1. Introduction
The research community has witnessed a resurgence over the last several years in the enthusiasm for phenotypic screening as an approach for small-molecule discovery. In the course of running screening campaigns, special attention must be paid to the means by which compounds are identified and advanced. Most efforts in the last 10–20 years have focused on target-based discoveries, in which disease modeling and pathway analysis lead to candidate proteins, the modulation of which may lead to disease-modifying therapies. Phenotype-based screening, however, does not rely upon specific hypotheses, but rather takes an unbiased approach to finding compounds that modify a particular cellular outcome of interest, usually in cell culture, but sometimes in whole organisms, such as zebrafish or C. elegans. Further, this approach can be useful to identify new targets for a disease, by repurposing existing drugs or by uncovering new mechanisms by which to achieve a desired phenotype. In reality, phenotypic screening was the primary mechanism of drug discovery for decades before the development of genomics-enabled target selection,[Citation1] so this revival of interest in phenotypic screening is really a return to the past.
2. Phenotypic discoveries in drug development
A highly influential review investigated the means by which drugs were discovered between 1999 and 2008.[Citation2] Of the 50 first-in-class new molecular entities (NMEs) approved for clinical use, 28 (56%) were discovered using a phenotypic approach, while 17 (34%) were target-based discoveries. Those frequencies were reversed when examining follower drugs: 83 of 164 drugs (50%) were found in a target-based manner, while only 30 (18%) were discovered by phenotypic approaches. The cancer field has had more success in taking target-based approaches, especially with regard to kinase inhibitors. Between 1999 and 2013, 31 of the 48 oncology NMEs were discovered by target-based approaches, and 21 of those NMEs were kinase inhibitors.[Citation3] The paucity of historic phenotypic discoveries is likely due to past limitations in phenotypic readouts in cancer (usually cell viability) and in good disease models that recapitulate human biology in cell culture. In fact, the second-generation thalidomides, such as lenalidomide, are thought to be the only example in oncology where the primary discovery and next-generation development were phenotypic in nature. As assay development parameters continue to be improved, it is likely that cancer drug discovery will encounter the same trends involving phenotype-based screening and discovery.
The development of powerful genomic technologies made target-based approaches very attractive, particularly in the discovery of antibacterial drugs. In a tour-de-force effort to interrogate essential genes in a variety of bacterial strains, a group from GlaxoSmithKline ran 70 target-based high-throughput screens against key targets. For the vast majority of screens, no hits were identified, but even when hits and a few optimized leads were identified, most of the compounds had no activity against the bacteria themselves.[Citation4] Overall, this campaign resulted in only five leads, a low success rate that is unsustainable over long-term drug discovery. In contrast, phenotypic approaches have become quite systematic in the malaria field,[Citation5] where drug resistance has become a huge problem in developing effective therapies.
A particularly attractive reason for the overall resurgence in phenotypic screening is the development of increasingly sophisticated cell models and assay readouts. The older models of genetic reporter assays in generic cell lines have given way to highly selective readouts carried out in patient-derived samples. A recent recommendation of a phenotypic ‘rule of 3’ (reminiscent of the chemical ‘rule of 5’) includes (1) highly disease-relevant assay systems, (2) disease relevance of the cell stimulus (e.g. do not use a reagent like hydrogen peroxide to model oxidative stress), and (3) assay readouts that are close to the clinically desired outcome.[Citation6] Each of these components will help increase the likelihood of clinical applicability; examples of success have included correction of mutations in the cystic fibrosis transmembrane conductance regulator (CFTR) [Citation7] and the development of potential single-cure malaria treatments.[Citation8]
3. The importance of mechanism-of-action studies
Despite the apparent advantage, and relative ease, of running phenotypic assays, a key factor preventing even greater use has been the difficulty in determining the mechanism of action (MoA) for validated screening hits. An important consideration here is the definition of MoA; although several researchers view this activity solely as the identification of compound targets, MoA can be gleaned at the level of the molecule, the biochemical pathway, the cell, or the whole organism.[Citation9] Perhaps surprisingly, detailed knowledge about small-molecule MoA is not strictly required for FDA approval; of the 28 NMEs discovered between 1999 and 2008 by phenotypic approaches, nine of them still had unknown MoA.[Citation2] In this regard, the standards required for claiming that a small molecule is a suitable chemical probe [Citation10] are actually more stringent than those required for drug development. Although it has been proposed that almost 20% of drugs have an unclear MoA, cheminformatic approaches, such as similarity ensemble approach (SEA), suggest that targets may be inferred, and that the number may be closer to 4–5%.[Citation11]
As detection technologies have improved, the ability to tackle MoA in a systematic manner has become more feasible.[Citation12,Citation13] Historically, affinity-based approaches have relied upon attaching a compound to solid support for cellular target pull-down, and has been successful for several MoA projects. More focused quantitative proteomics can also be used to evaluate, for example, ubiquitinated proteins, as was done to uncover the MoA of lenalidomide.[Citation14] Intriguingly, label-free approaches that rely on the cellular thermal stability of protein targets have great potential to provide information about a compound’s activity.[Citation15]
Beyond proteomics approaches, cell-based methods to understand MoA have included inferential methods, based on a reference set of compound treatments. For example, gene-expression analysis, as exemplified by a yeast compendia [Citation16] and connectivity map profiling,[Citation17] enables a comparison of unknown compounds with a bioactive set for similarity searching. Cell painting [Citation18] also takes a similarity approach, instead using cell morphology for compound comparison. More sophisticated computational analyses have evolved to take an assay-agnostic approach to inference generation. For example, high-throughput screening fingerprints [Citation19] and assay-performance profiling [Citation20] each take advantage of vast historical screening data available at Novartis and the Broad Institute, respectively, to create similarity networks between compounds, even when they have not been run on the same assays.
The selection of compound-resistance mutants, followed by genome sequencing, has been used frequently by the infectious disease community to find direct targets of compounds.[Citation21] Expanding to mammalian systems, modifier screening using shRNA or CRISPR libraries [Citation22] look for genetic knockdowns that reduce or enhance compound action to uncover compound activity. For any MoA effort, the application of multiple approaches is not only more likely to result in success, but also practically required for understanding the mechanism of a new compound. Each of these techniques, as well as a number of others, has helped embolden the scientific community in taking phenotypic approaches to small-molecule discovery.
4. Moving forward with cell profiling technologies
Cell profiling takes advantage of advances in high-throughput multidimensional detection methods to create large-scale maps between cellular parameters and small-molecule responses (). Researchers are likely most familiar with this approach in the context of cancer biology.[Citation23] Here, tens to hundreds of genomically characterized cells are profiled against tens to hundreds of well-annotated small molecules. Correlation analysis then enables the identification of new cancer dependencies, novel targets for intervention, or prediction of combination therapies.
Figure 1. Profiling technologies: an exercise in balancing throughput and dimensionality. Two-dimensional plot showing various profiling technologies according to their dimensionality (number of measurements that can be made at one time) and throughput (number of compounds that can feasibly be tested at one time).
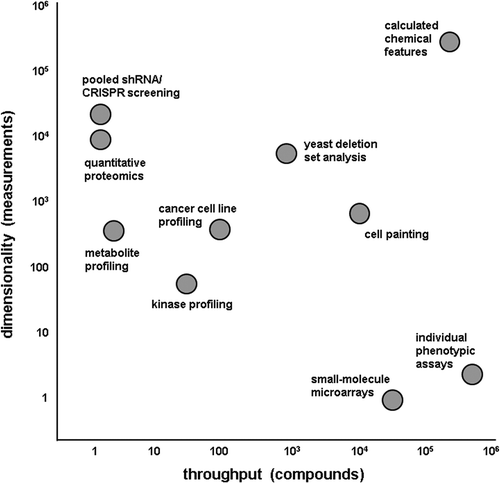
Many other profiling technologies are available (), with a major consideration being the balance between the number of compounds to be tested and the dimensionality of the throughput. Proteomics approaches, like kinase profiling and genetic suppressor screening, measure many effects, but on few compounds at a time. Individual phenotypic assays, on the other hand, measure a few effects across many compounds. Ideally, a high-throughput, high-dimensional readout would enable an ‘all versus all’ comparison at once; currently, computational approaches to calculate chemical features can achieve that level of dimensionality, but cell-based approaches are starting to move toward that quadrant of the graph ().
5. Conclusion
The increasing ability to develop physiologically relevant cell models and readouts has helped fuel a renewed zeal for making phenotypic measurements. In parallel, sophisticated detection technologies have helped make MoA determination more straightforward, imbuing phenotypic screening with far less uncertainty than in earlier days, where cell death in a single cell line may be the only phenotype under measurement. Further, the implementation of large-scale profiling techniques, and the computational methods that enable their analysis, has expanded the capacity to take a systems-level perspective toward small-molecule phenotypes. As a result, probe and drug discovery efforts have seen a growing reliance on unbiased phenotypic screening; these three capabilities have made the allure and productivity of this strategy possible.
6. Expert opinion
Phenotypic screening has received quite a bit of attention, and has become an increasingly popular approach, due to recent analyses showing that more NMEs have been discovered using phenotypic assays.[Citation2] It is possible that, in some disease biology areas, the low-hanging fruit for target selection and validation has been exhausted, and that new mechanisms for observing cellular phenotypes of interest need to be discovered. Further, using animal models of disease sets limitations on the translation of biological discoveries to human disease. It has been thought that the use of human ‘experiments of nature’ to identify new screening targets, by focusing on human genetic loci either enriched or depleted in disease, may be a more effective approach for drug discovery.[Citation24] Nonetheless, the discovery of small molecules that induce a desired phenotype provides direct evidence of efficacy toward a model of disease, regardless of the intracellular target. Key weaknesses of this approach have been the historical use of relatively crude assay readouts (such as ATP levels as a proxy for cell viability), and cell models inadequate to accurately portray the biology being interrogated. Recent recognition of these drawbacks,[Citation6] combined with advances in cell culture and assay technologies, have helped address these weaknesses.
The combination of sophisticated phenotypic screening and MoA studies enables our knowledge of newly discovered small molecules, predictive toxicology, repurposing of approved drugs for new indications, pharmacodynamics, and polypharmacology. Ultimately, a great advantage in taking a phenotypic approach is that discoveries are thought to have a greater likelihood of success in more advanced stages of drug development. The careful optimization of highly targeted small molecules, only to see them fail to induce a phenotype in cells, or to see them cause unanticipated toxicities, advises caution in taking such an approach. Indeed, that might be a critical reason why so many follower drugs are discovered by a target-based approach: existing drugs, by virtue of their clinical use, lend validation to those human targets, and decrease the risk in developing new small molecules toward these targets. However, such an approach fails to leverage the vast biology of the cell and the organism that we do not yet understand. Phenotypic screening, on the other hand, lets the cell reveal the chemical biology necessary to induce a phenotype, and thus not only improves chances of success, but also contributes to our corpus of biology knowledge.
Much work still remains to be done in developing sophisticated cell models for phenotypic screening. Nevertheless, entire fields have now been opened up for drug discovery based on these approaches. For example, neurodegenerative diseases have been refractory to small-molecule discovery, because not only were few targets known for intervention, but cell models were severely lacking. Advances in reprogramming and genome editing have enabled, for example, stem cell-based approaches to assay development.[Citation25] Further, it is likely that single targets will be inadequate to effect cellular change, so phenotypic screening increases the probability of success in this field.[Citation26] Even better than individual cell models is the development of organ-based models that can be screened in a high-throughput manner.[Citation27,Citation28] These types of advances will allow more disease areas, historically intractable to phenotypic screening, to be accessed for drug discovery.
In the interest of saving costs from late-stage clinical failures, it would seem attractive for the pharmaceutical industry to take phenotypic approaches more frequently during screening campaigns. For example, as cellular models of human toxicology develop and improve, these types of assays may even be used as secondary screens to prioritize compounds at a very early stage of the project. In the limit, one might envision a screening collection being profiled for activity up-front, establishing a ‘look-up table’ for data mining before screening even occurs. Again, the major barrier to these approaches has been understanding MoA following initial discovery. As MoA technologies continue to improve, that hesitation should steadily be alleviated. In that vein, label-free approaches to MoA are particularly attractive, as they do not require chemical modification (and verification of activity) for candidate compounds. Together, phenotypic screening and MoA studies should help increase the rate and improve the chance of success in drug discovery.
Declaration of interest
BK Wagner is supported in part by the Juvenile Diabetes Research Foundation Ltd (JDRF). She has no other relevant affiliations or financial involvement with any organization or entity with a financial interest in or financial conflict with the subject matter or materials discussed in the manuscript apart from those disclosed.
ORCID
Bridget K. Wagner http://orcid.org/0000-0002-2629-361X
References
- Papers of special note have been highlighted as either of interest (•) or of considerable interest (••) to readers.
- Zheng W, Thorne N, McKew JC. Phenotypic screens as a renewed approach for drug discovery. Drug Discov Today. 2013;18:1067–1073.
- Swinney DC, Anthony J. How were new medicines discovered? Nat Rev Drug Discov. 2011;10:507–519.
••This important article presents a comprehensive analysis of drug discovery during the 2000s, and makes a case for the importance of phenotype-based discoveries
- Moffatt JG, Rudolph J, Bailey D. Phenotypic screening in cancer drug discovery – past, present and future. Nat Rev Drug Discov. 2014;13:588–602.
- Payne DJ, Gwynn MN, Holmes DJ, et al. Drugs for bad bugs: confronting the challenges of antibacterial discovery. Nat Rev Drug Discov. 2007;6:29–40.
•This presentation of a nearly decade-long effort toward antibacterial discovery highlights the limitations of taking a purely target-based approach toward drug discovery
- Guiguemde WA, Shelat AA, Garcia-Bustos JF, et al. Global phenotypic screening for antimalarials. Chem Biol. 2012;19:116–129.
- Vincent F, Loria P, Pregel M, et al. Developing predictive assays: the phenotypic screening “rule of 3”. Sci Transl Med. 2015;7:293ps15.
•This work outlines the authors’ recommended criteria in developing an effective phenotypic assay, and emphasizes the importance of using physiologically relevant material and readouts
- Van Goor F, Hadida S, Grootenhuis PD, et al. Correction of the F508del-CFTR protein processing defect in vitro by the investigational drug VX-809. Proc Natl Acad Sci USA. 2011;108:18843–18848.
- Rottmann M, McNamara C, Yeung BK, et al. Spiroindolones, a potent compound class for the treatment of malaria. Science. 2010;329:1175–1180.
- Swinney DC. The contribution of mechanistic understanding to phenotypic screening for first-in-class medicines. J Biomol Screen. 2013;18:1186–1192.
- Schreiber SL, Kotz JD, Li M, et al. Advancing biological understanding and therapeutics discovery with small-molecule probes. Cell. 2015;161:1252–1265.
•This article summarizes the success stories that emerged from the NIH’s MLPCN screening program, and differentiates the characteristics of small-molecule probes and drugs
- Gregori-Puigjané E, Setola V, Hert J, et al. Identifying mechanism-of-action targets for drugs and probes. Proc Natl Acad Sci USA. 2012;109:11178–11183.
- Schirle M, Jenkins JL. Identifying compound efficacy targets in phenotypic drug discovery. Drug Discov Today. 2015. DOI:10.1016/j.drudis.2015.08.001.
•This review provides a good overview of the many techniques necessary to understand small-molecule MoA
- Schenone M, Dančík V, Wagner BK, et al. Target identification and mechanism of action in chemical biology and drug discovery. Nat Chem Biol. 2013;9:232–240.
- Kronkë J, Udeshi ND, Narla A, et al. Lenalidomide causes selective degradation of IKZF1 and IKZF3 in multiple myeloma cells. Science. 2014;343:301–305.
- Savitski MM, Reinhard FB, Franken H, et al. Tracking cancer drugs in living cells by thermal profiling of the proteome. Science. 2014;346:1255784.
- Hughes TR, Marton MJ, Jones AR, et al. Functional discovery via a compendium of expression profiles. Cell. 2000;102:109–126.
- Lamb J, Crawford ED, Peck D, et al. The Connectivity Map: using gene-expression signatures to connect small molecules, genes, and disease. Science. 2006;313:1929–1935.
- Gustafsdottir SM, Ljosa V, Sokolnicki KL, et al. Mutliplex cytological profiling assay to measure diverse cellular states. PLoS One. 2013;8:e80999.
- Petrone PM, Simms B, Nigsch F, et al. Rethinking molecular similarity: comparing compounds on the basis of biological activity. ACS Chem Biol. 2012;7:1399–1409.
- Dančík V, Carrel H, Bodycombe N, et al. Connecting small molecules with similar assay performance profiles leads to new biological hypotheses. J Biomol Screen. 2014;19:771–781.
- Lukens AK, Heidebrecht RW Jr, Mulrooney C, et al. Diversity-oriented synthesis probe targets plasmodium falciparum cytochrome b ubiquinone reduction site and synergizes with oxidation site inhibitors. J Infect Dis. 2015;211:1097–1103.
- Shalem O, Sanjana NE, Hartenian E, et al. Genome-scale CRISPR-Cas9 knockout screening in human cells. Science. 2014;343:84–87.
- Johannessen CM, Clemons PA, Wagner BK. Integrating phenotypic small-molecule profiling and human genetics: the next phase in drug discovery. Trends Genet. 2015;31:16–23.
- Plenge RM, Scolnick EM, Altshuler D. Validating therapeutic targets through human genetics. Nat Rev Drug Discov. 2013;12:581–594.
••This article discusses a genomics-based approach toward target validation and drug discovery, arguing that such human “experiments of nature” inspire more confidence in targets for therapeutics development
- Khurana V, Tardiff DF, Chung CY, et al. Toward stem cell-based phenotypic screens for neurodegenerative diseases. Nat Rev Neurol. 2015;11:339–350.
- Prior M, Chiruta C, Currais A, et al. Back to the future with phenotypic screening. ACS Chem Neurosci. 2014;5:503–513.
- Esch EW, Bahinski A, Huh D. Organs-on-chips at the frontiers of drug discovery. Nat Rev Drug Discov. 2015;14:248–260.
- Walpita D, Wagner BK. Evaluation of compounds in primary human islet cell culture. Curr Protoc Chem Biol. 2014;6:157–168.