US0142182; 2005
- Choi KY, Liu G, Lee S, Theranostic platforms for simultaneous cancer imaging and therapy: current approaches and future perspectives. Nanoscale 2012;4:330-42
- Al-Jamal WT, Kostarelos K. Liposomes: from a clinically established drug delivery system to a nanoparticle platform for theranostic nanomedicine. Acc Chem Res 2011;44:1094-104
- Yang C, Ding N, Xu Y, Folate receptor–targeted quantum dot liposomes as florescence probes. J Drug Target 2009;17:502-11
- Wen CJ, Zhang LW, Al-Suwayeh SA, Theranostic liposomes loaded with quantum dots and apomorphine for brain targeting and bioimaging. Int J Nanomedicine 2012;7:1599-611
- Grange C, Geninatti-Crich S, Esposito G, Combined delivery and magnetic resonance imaging of neural cell adhesion molecule-targeted doxorubicin-containing liposomes in experimentally induced Kaposi's sarcoma. Cancer Res 2010;70:2180-90
- Al-Jamal WT, Al-Jamal KT, Tian B, Lipid-quantum dot bilayer vesicles enhance tumor cell uptake and retention in vitro and in vivo. ACS Nano 2008;2:408-18
- Muthu MS, Kulkarni SA, Raju A, Theranostic liposomes of TPGS coating for targeted co delivery of docetaxel and quantum dots. Biomaterials 2012;33:3494-501
- Mukerjee A, Ranjan AP, Vishwanatha JK. Combinatorial nanoparticles for cancer diagnosis and therapy. Curr Med Chem 2012;19:3714-21
- Santra S. The potential clinical impact of quantum dots. Nanomedicine (Lond.) 2012;7:623-6