ABSTRACT
Squamous cell carcinomas of the head and neck are the sixth most frequent tumors worldwide. Risk factors are carcinogenic exposure, infection with the human papillomavirus (HPV) and genetic predisposition. Lymph node metastasis in the neck and HPV status are major prognostic factors. There are several important clinical challenges that determine the research agenda in head and neck cancer. The first is more accurate staging, particularly of occult metastatic lymph nodes in the neck. A second challenge is the lack of biomarkers for personalized therapy. There are a number of treatment modalities that can be employed both single and in combination, but at present only site and stage of the tumor are used for treatment planning. Provided here is an overview of the successes and failures of molecular diagnostic approaches that have been and are being evaluated to address these clinical challenges.
Introduction
Head and neck squamous cell carcinoma (HNSCC) originates in the mucosal epithelial linings of the oral cavity, oropharynx, larynx or hypopharynx. In 2015, a predicted 650,000 patients worldwide will be diagnosed with the disease. In the same year, approximately 350,000 cancer deaths can be attributed to head and neck cancer.[Citation1]
Classic risk factors of HNSCC are excessive tobacco use and consumption of alcohol-containing beverages, particularly the combination of both.[Citation2] In the past decades, an important third risk factor was identified, which is most prominent in oropharyngeal squamous cell carcinoma (OPSCC): infection with an oncogenic human papillomavirus (HPV).[Citation3,Citation4] The significance of HPV as risk factor increased during the last decades, particularly affecting younger individuals.[Citation5] The prevalence of HPV-positive HNSCC depends on the geographical region. The highest prevalence and attributable fractions are reported in North America with approximately 70% of all OPSCC being caused by HPV.[Citation6] In Europe, prevalence rates vary but are generally between 30% and 60%.[Citation7] HPV-positive tumors are found most frequently in the oropharynx, but HPV is identified in tumors at other subsites as well.[Citation8] HPV-positive OPSCCs form a distinct subgroup, as they are clinically,[Citation9] histologically[Citation10] and molecularly[Citation11] different from the classical HPV-negative OPSCCs. Finally, a small group of HNSCCs is caused by inherited genetic predisposition disorders, such as Fanconi anemia and Familial Cutaneous Telangiectasia and Cancer Syndrome.[Citation12] Although these disorders are rare, the relative risk (RR) of developing head and neck cancer in Fanconi anemia patients is very high (RR 706, 95% CI 260–1540)[Citation13] and tumors may occur at very young age. We have decided to focus on squamous cell carcinoma only and therefore do not address nasopharyngeal or paranasal sinus cancer nor their specific risk factors in this review.
The prognosis of HNSCC depends primarily on the stage of disease, as defined by the TNM Classification of Malignant Tumors (TNM stage), and HPV status. TNM is defined by the local size and extension of the tumor (T-stage), the presence and extension of lymph node metastasis (N-stage) and the presence or absence of distant metastasis (M-stage) as defined by the Union for International Cancer Control. Other important prognostic features are histopathological variables (e.g. noncohesive growth, tumor-free margins and extranodal extension).[Citation14] Treatment planning is based on TNM stage and site. Early-stage local disease is generally treated by single-modality therapy (i.e. surgery or radiotherapy) and has a favorable prognosis. Advanced stage tumors are treated by multimodality therapy. Depending on the subsite, this can either be surgery with postoperative radiation or chemoradiation therapy or organ-preservation protocols using upfront concomitant chemoradiation with surgical salvage if needed.[Citation15,Citation16]
Unfortunately, the 5-year survival rates are still disappointing and improved only marginally in the past decades.[Citation17] This can be attributed to the frequent development of locoregional recurrences, distant metastasis and second primary tumors (SPTs). Local recurrences occur in 10–30% of the tumors[Citation18] and are defined as tumors that arise within 2 cm and within 3 years of the original primary tumor. Second primary tumors develop at a 3-year rate of 7.6%[Citation19] and are defined by any tumor that arises beyond 2 cm of the primary tumor or after 3 years at the same location.[Citation20] In the past, a lot of effort has been put in molecular detection of residual disease as a source of locoregional relapse. The research revealed a second type of local recurrences, besides those that originate from cancer cells that remain behind. Tumors develop within mucosal fields with preneoplastic changes, and these can be a source of local recurrences and SPTs as well.[Citation21,Citation22]
Clinical challenges
There are a number of clinical challenges in the management of HNSCC that dominate the scientific research agenda. Of note, these challenges may depend on the subsite of the tumors: oral cancer show other clinical characteristics than laryngeal tumors, which hampers generalizations. However, as most studies are not subsite-specific, we focus on HNSCC in general.
The first challenge is how to treat the clinically N0 neck in early-stage disease. Treatment of the neck depends in part on treatment of the primary tumor and is either by neck dissection or the lymph nodes at risk can be irradiated. The risk for occult metastases in the clinically N0 neck depends on subsite and stage. The best studied numbers derive from early-stage oral cavity tumors (cT1/cT2) and vary between 30% and 40%.[Citation23,Citation24] Hence, several strategies are defendable, which will either cause undertreatment or overtreatment of a substantial number of patients.
A second challenge is to personalize treatment. To allow this, biomarkers predicting therapy response or defining prognostic subgroups are required. Two major molecular subtypes of OPSCC are now distinguished based on HPV status. HPV-positive tumors have a favorable prognosis compared to the HPV-negative tumors[Citation25–Citation27] and this led to clinical treatment de-intensification trials (amongst others: NCT01874171, NCT01302834, NCT01084083).[Citation28] Given its clinical impact, it is important to detect these oncogenic HPV infections reliably. Next to the subdivision in HPV-positive and -negative HNSCC, large-scale molecular profiling studies have recently revealed more subgroups of tumors based on their molecular characteristics. Furthermore, molecular profiling studies have also been applied for the prediction of prognosis.
Molecular toolbox
The molecular toolbox to answer previously described clinical challenges has evolved dramatically in the past decades. Historically, to detect genetic alterations, specific genes or genomic regions of interest were examined individually, for example, by Sanger sequencing (point mutations) and microsatellite analysis (patterns of allelic loss). These techniques provided recurrent changes, but generally at a low resolution and with labor-intensive methods, which hampered a comprehensive identification of candidate cancer genes and associated genetic changes. Moreover, the cloning of these genes was cumbersome and the molecular tools to characterize these genes were limited to ectopic expression in cell lines.
After its discovery in 1992, comparative genomic hybridization (CGH)[Citation29] made the detection of copy number aberrations (CNAs) accessible on a genome-wide scale albeit with limited resolution. Additionally, after the implementation of array CGH, with DNA microarrays replacing the original metaphase chromosome spreads, it even became possible to analyze CNAs across the entire genome with initially moderate and later ultra-high resolution.[Citation30–Citation32] The same hybridization-based techniques were used to determine gene expression of many genes simultaneously in a tumor or other sample.[Citation33,Citation34] Later, platforms became available for genome-wide expression profiling, supporting integral molecular profiling of the transcriptome. During the last years, next-generation sequencing (NGS) has taken over the descriptive genomics field and is currently used to determine somatic mutations (whole-exome sequencing), gene expression profiles (RNA sequencing) and copy number alterations (low-coverage whole-genome sequencing).
Some limitations came along with this era of high-throughput descriptive genomics. First of all, the costs are still very high. To be able to clinically implement results of high-throughput studies, researchers have developed dedicated research platforms that are less expensive; examples are the MammaPrint dedicated microarray platform[Citation35] and Ampliseq Cancer Hotspot Panel for targeted sequencing.[Citation36] Another possibility has been explored by Nielsen et al.[Citation37] They developed an immunohistochemistry assay to distinguish the molecular classes of breast cancer that were previously described by Perou et al.[Citation38] on the basis of expression profiles.
A second limitation involves the interpretation of the data and the validation of results. New statistical techniques had to be developed to handle the statistical challenges of big data research. Interpretation became more difficult for biologists and clinicians, emphasizing the important role of biostatisticians and bioinformaticians. Initial studies were usually underpowered and suffered from a lack of thorough validation in independent series. Finally, the results of microarray analysis are affected by the use of different platforms, different experimental protocols and many other variables. Quite some effort has been made to improve the reproducibility, but it is far from optimal.[Citation39] NGS platforms are expectantly less sensitive to these difficulties, but the available gene expression data sets are still limited.
Besides the descriptive genomics toolbox, also the functional genomics toolbox increased tremendously with the introduction of retroviral and lentiviral vectors, germline modification of the mouse, small interference RNA gene expression knockdown and recently genome editing by, for example, CRISPR/Cas9 technology.
Carcinogenesis of HPV-negative HNSCC
Cancer, and also HNSCC, is caused by the accumulation of genetic and epigenetic alterations of genes in various signaling pathways. The most frequently affected pathways involve the cell cycle checkpoints (e.g. p53/pRb pathways), RTK/RAS/PI(3)K signaling (e.g. epidermal growth factor receptor (EGFR), PIK3CA, PTEN), differentiation (e.g. NOTCH1, FAT1, TP63) and cell death (e.g. FADD, CASP8). Most genes involved can be classified as tumor suppressor genes. An important exception is PIK3CA, which harbors activating mutations or copy number gains in one-tenth to one-third of the HPV-negative tumors.[Citation40–Citation42]
In 1996, Califano et al.[Citation43] presented the first stepwise genetic progression model of HNSCC, based on molecular analysis of histological premalignant mucosal changes and invasive cancers. They identified that the number of chromosomal aberrations (i.e. loss of heterozygosity (LOH) at specific loci) increases with histopathological progression. Furthermore, specific alterations were recurrently found in histological lesions that represent the early steps in the development of invasive cancer, that is, LOH at chromosomes 3p, 9p and 17p. These early genomic alterations were also the most frequent changes in the invasive tumors. Mutations in TP53 (17p) can be found in 60–84% of the HPV-negative tumors[Citation40,Citation42,Citation44] and mutations and/or focal deletions of CDKN2A (9p) in 57%.[Citation42] Deletions of chromosome 3p (often the entire arm) can be found in 80% of the tumors.[Citation42] Remarkably the critical cancer gene on 3p is still a mystery. Other genetic alterations were found in more advanced premalignant lesions and invasive cancer and are believed to occur later during carcinogenesis. This initial model was later revised by Braakhuis et al.[Citation45] that is described below (Section ‘Field cancerization’ and ).
Figure 1. Progression model of molecular carcinogenesis of oral squamous cell carcinoma.
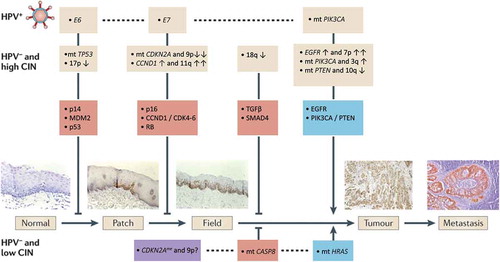
Recent NGS studies revealed many more candidate cancer genes. Alterations in these genes are commonly shared, but are generally less frequent and sometimes very infrequent. Examples are mutations in NOTCH1 (26%) and FAT1 (32%). An explanation for this finding might be that cell cycle regulation needs to be disturbed by few specific key players as an early step in the carcinogenesis process, whereas the other hallmarks of cancer are likely regulated by more divergent pathways and genes.
Carcinogenesis of HPV-positive HNSCC
The HPV genome consists of six early genes (E1–E7) and two late genes (L1 and L2). Two early genes (i.e. E6 and E7) have a role in oncogenesis,[Citation46] particularly when expressed in the basal epithelial layers. The E6 protein binds to tumor suppressor protein p53 causing its inactivation and degradation.[Citation47] The E7 protein binds to the RB pocket proteins also promoting their degradation.[Citation48] By E6 and E7 gene expression, the virus bypasses the G1/S block, permitting S-phase entry and viral replication.[Citation18] The induction of these processes by the viral oncogenes can be regarded as early changes in the carcinogenesis of HPV-positive tumors, comparable to mutations and/or deletions of TP53 and CDKN2A, the latter acting in the Rb pathway (see ). Evidence from studies of cervical cancer indicated that while E6 and E7 are necessary to initiate and maintain the transformation of normal epithelial cells to invasive cancer, expression of these viral oncogenes is not sufficient to develop a full-blown tumor. The alteration of additional oncogenic pathways is required for malignant progression.[Citation49] However, the modification of additional pathways is accelerated by the chromosomal instability that is caused by E6 and E7 expression.[Citation50] The deregulated cellular S-phase entry promotes DNA damage, and when p53-mediated DNA damage response is abrogated by E6, this may lead to DNA changes.[Citation51] Frequently affected pathways in HPV-positive HNSCC involve RTK/RAS/PI(3)K signaling (e.g. PIK3CA, FGFR3), differentiation (e.g. NOTCH1, TP63) and cell death (e.g. TRAF3).[Citation42]
Field cancerization
Besides describing the progression of oral mucosa to invasive cancer, Califano et al. noted that some genetic alterations were shared between the invasive tumors and the associated surrounding abnormal (noninvasive) mucosal cells, which suggests that these cells originated from a common progenitor.[Citation43] This finding supported the concept of ‘field cancerization’, hypothesized by Slaughter et al. in 1953 to explain the frequent development of local recurrences and SPTs in oral squamous cell carcinoma patients.[Citation52] They examined 783 resected oral tumors and concluded that these cancers were often surrounded by histologically abnormal epithelium possibly caused by long-term carcinogen exposure. Moreover, the finding of multiple independent invasive foci suggested a multiclonal origin of squamous cell cancer. The genetic explanation of the field cancerization concept was studied in depth and reported in 2001 by Tabor et al.[Citation53] These authors analyzed biopsies of tumors and macroscopically normal adjacent mucosa and found shared genetic alterations in 50% of the cases. These genetically abnormal cells were coined as fields. In 25% of the cases, these fields extended into the surgical margins and apparently remained behind, causing local relapses and SPTs.[Citation21,Citation22]
Subsequent research of immunohistochemical staining patterns of mutated p53 allowed microscopic visualization of the likely earliest changes in the mucosal epithelium.[Citation54] These alterations presented as small patches that preceded the fields. This observation refined the progression model for HNSCC,[Citation45] which is depicted schematically in . Of note, these models are mainly based on oral cancer and oropharyngeal cancer, but there are no reasons to assume that laryngeal or hypopharyngeal tumors follow other models. It was hypothesized that a mucosal stem cell becomes genetically altered and forms a ‘patch’ of clonally related daughter cells with the same genetic alteration (TP53 mutation). This clonal unit concept has recently been demonstrated by lineage tracing experiments in the skin epithelium in mice.[Citation55] Subsequent alterations would provide a survival benefit or enhanced proliferation to the genetically altered stem cell, thereby laterally replacing the normal epithelial stem cells. This results in the formation of a larger precancerous field. Some of these fields become visible and present as white (leukoplakia) or red (erythroplakia) lesions. As the field becomes larger, additional genetic hits give rise to various subclones within the field (clonal divergence). These subclones may take over the field and ultimately acquire sufficient genetic alterations to transform into an invasive tumor.
The concept of field cancerization seems at present to be specific for HPV-negative tumors. This concept has been studied under the assumption that HPV infection is indeed the first event in HPV-mediated carcinogenesis and that the presence of transcriptionally active HPV could serve as reliable biomarker of field cancerization. In this study, HPV16-E6-mRNA could not be identified in resection margins of 20 HPV-positive cancers,[Citation56] suggesting that HPV-positive tumors are not surrounded by these large fields. Although the apparent absence of fields surrounding HPV-positive tumors is in line with the favorable prognosis of HPV-positive disease, it strongly relies on the assumption that HPV infection indeed is the starting event. There are also some contrasting observations. There are reports of cases presenting with multiple synchronous HPV-positive HNSCCs (e.g. [Citation57]). This could be explained by the presence of preneoplastic fields, although multiple infections could explain this as well. Altogether, the concept of field cancerization in HPV-positive tumors remains an interesting topic for future studies.
Staging of the clinically N0 neck
Prominent lymph node metastases are discovered by palpation, but smaller metastases cannot be discriminated from negative nodes. It has been shown that approximately 30–40% of early-stage oral cancer patients have metastatic disease after pathological examination of the elective neck dissection specimen, despite being clinically staged as N0 (cN0) and apparently free of lymph node metastasis.[Citation23] Hence, if we rely on the clinical staging only and do not treat a cN0 neck in T1/T2 oral cancer patients, we will undertreat 30–40% of the patients. However, overtreatment will occur in 60–70% of the cN0 patients when the neck is treated electively, that is, treated when there is no clinical diagnosis of nodal disease. This dilemma fueled both imaging and molecular research and led to the development of several methods to select patients at high risk for occult metastasis while preventing overtreatment in the low-risk group.
To assist manual palpation, several radiological techniques (including ultrasound imaging, computed tomography, magnetic resonance imaging and positron emission tomography) have been tested, but for the detection of occult metastasis most lack the required accuracy.[Citation58,Citation59] However, a combination of ultrasound (US) combined with fine-needle aspiration cytology (FNAC) yields better performance. In this case, the nodal size on ultrasound is used to select lymph nodes at risk of nodal disease. Subsequently, these nodes are assessed by FNAC, the whole procedure abbreviated as USgFNAC. USgFNAC can achieve a sensitivity of 73% and specificity of 100%.[Citation60] However, the diagnostic performance depends on the criteria used to select the lymph nodes at risk, the experience of the radiologist and pathologist, as well as the subgroup of patients studied.[Citation60] In practice, the reported sensitivity in multicenter studies is generally lower.[Citation61,Citation62] The sensitivity is troubled by sampling error (in case of a micrometastasis) and aspiration of the wrong nodes. Furthermore, up to 20% of the aspirates are not evaluable due to insufficient quantity or quality of the material. To increase the sensitivity of USgFNAC and increase the number of evaluable aspirates, a real-time quantitative PCR assay was developed using squamous cell-specific marker hLy6D.[Citation63] This assay was quite successful, but unfortunately false positives were introduced, possibly due to the introduction of skin keratinocytes in the aspirates and the suboptimal specificity of the marker. Other studies demonstrated markers with higher specificity like pemphigus vulgaris antigen [Citation64] and squamous cell carcinoma antigen (SCCA).[Citation65] Use of these markers could reduce the number of false positives, but large studies are still awaited to prove that molecular analysis of the aspirates really improves USgFNAC.
Sampling error issues when analyzing aspirates can be circumvented when the first draining lymph nodes at highest risk, also indicated as the sentinel lymph nodes, could be sampled and analyzed by scrutinizing histology. In the last decades, the value of sentinel lymph node biopsy (SLNB) in staging of cN0 OSCC patients became increasingly evident. This technique attempts to identify the first draining lymph nodes by injecting radioactive colloid and/or a blue dye around the tumor. Subsequently, the radioactive and/or blue lymph nodes are removed by a small excision. It acts on the premise that cancer cells will metastasize to these sentinel lymph node(s) in advance of spreading more widely throughout the lymphatic system of the neck. Removing the first draining node followed by meticulous histopathological examination should discriminate between N0 and N+ cases. A neck dissection is performed in a second surgical procedure when the sentinel lymph node turns out tumor-positive. Reported sensitivities are between 80% and 91% and negative predictive values (NPVs) between 88% and 94%.[Citation66–Citation68] A caveat is the reduced performance in tumors located at the floor of mouth due to difficult detection of the sentinel lymph node.[Citation67] In addition, the need of two surgical procedures when the sentinel node is tumor-positive hampers introduction of SNLB in common clinical practice. Also, fibrosis of the neck due to SLNB may complicate future surgical procedures that are necessary if a delayed lymph node metastasis develops in the operated region of the neck.
Reverse transcriptase PCR (RT-qPCR) assays have been developed to assess the presence of cancer cells in the removed lymph node during surgery next to scrutinizing histopathology. The accuracies of these assays are quite acceptable, ranging from 86% to 100% using conventional pathological examination as reference.[Citation65,Citation69] Unfortunately, the actual value of these assays has not been assessed in a prospective clinical setting. An intrinsic problem is that it is almost impossible to prove additional value over scrutinizing histopathology. In the studies, usually the lymph nodes are halved. Next, one half is analyzed by scrutinizing histopathology and the other half by RT-qPCR. Differences between histology and molecular assays may, therefore, be explained by sampling error instead of different test performance.
Alternative approaches have been evaluated. In studies in breast cancer patients, it was observed that metastasizing and nonmetastasizing breast tumors could be distinguished at baseline on basis of their expression profiles.[Citation70] In a number of subsequent papers, the value of gene expression profiling has been determined in HNSCC to predict whether a tumor metastasized to the neck or not.[Citation71–Citation75] Gene expression signatures showed surprisingly little overlap and reported accuracies vary significantly. Many studies have not been reproduced properly, limiting possible clinical implementation. Most promising in this respect was the initial study published by Roepman et al. The authors identified a set of 852 genes that predicted N-stage,[Citation75,Citation76] and this profile was validated using a dedicated diagnostic microarray platform in a multicenter setting.[Citation77] Furthermore, the authors specifically explored the performance of their signature in the clinically relevant cT1-2N0 HPV-negative oral cancer subgroup. They found a higher NPV (89%) and sensitivity (86%) in these early-stage patients compared to all patients. Nonetheless, despite the validation in multicenter setting, this profile has also not been implemented in clinical practice due to high costs and absence of an application to formalin-fixed paraffin-embedded (FFPE) tissue. In particular for the clinically relevant cT1-T2 oral cancer group, frozen samples are difficult to obtain. Furthermore, to be competitive with other techniques such as SLNB, the NPV should be preferably 95% or higher to make it the method of choice. Ultimately, a combination of both methods could be an interesting approach.[Citation78]
Early detection of recurrent disease by molecular diagnosis
As stated above, the frequent development of local and regional recurrences is a major problem in the treatment of HNSCC patients. Depending on stage and site, local and/or regional recurrence rates have been reported of 20–50%.[Citation79,Citation80] Moreover, distant metastasis occur in 10–40%[Citation81] during follow-up. Two explanations of this relatively high incidence of locoregional recurrence have been suggested.[Citation18] First, residual tumor cells that are undetectable by standard histological and radiological examination can remain in situ after surgery. These residual cells have been defined minimal residual cancer (MRC). Furthermore, it has been shown and described above that tumors develop in precancerous fields that can be 5–10 cm in diameter. Part of this precancerous field can extend into the surgical margins and remain behind unnoticed. Additional genetic alterations can cause outgrowth of a second tumor from the residual premalignant field (‘second field tumor’). Second field tumors can occur in close proximity of the primary resected tumor and within 3 years. These tumors are clinically diagnosed as local recurrences. Second field tumors can also develop at more distant sites or after 3 years and are then diagnosed as SPTs. Hence, local recurrences can be subdivided in two categories, those resulting from MRC and those resulting from fields that remained behind. The different types of recurrent disease are illustrated in .
Figure 2. Field cancerization and local relapse.
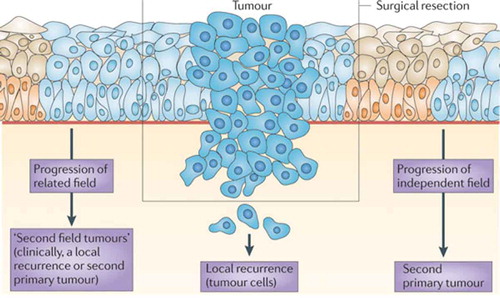
Detection of minimal residual head and neck cancer
First attempts to detect residual cancer cells in margins that were histologically free of tumor were published 20 years ago by Brennan et al. These authors used TP53 mutations as molecular marker, which was elegantly detected by ‘plaque assays’.[Citation82]The authors showed that mutated TP53 in the surgical margins is associated with the risk for locoregional recurrence. In many subsequent studies, the paradigm remained unchanged: detection of TP53 mutations (if present in the primary tumor) in the corresponding margins is a risk factor for locoregional recurrence.[Citation83,Citation84] However, techniques based on detection of mutated DNA lack specificity. Partly, this can be explained by the use of mutated TP53. This is an early change in carcinogenesis and will not detect only residual tumor cells but also residual precancerous fields that do not always progress. Residual tumor cells should be treated by postoperative radiotherapy, but this is not indicated for residual fields. Furthermore, mutated DNA leaks from necrotic tumors. In particular, the latter combined with the high sensitivity of the methods causes specificity problems. Other research groups have suggested to exploit other DNA markers such as methylation markers[Citation85] or microsatellite markers[Citation86] to determine the presence of minimal residual disease, but the performance was similar to mutated TP53 analysis and most studies were small.
DNA is a very stable molecule that easily causes contamination problems. Therefore, RNA-based assays to detect MRC have been evaluated as well. These tests were expected to be more specific than DNA-based assays.[Citation83] Several genes were considered promising markers since they were highly expressed in HNSCC tumor cells, but not in stromal cells. Examples are hLy-6D, SCCA and EGFR. These RT-PCR-based assays usually have a very high sensitivity and are, for example, able to detect a single tumor cell in a background of 107 nucleated blood cells.[Citation87] However, a prospective study including 105 cases failed to show prognostic relevance of hLy6D expression in deep surgical margins.[Citation88] Even when the tumors with high hLy6D expression were analyzed as subgroup and between 5 and 15 biopsies per patient were analyzed, there was no association with clinical outcome.
One of the major issues that was indicated by the authors and has to be addressed in future research is the sampling of these margins. Even 15 biopsies are subject to sampling-error given the large resections. A more comprehensive way of sampling the margins could overcome the false-negative results. Wrapping the specimen in nitrocellulose and analysis of the DNA by methylation markers has been tried, but thus only far in very small series.[Citation89]
Despite that the concept of detecting residual tumor cells in tissue samples by molecular methods is very attractive, the sampling error problem, that also hampers histological examination, is an issue that is not easily solved. RT-qPCR methods using histogenic markers have the additional disadvantage that false-positive results are easily found due to the presence of normal epithelial cells in the superficial margins. Tumor-specific DNA markers (e.g. TP53 mutations) have the limitation that DNA from necrotic tumor cells contaminate the samples, causing false-positive results, and might detect precancerous changes in superficial margins. Hence, the results of all these studies still leave much to be desired. In later studies, expression profiling studies to predict local recurrences have been tried as well, but the associations were generally not strong and profiles have not yet been adequately validated.[Citation90,Citation91]
Nonetheless, the gains are high: postoperative radiotherapy or nowadays postoperative chemoradiation could be tailored on the basis of the presence of residual disease. An intrinsic problem remains that local recurrences may develop from two different sources: tumor cells and precancerous fields that stayed behind. This has major biological but also clinical consequences. Hence, it will be required to make a separation between residual tumor cells and residual fields, particularly when superficial margins containing mucosal epithelium are analyzed.
The fact that DNA is stable and easily contaminates margin samples has an intriguing other application. The shedding of DNA might well result in tumor-specific alterations in the blood. Already in the 1990s microsatellite alterations in the serum of HNSCC patients were discovered.[Citation92] Since then, new techniques have been developed to detect what is now called circulating tumor DNA (ctDNA). At present, two frequently applied techniques are NGS and digital PCR. Relatively few studies focused on the detection of ctDNA in HNSCC. In 2014, Bettegowda et al.[Citation93] explored the detectability of ctDNA in 15 types of cancer including 10 advanced stage HNSCC patients. CtDNA was detectable in the majority, but results are difficult to interpret given the small number of cases. More recently, Wang et al.[Citation94] published a study that focused specifically on the detection of ctDNA in HNSCC. They performed targeted sequencing of recurrently mutated genes and the HPV16 E6 gene in DNA extracted from plasma (47 patients) and saliva samples (93 samples). Depending on the subsite, ctDNA in plasma was detectable in 80–100% of the cases, whereas tumor DNA was detected in 47–100% in saliva. Moreover, post-treatment saliva samples in a subset of cases (n = 9) showed positive tumor DNA in advance of clinical relapse in 4/9 cases. The remaining five cases had no detectable tumor DNA in their saliva during follow-up and remained clinically disease free. These results suggest new approaches for early detection of residual/recurrent disease. However, numbers are small and postoperative saliva sampling was performed at random time points. Longitudinal sampling combined with optimized detection methods will be required to assess the clinical implications.
Detection of residual preneoplastic fields
Residual preneoplastic fields are responsible for approximately 1/3 of local relapses. Moreover, SPTs in the upper aerodigestive tract occur in an annual rate of approximately 2–3%. SPTs, too, can derive from residual precancerous fields. Second primary tumors can either be clonally related to the first tumor or derive from an independent field. Early detection of these (residual) preneoplastic fields could provide opportunities to prevent its progression to invasive carcinoma, for example, by chemoprevention. Several methods have been published to identify fields and assess the risk for malignant progression. Importantly, histological changes (e.g. dysplasia) have been shown to be suboptimal to detect premalignant changes or predict malignant potential of the fields. Other methods have been evaluated, which mostly focus on the detection of genomic alterations in tissue biopsies. This can be tested in biopsies from both visible lesions (e.g. leukoplakia) and the mucosal margins of surgical specimens. Retrospective studies as early as 1996 have convincingly shown that detection of specific genetic alterations (e.g. LOH at chromosome 9p and 3p) are important for risk assessment of oral precancer.[Citation95–Citation97] These findings were also found to be applicable for analyses of surgical margins.[Citation98] Moreover, prospective evaluation of these markers was successful,[Citation99,Citation100] ultimately demonstrating the added value of detection of these genomic alterations in premalignant fields. Remarkably, this has not become the new clinical standard. Clinical implementation is hampered mainly by the fact that, despite excellent prognostic stratification of patients, for the individual patient it does not have clinical consequences. Although not very effective, it is generally accepted to remove visible preneoplastic lesions (leukoplakia) surgically, followed by active surveillance.[Citation101] Given the limited toxicity of this protocol, this policy is not affected by molecular stratification of patients. This may change when more effective, but also more toxic, treatments become available. When effective therapeutic interventions have been developed and tested to reduce malignant transformation and improve overall survival, a simple LOH-based or NGS-based test might become an important companion diagnostic assay.
Also, screening for and monitoring of fields might become an option by noninvasive diagnostic approaches using brushed cells or saliva. Promising results were shown in a study on the detection of specific genomic alterations in exfoliated cells sampled by brush cytology. In a pilot study using allelic imbalance of cancer-associated chromosome regions assessed by 12 microsatellite markers, an estimated sensitivity of 78% and a specificity of 100% was determined.[Citation102] However, in a larger follow-up study the analytical sensitivity reduced to 45%.[Citation100] Future studies with more sensitive NGS techniques may improve these test characteristics.
Besides noninvasive molecular diagnosis, also visualization of premalignant fields may be very helpful. Poh et al. showed that loss of autofluorescence in tumor adjacent mucosa is associated with histological and molecular premalignant changes.[Citation103,Citation104] Furthermore, these authors developed a handheld device to determine loss of fluorescence visualization in the operating theater and are currently assessing its value in a randomized controlled trial (COOLS trial).[Citation105] Notwithstanding, detecting and risk assessment of fields is one step, but new and perhaps targeted chemopreventive therapies need to be developed and translated from early experimental phases to clinical trials.
In conclusion, the detection of residual precancerous fields is possible and clinically relevant when using tissue biopsies. The applied methods have been validated thoroughly. Future research should focus on noninvasive detection of these fields. A noninvasive test could also be applied to invisible premalignant fields, which could be specifically interesting for screening of high-risk subjects. However, in parallel to diagnostic approaches, chemopreventive therapies have to be developed, allowing detected precancerous changes to be treated.
Molecular classification of HNSCC: HPV
As indicated above, molecular detection and risk assessment of preneoplastic changes is quite effective. In contrast, detection of residual disease has not been very successful to identify patients at high risk for locoregional recurrence or distant metastases. The intrinsic issue of sampling error hampers applications and other approaches are required. With the upcoming of reliable, high-throughput profiling methods, molecular stratification of HNSCC seems to be the most interesting alternative approach. The most pronounced molecular subgroup in HNSCC is defined by HPV status. Intriguingly, these tumors show very different molecular profiles including a lack of TP53 mutations and fewer chromosomal aberrations that are typically present in HPV-negative HNSCC.[Citation11,Citation106–Citation108] Moreover, HPV-positive and -negative tumors can be distinguished by gene expression profiles[Citation109] and epigenetic signatures.[Citation110,Citation111] Updates of these molecular differences using NGS have recently been published by the Cancer Genome Atlas[Citation42] and Seiwert et al.[Citation41] The majority of previous findings were confirmed. Additionally, new findings provided a more comprehensive overview of the molecular landscape of HPV-positive HNSCC. In contrast to results of previous studies,[Citation40,Citation44] the absolute number of somatic mutations was found to be comparable between HPV-positive and HPV-negative tumors.[Citation42] However, as expected, the landscape of somatic mutations and copy number alterations differed importantly. Altogether, HPV-positive tumors can be considered a very well-validated molecular subgroup of HNSCC with major impact on prognosis. In fact, HPV-positive OPSCC patients have such a favorable prognosis that treatment de-intensification trials have been initiated.
The increasing importance of HPV-positive tumors as a separate molecular and clinical subgroup of HNSCC also fueled research for reliable HPV detection methods, particularly of archival FFPE tumor specimen. In theory, this is easily determined by HPV DNA detection, but in practice this turned out to be quite a challenge. Most assays have been borrowed from the cervical cancer screening research field and these PCR-based assays are generally very sensitive, causing false-positive results. The generally accepted ‘gold standard’ test for tumor tissue is the detection of E6 and E7 mRNA expression detected by RT-PCR. The RT-PCR assay can be reliably performed on mRNA from fresh-frozen tumor tissue. However, these standard assays are less reliable on mRNA that is isolated from FFPE tissue since the RNA is degraded and of poor quality. This is a major disadvantage because often only FFPE material of tumors is available. Hence, other HPV detection methods have been developed with variable performances.
The most frequently applied methods are PCR-based approaches to detect HPV DNA. However, due to the high sensitivity, these methods result in false-positive results.[Citation112] A second, commonly utilized method is fluorescence in situ hybridization. This test is highly specific, but relatively difficult to implement and the sensitivity is suboptimal (83–88%).[Citation113,Citation114] The third procedure relies on the detection of surrogate markers in combination with HPV detection assays. The most widely applied surrogate marker is the expression of p16 (encoded by CDKN2A). In OPSCC, there is a high correlation between p16 expression and the presence of a transcriptionally active HPV infection. However, this test lacks specificity when used as individual test (false-positive rates between 15% and 20%).[Citation113–Citation115] Algorithms have been developed to overcome the weaknesses of using individual tests. Smeets et al.[Citation114] assessed the performance of several combinations of individual HPV tests in OPSCC. They found that an algorithm of p16 expression followed by detection of high-risk HPV DNA by GP5+/6+ PCR on the p16-positive samples showed the highest correlation to E6/E7 mRNA expression in the corresponding frozen specimen. This was later confirmed in a follow-up study of independent cases.[Citation7] Thus far, no algorithm has been developed for other subsites besides the oropharynx. The sensitivity of immunostaining for p16 in subsites other than the oropharynx seems to be much lower.[Citation8,Citation116] Hence, a reliable and simple test for HPV applicable to all tumor sites still needs to be developed and, more importantly, thoroughly validated. This would enable us to obtain reliable figures on incidence and prevalence of HPV-positive tumors outside the oropharynx and, more importantly, determine whether HPV presence is also a major prognostic marker for non-OPSCC. For OPSCC, test algorithms that include p16-immunostaining and some kind of HPV detection can be considered as well validated and reliable.
With the start of treatment de-intensification trials in OPSCC and personalized treatment based on HPV status at the horizon, the importance of accurate HPV testing has again come to attention, but thus far, no consensus in the community has been reached.
Molecular classification of HNSCC: other subclasses
Besides the subclass of tumors defined by HPV-status, a third molecular subgroup is emerging. This subgroup was first discovered by Smeets et al.[Citation117] and is characterized by a very limited number of genetic changes and wild-type TP53 status. Tumors are HPV-negative and arise primarily in the oral cavity. A recent report of the TCGA[Citation42] confirmed that approximately 15% of the cases showed very few chromosomal aberrations (somatic copy number alteration (SCNA) quiet). Moreover, this subset was enriched with activating mutations in HRAS, inactivating mutations in CASP8 and wild-type TP53. Molecular findings are supported by clinical behavior as this SCNA quiet subtype has a markedly favorable prognosis compared to other HPV-negative tumors (supplementary figure S1.5 of TCGA paper).[Citation42]
However, these stratifications rely on HPV presence, genetic changes and mutational profiles. In the landmark study by Perou et al.,[Citation38] subclasses of breast cancer were identified using expression profiling, an observation that changed the breast cancer research field. Along these lines, several groups have studied the molecular classification of HNSCC by gene expression signatures. The first study was performed by Chung et al. using microarray data of 60 tumors.[Citation71] They identified four subtypes that were later referred to as basal (BA), mesenchymal (MS), atypical (AT) and classical (CL).[Citation118] In retrospect, a limitation of this study was that assessment of HPV status was not standard at that period. More recently, meta-analyses have been performed to detect and validate molecular subtypes in larger cohorts,[Citation119,Citation120] which support the existence of these subclasses. The referred studies show a strong correlation to the original subtypes defined by Chung et al.[Citation71] The breast cancer classification shows a clear difference in clinical response between the different subgroups.[Citation121] These findings have been carefully validated.[Citation122–Citation124] Similar clinical relevance of discovered molecular subtypes was demonstrated in HNSCC. However, despite the strong correlation of the different signatures, these studies showed conflicting clinical associations. For example, while ‘basal-like’ tumors showed the worst prognosis in the paper of Chung et al.,[Citation71] Walter et al.[Citation118] showed that ‘atypical’ tumors had the poorest outcome. Another difficulty is caused by the inclusion of HPV-positive tumors. Cluster analyses often show enrichment of HPV-positive cases in certain clusters, which is expected based on their different biological background. However, HPV-positive tumors also cluster together with HPV-negative tumors frequently.[Citation42] Unfortunately, this separation of HPV-positive tumors in different clusters is not explained by a separate clinical behavior.[Citation120] Hence, the subclasses identified by unsupervised cluster analyses of expression profiles do not match with the relevant genetic subtypes, that is, HPV-positive and HPV-negative tumors and within the HPV-negative tumors the SCNA-quiet group.
In summary, molecular subtypes of HNSCC characterized by either HPV status or few genomic alterations are emerging; independent final validation in large cohorts of various populations is still necessary. Moreover, additional molecular subtypes of HNSCC identified by gene expression profiling seem to be present, but clinical applications need further investigation and validation.
Prognostic modeling of HNSCCs
Besides TNM and some histological features, the HPV status is the most important prognostic marker in HNSCC. Several studies from a variety of countries demonstrated that patients with HPV-positive OPSCC have a strikingly prognostic advantage compared to HPV-negative OPSCC patients.[Citation25,Citation26] A number of multivariate prognostic risk models that include HPV status and other risk factors have been developed for OPSCC. [Citation25,Citation26,Citation125,Citation126]
Interestingly, patients with HPV-positive OPSCC show a favorable prognosis, but a subgroup of these patients does not. To some extent, the above-mentioned risk models enable stratification of HPV-positive patients in high and low risk by the use of clinical variables (e.g. smoking, comorbidity, stage of disease). However, the identification at baseline of HPV-positive tumors with poor prognosis is still suboptimal. Biomarkers are under investigation and promising results include the quantification of tumor-infiltrating lymphocytes,[Citation127,Citation128] expression of human leukocyte antigen class I[Citation129] and expression of cancer stem cell enrichment markers (e.g. CD98).[Citation130] However, results should be considered experimental and validation in larger cohorts is required.
Other biomarkers for prognostic modeling are still awaited, especially to stratify HPV-negative HNSCC patients in high- and low-risk groups. One approach is through gene expression profiling. Several groups have used microarray data to predict metastatic behavior, locoregional recurrence and/or overall survival.[Citation76,Citation91,Citation131–Citation133] However, clinical implementation lags behind. Thinkable reasons are the inadequate reproducibility of the classification algorithms, the high costs and the lack of applications for FFPE specimens. Combining multiple data sets is a possible solution to improve the reproducibility,[Citation131] but very well-annotated clinical data (including HPV-status, pN-stage, follow-up data, etc.) are required to make maximum use of the data sets. Independent validation by other techniques would probably substantiate the reproducibility, but is frequently missing. Moreover, in most studies heterogeneous patient populations are studied (e.g. regarding HPV status, treatment and tumor subsite) and often studies are underpowered. This does not affect generation of hypotheses, but it hampers clinical implementation in the end.
Expert commentary
For some important clinical challenges in the management of head and neck cancer, solutions seem at the horizon. Staging of the clinically N0 neck has improved tremendously with the introduction of expression profiling and particularly SLNB. Some challenges have remained. SNLB still requires two surgical procedures when the sentinel node appears positive, the performance is less accurate in tumors located at the floor of the mouth and the evidence of this technique is primarily based on tumors located in the oral cavity.[Citation134] An attractive alternative is stratification of patients by gene expression profiling of the primary tumor. Results look promising, but higher accuracy is necessary, preferably combined with a cheaper, more user-friendly test that is applicable to FFPE material.
A second clinical problem is the standstill of prognosis during the last decades, despite the application of continuously more invasive treatment protocols. The incidence of locoregional recurrences, SPTs and distant metastasis remains high and forms an important challenge to address, possibly by personalization of treatment and novel therapies. Local relapses can develop by two mechanisms: tumor cells can remain in situ after treatment (MRC) and precancerous fields that remain behind can develop into new tumors (second field tumors). Molecular diagnostics of MRC has been studied extensively but faces technical challenges. Sampling errors lead to false-negative results, and contamination of margins by DNA from necrotic tumor cells causes false-positive results. These central problems seem difficult to solve. The importance of residual preneoplastic fields has been convincingly demonstrated and well-validated simple assays are at hand. The challenges that remain are visualization of fields and, most importantly, chemopreventive treatments to prevent malignant progression into cancer. Only the combination of accurate diagnosis and treatment might decrease the number of second field tumors and improve prognosis of HNSCC patients.
Finally, personalized therapy can optimize clinical responses and prevent unwanted effects as much as possible. Molecular classification of HNSCC patients may help to select patients for the most effective therapies. Thus far, HPV-positive OPSCC is the best validated clinically relevant subtype of HNSCC and personalized treatment strategies (de-intensified regimens) are currently tested in clinical trials. A second molecular subtype is emerging that is characterized by few copy number alterations, frequent mutations in HRAS, as well as CASP8 and TP53 wild type. Other molecular classifications have been proposed, but these have not reached the stage of maturation to make it to the clinic.
Five-year view
At present, two molecular subgroups of HNSCC have been established, that is, HPV-positive HNSCC and HPV-negative HNSCC. In oropharyngeal cancers, this has led to treatment de-intensification trials. Future research will determine the importance of HPV in nonoropharyngeal sites. Optimal and well-validated detection methods to detect HPV in these subsites will be developed.
The existence of a CNA-silent group has been demonstrated in at least two studies. In the next years, simple methods to distinguish this group using FFPE specimen will facilitate large studies to analyze its existence and clinical relevance. We expect that other molecular subgroups will emerge that relate to prognosis. Whether prognostic associations will be strong enough to personalize therapy remains to be determined.
Given the excellent performances, we foresee that the introduction of molecular profiling and particularly SLNB will change the clinical management of the N0 neck. There is room for both, particularly when profiling can become applicable to FFPE specimens and reaches a higher sensitivity.
Technical developments and future research will hopefully resolve the most prominent question in the detection of minimal residual disease in the surgical margins: will this become sensitive enough to overcome the intrinsic issue of sampling error?
Detection and risk assessment of fields is well accepted, although new NGS-based assays will emerge. These will also fuel noninvasive applications, both local and systemic. The challenge here is to find a preventive treatment to eradicate these fields when diagnosed.
At present, only site and stage of tumor are used for treatment planning of head and neck squamous cell carcinoma (HNSCC).
Both molecular profiling and SLNB are promising tools to optimize lymph node staging and adequate management of the clinically N0 neck.
Prognosis of HNSCC improved only moderately during the last decades. This improvement may relate to a steadily increase in oropharyngeal cancers caused by human papillomavirus (HPV) that have a very favorable prognosis.
Detection of residual cancer cells may provide early discovery of recurrent disease and tailoring of postoperative radiation or chemoradiation therapy, but is hampered by sampling error.
Detection of premalignant fields by molecular markers reliably predicts malignant transformation. Noninvasive diagnostics may further enhance clinical implementation.
At present, two clinically relevant molecular subgroups are recognized: HPV-positive tumors and HPV-negative tumors.
Personalized treatment of HPV-positive tumors is within reach, but accurate detection of HPV in formalin-fixed paraffin-embedded specimen is still challenging.
A third molecular subgroup is emerging, which is characterized by few chromosomal aberrations, wild-type TP53 and a favorable prognosis.
Other molecular subgroups of HNSCC have been established with gene expression profiling, but the clinical relevance has to be established in the future.
Financial & competing interests disclosure
This project has received funding from the European Union’s Seventh Framework Programme (FP7/2007–2013) under grant agreement 611425. The molecular cloning and applications in diagnostics of the hLy-6D antigen was co-sponsored in the past by Centocor BV, Leiden, The Netherlands. The authors have no other relevant affiliations or financial involvement with any organization or entity with a financial interest in or financial conflict with the subject matter or materials discussed in the manuscript apart from those disclosed.
References
- Papers of special note have been highlighted as:
- • of interest
- •• of considerable interest
- Ferlay J, Soerjomataram I, Dikshit R, et al. Cancer incidence and mortality worldwide: sources, methods and major patterns in GLOBOCAN 2012. Int J Cancer. 2015;136(5):E359–386.
- Forastiere A, Koch W, Trotti A, et al. Head and neck cancer. N Engl J Med. 2001;345(26):1890–1900.
- Syrjänen K, Syrjänen S, Lamberg M, et al. Morphological and immunohistochemical evidence suggesting human papillomavirus (HPV) involvement in oral squamous cell carcinogenesis. Int J Oral Surg. 1983;12(6):418–424.
- Gillison ML, Koch WM, Capone RB, et al. Evidence for a causal association between human papillomavirus and a subset of head and neck cancers. J Natl Cancer Inst. 2000;92(9):709–720.
- Chaturvedi AK, Engels EA, Anderson WF, et al. Incidence trends for human papillomavirus-related and -unrelated oral squamous cell carcinomas in the United States. J Clin Oncol. 2008;26(4):612–619.
- Mehanna H, Beech T, Nicholson T, et al. Prevalence of human papillomavirus in oropharyngeal and nonoropharyngeal head and neck cancer–systematic review and meta-analysis of trends by time and region. Head Neck. 2013;35(5):747–755.
- Rietbergen MM, Leemans CR, Bloemena E, et al. Increasing prevalence rates of HPV attributable oropharyngeal squamous cell carcinomas in the Netherlands as assessed by a validated test algorithm. Int J Cancer. 2013;132(7):1565–1571.
- Chung CH, Zhang Q, Kong CS, et al. p16 protein expression and human papillomavirus status as prognostic biomarkers of nonoropharyngeal head and neck squamous cell carcinoma. J Clin Oncol. 2014;32(35):3930–3938.
- Gillison ML, D’Souza G, Westra W, et al. Distinct risk factor profiles for human papillomavirus type 16-positive and human papillomavirus type 16-negative head and neck cancers. J Natl Cancer Inst. 2008;100(6):407–420.
- Westra WH. The morphologic profile of HPV-related head and neck squamous carcinoma: implications for diagnosis, prognosis, and clinical management. Head Neck Pathol. 2012;6(Suppl 1):S48–54.
- Braakhuis BJ, Snijders PJ, Keune WJ, et al. Genetic patterns in head and neck cancers that contain or lack transcriptionally active human papillomavirus. J Natl Cancer Inst. 2004;96(13):998–1006.
- Tanaka A, Weinel S, Nagy N, et al. Germline mutation in ATR in autosomal- dominant oropharyngeal cancer syndrome. Am J Hum Genet. 2012;90(3):511–517.
- Rosenberg PS, Greene MH, Alter BP. Cancer incidence in persons with Fanconi anemia. Blood. 2003;101(3):822–826.
- Woolgar JA, Triantafyllou A. A histopathological appraisal of surgical margins in oral and oropharyngeal cancer resection specimens. Oral Oncol. 2005;41(10):1034–1043.
- Grégoire V, Lefebvre JL, Licitra L, et al. Group E-E-EGW. Squamous cell carcinoma of the head and neck: EHNS-ESMO-ESTRO Clinical Practice Guidelines for diagnosis, treatment and follow-up. Ann Oncol. 2010;21:Suppl 5v184–186.
- Pfister DG, Ang KK, Brizel DM, et al. Head and neck cancers. J Natl Compr Canc Netw. 2011;9(6):596–650.
- Braakhuis BJ, Leemans CR, Visser O. Incidence and survival trends of head and neck squamous cell carcinoma in the Netherlands between 1989 and 2011. Oral Oncol. 2014;50(7):670–675.
- Leemans CR, Braakhuis BJ, Brakenhoff RH. The molecular biology of head and neck cancer. Nat Rev Cancer. 2011;11(1):9–22.
- Gan SJ, Dahlstrom KR, Peck BW, et al. Incidence and pattern of second primary malignancies in patients with index oropharyngeal cancers versus index nonoropharyngeal head and neck cancers. Cancer. 2013;119(14):2593–2601.
- Braakhuis BJ, Tabor MP, Leemans CR, et al. Second primary tumors and field cancerization in oral and oropharyngeal cancer: molecular techniques provide new insights and definitions. Head Neck. 2002;24(2):198–206.
- Tabor MP, Brakenhoff RH, Ruijter-Schippers HJ, et al. Multiple head and neck tumors frequently originate from a single preneoplastic lesion. Am J Pathol. 2002;161(3):1051–1060.
- Tabor MP, Brakenhoff RH, Ruijter-Schippers HJ, et al. Genetically altered fields as origin of locally recurrent head and neck cancer: a retrospective study. Clin Cancer Res. 2004;10(11):3607–3613.
- van den Brekel MW, van der Waal I, Meijer CJ, et al. The incidence of micrometastases in neck dissection specimens obtained from elective neck dissections. Laryngoscope. 1996;106(8):987–991.
- D’Cruz AK, Vaish R, Kapre N, et al. Elective versus Therapeutic Neck Dissection in Node-Negative Oral Cancer. N Engl J Med. 2015;373(6):521–529.
- Ang KK, Harris J, Wheeler R, et al. Human papillomavirus and survival of patients with oropharyngeal cancer. N Engl J Med. 2010;363(1):24–35.
•• A multiparameter prognostic model of advanced stage oropharyngeal cancer is presented that provides insights into the major prognostic advantage of human papillomavirus (HPV)-related oropharyngeal squamous cell carcinoma (OPSCCs) compared to HPV-negative tumors.
- Rietbergen MM, Brakenhoff RH, Bloemena E, et al. Human papillomavirus detection and comorbidity: critical issues in selection of patients with oropharyngeal cancer for treatment De-escalation trials. Ann Oncol. 2013;24(11):2740–2745.
- Rietbergen MM, Witte BI, Velazquez ER, et al. Different prognostic models for different patient populations: validation of a new prognostic model for patients with oropharyngeal cancer in Western Europe. Br J Cancer. 2015;112(11):1733–1736.
- Mirghani H, Amen F, Blanchard P, et al. Treatment de-escalation in HPV-positive oropharyngeal carcinoma: ongoing trials, critical issues and perspectives. Int J Cancer. 2015;136(7):1494–1503.
- Kallioniemi A, Kallioniemi OP, Sudar D, et al. Comparative genomic hybridization for molecular cytogenetic analysis of solid tumors. Science. 1992;258(5083):818–821.
- Solinas-Toldo S, Lampel S, Stilgenbauer S, et al. Matrix-based comparative genomic hybridization: biochips to screen for genomic imbalances. Genes Chromosomes Cancer. 1997;20(4):399–407.
- Pinkel D, Segraves R, Sudar D, et al. High resolution analysis of DNA copy number variation using comparative genomic hybridization to microarrays. Nat Genet. 1998;20(2):207–211.
- Pollack JR, Perou CM, Alizadeh AA, et al. Genome-wide analysis of DNA copy-number changes using cDNA microarrays. Nat Genet. 1999;23(1):41–46.
- Schena M, Shalon D, Davis RW, et al. Quantitative monitoring of gene expression patterns with a complementary DNA microarray. Science. 1995;270(5235):467–470.
- Lashkari DA, DeRisi JL, McCusker JH, et al. Yeast microarrays for genome wide parallel genetic and gene expression analysis. Proc Natl Acad Sci U S A. 1997;94(24):13057–13062.
- Cardoso F, van’t Veer L, Rutgers E, et al. Clinical application of the 70-gene profile: the MINDACT trial. J Clin Oncol. 2008;26(5):729–735.
- Singh RR, Patel KP, Routbort MJ, et al. Clinical validation of a next-generation sequencing screen for mutational hotspots in 46 cancer-related genes. J Mol Diagn. 2013;15(5):607–622.
- Nielsen TO, Hsu FD, Jensen K, et al. Immunohistochemical and clinical characterization of the basal-like subtype of invasive breast carcinoma. Clin Cancer Res. 2004;10(16):5367–5374.
- Perou CM, Sørlie T, Eisen MB, et al. Molecular portraits of human breast tumours. Nature. 2000;406(6797):747–752.
- Ioannidis JP, Allison DB, Ball CA, et al. Repeatability of published microarray gene expression analyses. Nat Genet. 2009;41(2):149–155.
- Stransky N, Egloff AM, Tward AD, et al. The mutational landscape of head and neck squamous cell carcinoma. Science. 2011;333(6046):1157–1160.
- Seiwert TY, Zuo Z, Keck MK, et al. Integrative and comparative genomic analysis of HPV-positive and HPV-negative head and neck squamous cell carcinomas. Clin Cancer Res. 2015;21(3):632–641.
- Network CGA. Comprehensive genomic characterization of head and neck squamous cell carcinomas. Nature. 2015;517(7536):576–582.
•• Important overview of the genomic landscape of head and neck squamous cell carcinoma (HNSCC)
- Califano J, van der Riet P, Westra W, et al. Genetic progression model for head and neck cancer: implications for field cancerization. Cancer Res. 1996;56(11):2488–2492.
• This landmark report discusses the first genetic progression model of HNSCC
- Agrawal N, Frederick MJ, Pickering CR, et al. Exome sequencing of head and neck squamous cell carcinoma reveals inactivating mutations in NOTCH1. Science. 2011;333(6046):1154–1157.
- Braakhuis BJ, Tabor MP, Kummer JA, et al. A genetic explanation of Slaughter’s concept of field cancerization: evidence and clinical implications. Cancer Res. 2003;63(8):1727–1730.
• Reviews the genetic explanation of field cancerization of head and neck cancer, and presents the earliest stages of carcinogenesis
- zur Rhausen H. Papillomaviruses and cancer: from basic studies to clinical application. Nat Rev Cancer. 2002;2(5):342–350.
- Hubbert NL, Sedman SA, Schiller JT. Human papillomavirus type 16 E6 increases the degradation rate of p53 in human keratinocytes. J Virol. 1992;66(10):6237–6241.
- Dyson N, Howley PM, Münger K, et al. The human papilloma virus-16 E7 oncoprotein is able to bind to the retinoblastoma gene product. Science. 1989;243(4893):934–937.
- Steenbergen RD, Snijders PJ, Heideman DA, et al. Clinical implications of (epi)genetic changes in HPV-induced cervical precancerous lesions. Nat Rev Cancer. 2014;14(6):395–405.
- Moody CA, Laimins LA. Human papillomavirus oncoproteins: pathways to transformation. Nat Rev Cancer. 2010;10(8):550–560.
- Bartkova J, Horejsí Z, Koed K, et al. DNA damage response as a candidate anti-cancer barrier in early human tumorigenesis. Nature. 2005;434(7035):864–870.
- Slaughter DP, Southwick HW, Smejkal W. Field cancerization in oral stratified squamous epithelium; clinical implications of multicentric origin. Cancer. 1953;6(5):963–968.
- Tabor MP, Brakenhoff RH, van Houten VM, et al. Persistence of genetically altered fields in head and neck cancer patients: biological and clinical implications. Clin Cancer Res. 2001;7(6):1523–1532.
• This research provided molecular evidence for the existence of premalignant changes in the mucosal epithelium surrounding the tumor, which explains the field cancerization concept and explains the frequent relapses in head and neck cancer patients treated with radical surgery
- van Houten VM, Tabor MP, van den Brekel MW, et al. Mutated p53 as a molecular marker for the diagnosis of head and neck cancer. J Pathol. 2002;198(4):476–486.
- Lim X, Tan SH, Koh WL, et al. Interfollicular epidermal stem cells self-renew via autocrine Wnt signaling. Science. 2013;342(6163):1226–1230.
- Rietbergen MM, Braakhuis BJ, Moukhtari N, et al. No evidence for active human papillomavirus (HPV) in fields surrounding HPV-positive oropharyngeal tumors. J Oral Pathol Med. 2014;43(2):137–142.
- McGovern SL, Williams MD, Weber RS, et al. Three synchronous HPV-associated squamous cell carcinomas of Waldeyer’s ring: case report and comparison with Slaughter’s model of field cancerization. Head Neck. 2010;32(8):1118–1124.
- de Bree R, Takes RP, Castelijns JA, et al. Advances in diagnostic modalities to detect occult lymph node metastases in head and neck squamous cell carcinoma. Head Neck. 2014;37(12):1829–1839.
- Kummer AJ, Nieuwenhuis EJC, Snow G, et al. New developments using non invasive techniques to assess the status of the neck in head and neck cancer patients. Curr Top Radiol. 2000;2(2):41–45.
- van den Brekel MW, Castelijns JA, Stel HV, et al. Occult metastatic neck disease: detection with US and US-guided fine-needle aspiration cytology. Radiology. 1991;180(2):457–461.
- Righi PD, Kopecky KK, Caldemeyer KS, et al. Comparison of ultrasound-fine needle aspiration and computed tomography in patients undergoing elective neck dissection. Head Neck. 1997;19(7):604–610.
- Takes RP, Righi P, Meeuwis CA, et al. The value of ultrasound with ultrasound-guided fine-needle aspiration biopsy compared to computed tomography in the detection of regional metastases in the clinically negative neck. Int J Radiat Oncol Biol Phys. 1998;40(5):1027–1032.
- Nieuwenhuis EJ, Jaspars LH, Castelijns JA, et al. Quantitative molecular detection of minimal residual head and neck cancer in lymph node aspirates. Clin Cancer Res. 2003;9(2):755–761.
- Ferris RL, Xi L, Raja S, et al. Molecular staging of cervical lymph nodes in squamous cell carcinoma of the head and neck. Cancer Res. 2005;65(6):2147–2156.
- Solassol J, Burcia V, Costes V, et al. Pemphigus vulgaris antigen mRNA quantification for the staging of sentinel lymph nodes in head and neck cancer. Br J Cancer. 2010;102(1):181–187.
- Broglie MA, Haile SR, Stoeckli SJ. Long-term experience in sentinel node biopsy for early oral and oropharyngeal squamous cell carcinoma. Ann Surg Oncol. 2011;18(10):2732–2738.
- Alkureishi LW, Ross GL, Shoaib T, et al. Sentinel node biopsy in head and neck squamous cell cancer: 5-year follow-up of a European multicenter trial. Ann Surg Oncol. 2010;17(9):2459–2464.
- Flach GB, Bloemena E, Klop WM, et al. Sentinel lymph node biopsy in clinically N0 T1-T2 staged oral cancer: the Dutch multicenter trial. Oral Oncol. 2014;50(10):1020–1024.
- Ferris RL, Stefanika P, Xi L, et al. Rapid molecular detection of metastatic head and neck squamous cell carcinoma as an intraoperative adjunct to sentinel lymph node biopsy. Laryngoscope. 2012;122(5):1020–1030.
- van ‘t Veer LJ, Dai H, van de Vijver MJ, et al, Gene expression profiling predicts clinical outcome of breast cancer. Nature. 2002;415(6871):530–536.
- Chung CH, Parker JS, Karaca G, et al. Molecular classification of head and neck squamous cell carcinomas using patterns of gene expression. Cancer Cell. 2004;5(5):489–500.
- O’Donnell RK, Kupferman M, Wei SJ, et al. Gene expression signature predicts lymphatic metastasis in squamous cell carcinoma of the oral cavity. Oncogene. 2005;24(7):1244–1251.
- Onken MD, Winkler AE, Kanchi KL, et al. A surprising cross-species conservation in the genomic landscape of mouse and human oral cancer identifies a transcriptional signature predicting metastatic disease. Clin Cancer Res. 2014;20(11):2873–2884.
- Warner GC, Reis PP, Jurisica I, et al. Molecular classification of oral cancer by cDNA microarrays identifies overexpressed genes correlated with nodal metastasis. Int J Cancer. 2004;110(6):857–868.
- Roepman P, Wessels LF, Kettelarij N, et al. An expression profile for diagnosis of lymph node metastases from primary head and neck squamous cell carcinomas. Nat Genet. 2005;37(2):182–186.
- Roepman P, Kemmeren P, Wessels LF, et al. Multiple robust signatures for detecting lymph node metastasis in head and neck cancer. Cancer Res. 2006;66(4):2361–2366.
- van Hooff SR, Leusink FK, Roepman P, et al. Validation of a gene expression signature for assessment of lymph node metastasis in oral squamous cell carcinoma. J Clin Oncol. 2012;30(33):4104–4110.
•• Multicenter validation of previously reported lymph node metastasis gene expression signature of HNSCC. The highest negative predictive value was found in the clinically relevant subgroup with early-stage disease.
- Leusink FK, van Es RJ, de Bree R, et al. Novel diagnostic modalities for assessment of the clinically node-negative neck in oral squamous-cell carcinoma. Lancet Oncol. 2012;13(12):e554–561.
- Vermorken JB, Specenier P. Optimal treatment for recurrent/metastatic head and neck cancer. Ann Oncol. 2010;21(Suppl 7):vii252–261.
- Ho AS, Kraus DH, Ganly I, et al. Decision making in the management of recurrent head and neck cancer. Head Neck. 2014;36(1):144–151.
- Takes RP, Rinaldo A, Silver CE, et al. Distant metastases from head and neck squamous cell carcinoma. Part I. Basic aspects. Oral Oncol. 2012;48(9):775–779.
- Brennan JA, Mao L, Hruban RH, et al. Molecular assessment of histopathological staging in squamous-cell carcinoma of the head and neck. N Engl J Med. 1995;332(7):429–435.
- van Houten VM, Leemans CR, Kummer JA, et al. Molecular diagnosis of surgical margins and local recurrence in head and neck cancer patients: a prospective study. Clin Cancer Res. 2004;10(11):3614–3620.
- Poeta ML, Manola J, Goldenberg D, et al. The Ligamp TP53 Assay for Detection of Minimal Residual Disease in Head and Neck Squamous Cell Carcinoma Surgical Margins. Clin Cancer Res. 2009;15(24):7658–7665.
- Shaw RJ, Hall GL, Woolgar JA, et al. Quantitative methylation analysis of resection margins and lymph nodes in oral squamous cell carcinoma. Br J Oral Maxillofac Surg. 2007;45(8):617–622.
- Temam S, Casiraghi O, Lahaye JB, et al. Tetranucleotide microsatellite instability in surgical margins for prediction of local recurrence of head and neck squamous cell carcinoma. Clin Cancer Res. 2004;10(12 Pt 1):4022–4028.
- Colnot DR, Nieuwenhuis EJ, Kuik DJ, et al. Clinical significance of micrometastatic cells detected by E48 (Ly-6D) reverse transcription-polymerase chain reaction in bone marrow of head and neck cancer patients. Clin Cancer Res. 2004;10(23):7827–7833.
- Graveland AP, Braakhuis BJ, Eerenstein SE, et al. Molecular diagnosis of minimal residual disease in head and neck cancer patients. Cell Oncol (Dordr). 2012;35(5):367–375.
- Roh JL, Westra WH, Califano JA, et al. Tissue imprint for molecular mapping of deep surgical margins in patients with head and neck squamous cell carcinoma. Head Neck. 2012;34(11):1529–1536.
- Salvi D, Picone M, Arredondo MT, et al. Merging person-specific bio-markers for predicting oral cancer recurrence through an ontology. IEEE Trans Biomed Eng. 2013;60(1):216–220.
- Chung CH, Parker JS, Ely K, et al. Gene expression profiles identify epithelial-to-mesenchymal transition and activation of nuclear factor-kappaB signaling as characteristics of a high-risk head and neck squamous cell carcinoma. Cancer Res. 2006;66(16):8210–8218.
- Nawroz H, Koch W, Anker P, et al. Microsatellite alterations in serum DNA of head and neck cancer patients. Nat Med. 1996;2(9):1035–1037.
• First report on the detection of genetic alteration in serum of HNSCC patients by microsatellite analysis
- Bettegowda C, Sausen M, Leary RJ, et al. Detection of circulating tumor DNA in early- and late-stage human malignancies. Sci Transl Med. 2014;6(224):224ra224.
- Wang Y, Springer S, Mulvey CL, et al. Detection of somatic mutations and HPV in the saliva and plasma of patients with head and neck squamous cell carcinomas. Sci Transl Med. 2015;7(293):293ra104.
• Focuses on the detection of cell-free tumor DNA in saliva and plasma of HNSCC patients. Importantly, tumor DNA is also detected in a significant subset of early-stage tumors
- Mao L, Lee JS, Fan YH, et al. Frequent microsatellite alterations at chromosomes 9p21 and 3p14 in oral premalignant lesions and their value in cancer risk assessment. Nat Med. 1996;2(6):682–685.
- Partridge M, Pateromichelakis S, Phillips E, et al. A case-control study confirms that microsatellite assay can identify patients at risk of developing oral squamous cell carcinoma within a field of cancerization. Cancer Res. 2000;60(14):3893–3898.
- Rosin MP, Cheng X, Poh C, et al. Use of allelic loss to predict malignant risk for low-grade oral epithelial dysplasia. Clin Cancer Res. 2000;6(2):357–362.
- Graveland AP, Golusinski PJ, Buijze M, et al. Loss of heterozygosity at 9p and p53 immunopositivity in surgical margins predict local relapse in head and neck squamous cell carcinoma. Int J Cancer. 2011;128(8):1852–1859.
- Zhang L, Poh CF, Williams M, et al. Loss of heterozygosity (LOH) profiles–validated risk predictors for progression to oral cancer. Cancer Prev Res (Phila). 2012;5(9):1081–1089.
- Graveland AP, Bremmer JF, de Maaker M, et al. Molecular screening of oral precancer. Oral Oncol. 2013;49(12):1129–1135.
- van der Waal I. Potentially malignant disorders of the oral and oropharyngeal mucosa; present concepts of management. Oral Oncol. 2010;46(6):423–425.
- Bremmer JF, Graveland AP, Brink A, et al. Screening for oral precancer with noninvasive genetic cytology. Cancer Prev Res (Phila). 2009;2(2):128–133.
- Poh CF, Zhang L, Anderson DW, et al. Fluorescence visualization detection of field alterations in tumor margins of oral cancer patients. Clin Cancer Res. 2006;12(22):6716–6722.
- Poh CF, MacAulay CE, Zhang L. Rosin MP. Tracing the “at-risk” oral mucosa field with autofluorescence: steps toward clinical impact. Cancer Prev Res (Phila). 2009;2(5):401–404.
- Poh CF, Durham JS, Brasher PM, et al. Canadian Optically-guided approach for Oral Lesions Surgical (COOLS) trial: study protocol for a randomized controlled trial. BMC Cancer. 2011;11:462.
- van Houten VM, Snijders PJ, van den Brekel MW, et al. Biological evidence that human papillomaviruses are etiologically involved in a subgroup of head and neck squamous cell carcinomas. Int J Cancer. 2001;93(2):232–235.
- Wiest T, Schwarz E, Enders C, et al. Involvement of intact HPV16 E6/E7 gene expression in head and neck cancers with unaltered p53 status and perturbed pRb cell cycle control. Oncogene. 2002;21(10):1510–1517.
- Smeets SJ, Braakhuis BJ, Abbas S, et al. Genome-wide DNA copy number alterations in head and neck squamous cell carcinomas with or without oncogene-expressing human papillomavirus. Oncogene. 2006;25(17):2558–2564.
- Slebos RJ, Yi Y, Ely K, et al. Gene expression differences associated with human papillomavirus status in head and neck squamous cell carcinoma. Clin Cancer Res. 2006;12(3 Pt 1):701–709.
- Lleras RA, Smith RV, Adrien LR, et al. Unique DNA methylation loci distinguish anatomic site and HPV status in head and neck squamous cell carcinoma. Clin Cancer Res. 2013;19(19):5444–5455.
- Kostareli E, Holzinger D, Bogatyrova O, et al. HPV-related methylation signature predicts survival in oropharyngeal squamous cell carcinomas. J Clin Invest. 2013;123(6):2488–2501.
- Snijders PJ, van den Brule AJ, Meijer CJ. The clinical relevance of human papillomavirus testing: relationship between analytical and clinical sensitivity. J Pathol. 2003;201(1):1–6.
- Schache AG, Liloglou T, Risk JM, et al. Evaluation of human papilloma virus diagnostic testing in oropharyngeal squamous cell carcinoma: sensitivity, specificity, and prognostic discrimination. Clin Cancer Res. 2011;17(19):6262–6271.
- Smeets SJ, Hesselink AT, Speel EJ, et al. A novel algorithm for reliable detection of human papillomavirus in paraffin embedded head and neck cancer specimen. Int J Cancer. 2007;121(11):2465–2472.
- Weinberger PM, Yu Z, Haffty BG, et al. Molecular classification identifies a subset of human papillomavirus–associated oropharyngeal cancers with favorable prognosis. J Clin Oncol. 2006;24(5):736–747.
- Lingen MW, Xiao W, Schmitt A, et al. Low etiologic fraction for high-risk human papillomavirus in oral cavity squamous cell carcinomas. Oral Oncol. 2013;49(1):1–8.
- Smeets SJ, Brakenhoff RH, Ylstra B, et al. Genetic classification of oral and oropharyngeal carcinomas identifies subgroups with a different prognosis. Cell Oncol. 2009;31(4):291–300.
- Walter V, Yin X, Wilkerson MD, et al. Molecular subtypes in head and neck cancer exhibit distinct patterns of chromosomal gain and loss of canonical cancer genes. PLoS One. 2013;8(2):e56823.
- De Cecco L, Nicolau M, Giannoccaro M, et al. Head and neck cancer subtypes with biological and clinical relevance: Meta-analysis of gene-expression data. Oncotarget. 2015;6(11):9627–9642.
- Keck MK, Zuo Z, Khattri A, et al. Integrative analysis of head and neck cancer identifies two biologically distinct HPV and three non-HPV subtypes. Clin Cancer Res. 2015;21(4):870–881.
- Sørlie T, Perou CM, Tibshirani R, et al. Gene expression patterns of breast carcinomas distinguish tumor subclasses with clinical implications. Proc Natl Acad Sci U S A. 2001;98(19):10869–10874.
- Sorlie T, Tibshirani R, Parker J, et al. Repeated observation of breast tumor subtypes in independent gene expression data sets. Proc Natl Acad Sci U S A. 2003;100(14):8418–8423.
- Fan C, Oh DS, Wessels L, et al. Concordance among gene-expression-based predictors for breast cancer. N Engl J Med. 2006;355(6):560–569.
- Carey LA, Perou CM, Livasy CA, et al. Race, breast cancer subtypes, and survival in the Carolina Breast Cancer Study. Jama. 2006;295(21):2492–2502.
- O’Sullivan B, Huang SH, Siu LL, et al. Deintensification candidate subgroups in human papillomavirus-related oropharyngeal cancer according to minimal risk of distant metastasis. J Clin Oncol. 2013;31(5):543–550.
- Rios Velazquez E, Hoebers F, Aerts HJ, et al. Externally validated HPV-based prognostic nomogram for oropharyngeal carcinoma patients yields more accurate predictions than TNM staging. Radiother Oncol. 2014;113(3):324–330.
- Oguejiofor K, Hall J, Slater C, et al. Stromal infiltration of CD8 T cells is associated with improved clinical outcome in HPV-positive oropharyngeal squamous carcinoma. Br J Cancer. 2015;113(6):886–893.
- Ward MJ, Thirdborough SM, Mellows T, et al. Tumour-infiltrating lymphocytes predict for outcome in HPV-positive oropharyngeal cancer. Br J Cancer. 2014;110(2):489–500.
- Näsman A, Andersson E, Marklund L, et al. HLA class I and II expression in oropharyngeal squamous cell carcinoma in relation to tumor HPV status and clinical outcome. PLoS One. 2013;8(10):e77025.
- Rietbergen MM, Martens-de Kemp SR, Bloemena E, et al. Cancer stem cell enrichment marker CD98: a prognostic factor for survival in patients with human papillomavirus-positive oropharyngeal cancer. Eur J Cancer. 2014;50(4):765–773.
- De Cecco L, Bossi P, Locati L, et al. Comprehensive gene expression meta-analysis of head and neck squamous cell carcinoma microarray data defines a robust survival predictor. Ann Oncol. 2014;25(8):1628–1635.
- Rickman DS, Millon R, De Reynies A, et al. Prediction of future metastasis and molecular characterization of head and neck squamous-cell carcinoma based on transcriptome and genome analysis by microarrays. Oncogene. 2008;27(51):6607–6622.
- Lohavanichbutr P, Méndez E, Holsinger FC, et al. A 13-gene signature prognostic of HPV-negative OSCC: discovery and external validation. Clin Cancer Res. 2013;19(5):1197–1203.
- Thompson CF, St John MA, Lawson G, et al. Diagnostic value of sentinel lymph node biopsy in head and neck cancer: a meta-analysis. Eur Arch Otorhinolaryngol. 2013;270(7):2115–2122.