A priori, it would seem logical to use targeted delivery to enhance the effectiveness of a mucosal vaccine. This notion is based in part on observations that selective capture of microbes by M cell surface receptors can help drive transport of antigen into lymphoid tissues and induce protective mucosal immune responses such as secretory IgA. However, the basic receptor capture principle alone might not be sufficient to drive mucosal vaccines to clinical practice; indeed, synthetic mucosal vaccines have not yet become part of the mainstream vaccine armamentarium. Therefore, it may be appropriate to re-examine the criteria for evaluating mucosal vaccine delivery and the strategies used for developing effective delivery systems. Taking into account the dynamics of the mucosal lumen, additional approaches may be incorporated into existing vaccine development strategies. Here, we consider the potential for examining forces such as long-range electrostatic interactions.
It is estimated that most infectious diseases enter the body through mucosal routes, so the development of effective mucosal vaccines is an important medical goal. Vaccines against mucosal pathogens fall into two general categories. The first category is live attenuated organisms, such as attenuated poliovirus, rotavirus, or cold-adapted influenza, or attenuated bacteria such as Salmonella typhi. The induction of immunity is dependent on the ability of the live organism to resemble pathogenic infection, and trigger protective immunity. In the case of mucosal infections, this immune response includes both T cell responses as well as serum IgG and mucosal IgA. This discussion will focus mainly on mucosal IgA responses; a fuller discussion of mucosal vaccine induction of cellular immunity is published elsewhere Citation[1].
While live attenuated vaccines are useful, they are not easily developed for all mucosal pathogens, so a second category includes killed microbes, extracts, and recombinant forms of microbial antigens. For example, killed versions of influenza and polio, and purified Salmonella typhi polysaccharide have been in use for decades. However, in contrast to live attenuated vaccines that are given orally, these killed or extracted forms of vaccines are injected into non-mucosal tissues (e.g., intramuscular). While this avoids the potential risks of administering live potentially pathogenic microbes, it also has the disadvantage that it mainly induces a central IgG immune response and no mucosal immunity. That is, while serum IgG can provide some protection, it is not as potent at mucosal surfaces as secretory IgA. Thus, there remains a need for a mucosal vaccination technology that can effectively induce protective mucosal immunity.
Mucosal lymphoid tissues such as intestinal Peyer's patches and tonsils are sites for the induction and development of mucosal immunity, especially secretory IgA Citation[2-5]. So it would seem that the best strategy for mucosal immunization would be to target the delivery of vaccine antigens to these lymphoid tissues Citation[6,7]. The entry route to these tissues is known to be through the specialized epithelial M cells, which can capture large (1 to 10 micron) particles and transcytose them to the underlying lymphoid tissues where antigen presenting cells pick up the delivered package and trigger the sequence of events leading to mucosal immune responses. Indeed, mucosal pathogens appear to have figured this out ages ago, as many pathogens, including Yersinia, HIV, Salmonella, Listeria, Shigella, Brucella, Reovirus, and Anthrax are already known to rely on M cell uptake for entry into the body Citation[8-15].
M cell-targeting strategies have been tested by several groups on the notion that targeting the M cell would deliver antigen far more efficiently than bulk ingestion of antigen, though in some cases this has also been used to induce tolerance rather than immunity Citation[16-21]. From an efficiency perspective, focused delivery would seem at first blush to be a better approach. Thus, the principle of M cell targeting has shown some efficacy in mouse models; quantitative studies using fluorescently labeled particles showed specific uptake by M cells of particles displaying short targeting peptides Citation[22,23]. More importantly, vaccine antigens attached to M cell-targeting ligands induced enhanced mucosal IgA responses when given intranasally Citation[17,24]. An added benefit is that mucosal immunization also induces strong central serum antibody responses that are quite efficient on a per-dose basis.
While this appears to be a promising strategy, the biology of the mucosal tissue has not been fully taken into account. If we were to impute the teleological goal of mucosal immune surveillance, it would probably be best described as efficient sampling of mucosal lumenal contents, rather than quantitative capture of specific particles. By this view, normal biology seems to conflict with the goals of mucosal vaccination, which aims to achieve quantitative delivery of vaccine antigen through M cells to mucosal lymphoid tissues. While a minimum dose might be considered sufficient (“good enough”) to induce protective immunity, for reliable administration among clinical populations a clear predictability in dose delivery is required.
Is such quantitative delivery achievable? In the case of oral/intestinal delivery, the anatomy of the intestinal lumen suggests that particles or vaccine antigens suspended in the lumenal fluids are not efficiently brought to the M cell apical membrane. Small soluble proteins diffuse efficiently in the lumenal fluids, but they are susceptible to digestion, and so may be considered unsuitable formulations. Vaccine formulations based on microparticles of encapsulated antigen diffuse far less efficiently; moreover, they will be affected by any material already present in the lumen and the overall effects of bulk movement and peristalsis. Intranasal delivery avoids the problem of protein degradation, but quantitative delivery remains a potential problem, as there is a practical limit to the maximum volume of a vaccine suspension that can be given, and most of the volume will end up in the stomach.
Despite these concerns, there are still ways in which the biology of M cells may inspire new technological solutions to mucosal vaccine delivery. As discussed above, M cells have the unique ability to take up large particles, compared with most receptor-mediated endocytosis, which commonly involves endosomes in the range of only 50 nanometers in diameter. We would argue that this is a clue to additional mechanisms used by M cells. The argument goes as follows: although many pathogens bind receptors on M cells, actual protein–protein high affinity binding interactions occur over very close distances, across a few nanometers. So how do ligands on large microbial particles in the mucosal lumen find their targets? Particles in suspension are quite unlike small highly diffusable molecules, and unlike tethered trans-membrane proteins, they are unable to take advantage of local contact during cell–cell interactions.
Particles in suspension can interact with surfaces (i.e., mucosal epithelium) through additional forces, including short-range van der Waals interactions (several nanometers) and longer-range electrostatic interactions (potentially up to hundreds of nanometers?). Electrostatic interactions include charge repulsion, as between objects with similar charge, or electrostatic attraction, as with objects of opposite charge. In general, mammalian proteins and cells are negatively charged, and most microbes also have a net negative charge; thus, interactions between microbes and epithelium should show electrostatic repulsion Citation[23]. However, it is also clear that in mucosal tissues, the apical membranes of M cells are quite distinct from neighboring epithelial cells. Bronchial epithelium and intestinal enterocytes both present highly complex and elaborate apical membrane surfaces (ciliated airway epithelium and intestinal brush border microvilli). Indeed, the name for M cells was based on the microfold appearance of their apical membrane.
Other components of the epithelial barrier will have an influence on particle access. For example, glycoproteins at the apical membrane of intestinal epithelium contribute a network of carbohydrates known as the glycocalyx. Electron microscopy studies of this network Citation[25] suggested that the depth of the glycocalyx may contribute to the epithelial barrier function. In the case of Peyer's patch M cells, the glycocalyx was found to be considerably thinner and far less organized than that overlying conventional villous epithelium. Unfortunately, the Caco-2BBe cell line used for M cell modeling produced only a minimal glycocalyx, so in vitro studies to directly correlate glycocalyx electrostatic charge and particle interaction were not possible. Another important component of the epithelial barrier is intestinal mucus, which appears to be thinner above the Peyer's patch epithelium. Studies on cervical mucus Citation[26,27] suggest that while mucus fibers might trap particles through charge interactions and thus help clear them, gaps in the mucus fiber network may enable particle access to epithelium. So while the mucus barrier may be an important consideration in M cell delivery mechanisms, it needs to be clarified whether specific electrostatic charge interactions described for cervical mucus can be applied equally well to intestinal mucus.
Based on these considerations, we suspect that the unique apical surface affects its interaction with particles in suspension. There is indirect evidence to support this hypothesis from studies on M cell-dependent uptake of intranasally administered microparticles displaying an M cell-targeting peptide. These studies examined the effects of ionic strength on uptake; at lower ionic strength, electrostatic interactions are increased, since there are fewer ions in solution to shield the interactions between particles and epithelium. While this might be predicted to increase electrostatic repulsion between negatively charged particles and epithelium, what was found instead was a paradoxical increase in M cell uptake compared to suspensions at higher ionic strength Citation[23]. Interestingly, at very low ionic strength, the effect on quantitative particle uptake was as strong as the effect of an M cell-targeting ligand. These results suggest that additional effects, possibly related to electrostatic interactions, are exploited by M cells to capture particles in suspension (depicted in ). Thus, new delivery technologies may be developed to take advantage of these multiple forces operating at the M cell apical membrane. Some steps in this direction have already been made Citation[28,29]; indeed, some synthetic particles can induce immunity when administered by the mucosal route. Unfortunately, while some of these may indeed be through enhanced M cell interactions Citation[16], many of them may instead have only enhanced penetration of villous epithelium. Clearing up this confusion will require clear quantitative tests of M cell-dependent uptake.
Figure 1. Hypothetical schematic of interactions between particles in suspension and mucosal epithelium. The figure illustrates the differences between short-range targeting interactions and long-range electrostatic particle interactions. Note that distances are for illustration, and do not accurately account for the thickness of the mucus and glycocalyx layers, and the height of microvilli, which are several hundred nanometers tall.
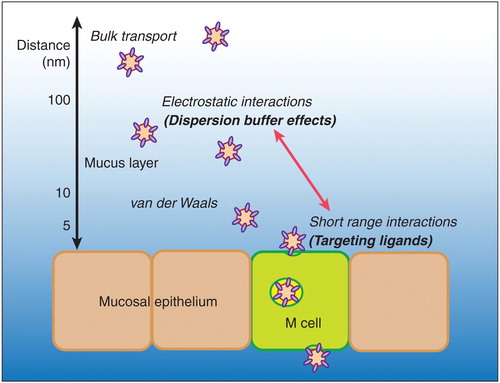
Expert opinion and conclusion
Mucosal vaccination is likely to be the best approach to inducing both protective IgA responses and central IgG responses, but more information is needed on the biology and kinetics of the response to mucosal vaccination. Although M cell-targeted vaccine delivery is a worthy aim, efficient quantitative delivery is probably not achievable given the physiology of the mucosal tissues. That is, most vaccine will pass through the system, making accurate dosing a major challenge. Targeting ligands only work at very short distances, so effective targeted delivery will have to include more comprehensive studies on the forces affecting particles in suspension in mucosal tissues.
Much work remains. It is clear that we need to clearly identify the specific measurable goals of mucosal vaccination Citation[1]; quantitative delivery of vaccine to target lymphoid tissues may need novel measurement standards, or at least different target goals. The effects of mucosal infection/inflammation, uptake in the presence of lumenal components such as food, and differences in mucosal microbiota will all have influences on mucosal vaccination, and it is not yet clear how to take these into account. In sum, it should be evident that we have not yet exhausted the possibilities for enhancing mucosal vaccine delivery, as we are still identifying new challenges to understanding the mechanisms of mucosal immune surveillance.
Declaration of interest
The authors state no conflict of interest and have received no payment in preparation of this manuscript.
Bibliography
- Woodrow KA, Bennett KM, Lo DD. Mucosal vaccine design and delivery. Annu Rev Biomed Eng 2012;14:17-46
- Asanuma H, Aizawa C, Kurata T, Tamura S. IgA antibody-forming cell responses in the nasal-associated lymphoid tissue of mice vaccinated by intranasal, intravenous and/or subcutaneous administration. Vaccine 1998;16:1257-62
- Craig SW, Cebra JJ. Peyer's patches: an enriched source of precursors for IgA-producing immunocytes in the rabbit. J. Exp Med 1971;134:188-200
- Wu HY, Nguyen HH, Russell MW. Nasal lymphoid tissue (NALT) as a mucosal immune inductive site. Scand J Immunol 1997;46:506-13
- Shikina T, Hiroi T, Iwatani K, IgA class switch occurs in the organized nasopharynx- and gut-associated lymphoid tissue, but not in the diffuse lamina propria of airways and gut. J Immunol 2004;172:6259-64
- Neutra MR, Frey A, Kraehenbuhl JP. Epithelial M cells: gateways for mucosal infection and immunization. Cell 1996;86:345-8
- Neutra MR, Kozlowski PA. Mucosal vaccines: the promise and the challenge. Nat Rev Immunol 2006;6:148-58
- Clark MA, Hirst BH, Jepson MA. M-cell surface beta1 integrin expression and invasin-mediated targeting of Yersinia pseudotuberculosis to mouse Peyer's patch M cells. Infect Immun 1998;66:1237-43
- Fotopoulos G, Harari A, Michetti P, Transepithelial transport of HIV-1 by M cells is receptor-mediated. Proc Natl Acad Sci USA 2002;99:9410-14
- Hase K, Kawano K, Nochi T, Uptake through glycoprotein 2 of FimH(+) bacteria by M cells initiates mucosal immune response. Nature 2009;462:226-30
- Jensen VB, Harty JT, Jones BD. Interactions of the invasive pathogens Salmonella typhimurium, Listeria monocytogenes, and Shigella flexneri with M cells and murine Peyer's patches. Infect Immun 1998;66:3758-66
- Martinoli C, Chiavelli A, Rescigno M. Entry route of Salmonella typhimurium directs the type of induced immune response. Immunity 2007;27:975-84
- Nakato G, Hase K, Suzuki M, Cutting edge: Brucella abortus exploits a cellular prion protein on intestinal M cells as an invasive receptor. J Immunol 2012;189:1540-4
- Wolf JL, Rubin DH, Finberg R, Intestinal M cells: a pathway for entry of reovirus into the host. Science 1981;212:471-2
- Glomski IJ, Piris-Gimenez A, Huerre M, Primary involvement of pharynx and peyer's patch in inhalational and intestinal anthrax. PLoS Pathog 2007;3:e76
- Garinot M, Fiévez V, Pourcelle V, Gylated PLGA-based nanoparticles targeting M cells for oral vaccination. J Control Release 2007;120:195-204
- Nochi T, Yuki Y, Matsumura A, A novel M cell-specific carbohydrate-targeted mucosal vaccine effectively induces antigen-specific immune responses. J Exp Med 2007;204:2789-96
- Rynda A, Maddaloni M, Mierzejewska D, Low-dose tolerance is mediated by the microfold cell ligand, reovirus protein sigma1. J Immunol 2008;180:5187-200
- Suzuki H, Sekine S, Kataoka K, Ovalbumin-protein sigma 1 M-cell targeting facilitates oral tolerance with reduction of antigen-specific CD4+ T cells. Gastroenterology 2008;135:917-25
- Wang X, Kochetkova I, Haddad A, Transgene vaccination using Ulex europaeus agglutinin I (UEA-I) for targeted mucosal immunization against HIV-1 envelope. Vaccine 2005;23:3836-42
- Wu Y, Wang X, Csencsits KL, M cell-targeted DNA vaccination. Proc Natl Acad Sci USA 2001;98:9318-23
- Rajapaksa TE, Stover-Hamer M, Fernandez X, Claudin 4-targeted protein incorporated into PLGA nanoparticles can mediate M cell targeted delivery. J Control Release 2010;142:196-205
- Rajapaksa TE, Bennett KM, Hamer M, Intranasal M cell uptake of nanoparticles is independently influenced by targeting ligands and buffer ionic strength. J Biol Chem 2010;285:23739-46
- Lo DD, Ling J, Eckelhoefer AH. M cell targeting by a Claudin 4 targeting peptide can enhance mucosal IgA responses. BMC Biotechnol 2012;12:7
- Frey A, Giannasca KT, Weltzin R, Role of the glycocalyx in regulating access of microparticles to apical plasma membranes of intestinal epithelial cells: implications for microbial attachment and oral vaccine targeting. J Exp Med 1996;184:1045-59
- Lai SK, O'Hanlon DE, Harrold S, Rapid transport of large polymeric nanoparticles in fresh undiluted human mucus. Proc Natl Acad Sci USA 2007;104:1482-7
- Lai SK, Wang Y-Y, Hida K, Nanoparticles reveal that human cervicovaginal mucus is riddled with pores larger than viruses. Proc Natl Acad Sci USA 2010;107:598-603
- Shakweh M, Ponchel G, Fattal E. Particle uptake by Peyer's patches: a pathway for drug and vaccine delivery. Expert Opin Drug Deliv 2004;1:141-63
- Shakweh M, Besnard M, Nicolas V, Fattal E. Poly (lactide-co-glycolide) particles of different physicochemical properties and their uptake by peyer's patches in mice. Eur J Pharm Biopharm 2005;61:1-13