Abstract
Tuberculosis (TB) is one of the world’s most important infectious causes of morbidity and mortality among adults. Between 8 and 9 million develop TB disease, and approximately 2 million die from TB each year. Despite this enormous global burden, case detection rates are low, posing major hurdles for TB control. Conventional TB diagnosis continues to rely on smear microscopy, culture and chest radiography. These tests have known limitations. Conventional tests for detection of drug resistance are slow, tedious and difficult to perform in field conditions. This second half of a two-part review series on new tools for TB diagnosis describes recent advances and emerging technologies in the diagnosis of active disease, and detection of drug resistance. For diagnosis, new tools include newer versions of nucleic acid amplification tests, immune-based assays, skin patch test and rapid culture systems. For drug resistance, new tools include line-probe assays, bacteriophage-based assays, molecular beacons and microscopic observation drug susceptibility assay. Although the ideal test for TB is still not in sight, substantial progress has been made in the past decade. With the resurgence of interest in the development of new tools for TB control, it is likely that the next decade will see greater progress and tangible benefits. However, the challenge will be to ensure that new tools undergo rigorous evaluations in field conditions, and also to make sure that benefits of promising new tools actually reach the populations in developing countries that need them most. Latent TB is discussed in Part I; 413–422 of this issue.
LAMP is characterized by its use of four different primers specifically designed to recognize six distinct regions on the target gene, and its process being performed at a constant temperature using a strand displacement reaction. Image courtesy of Eiken Genome Site, Eiken Chemical Co., Ltd (Tokyo, Japan) and reproduced with permission.
LAMP: Loop-mediated isothermal amplification.
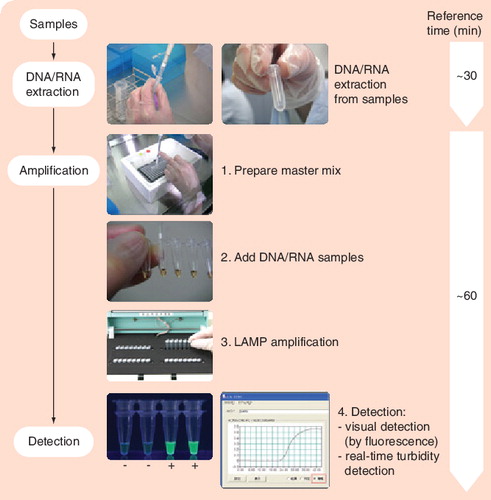
(A) An aqueous solution containing MPB64 protein is applied to the gauze of the Torii patch, and the patch is then immediately applied to the forearm. After 48–72 h, the patch is removed and the response assessed. (B) A positive patch test result. Image courtesy of Sequella, Inc. (MD, USA) and reproduced with permission.
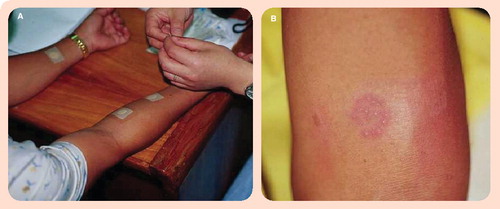
Mycobacterial growth changes the color of the medium from red to yellow. Common contaminants, such as fungi or Gram-negative bacteria, change the color from red to green. Image courtesy of Salubris, Inc. (MA, USA) and reproduced with permission.
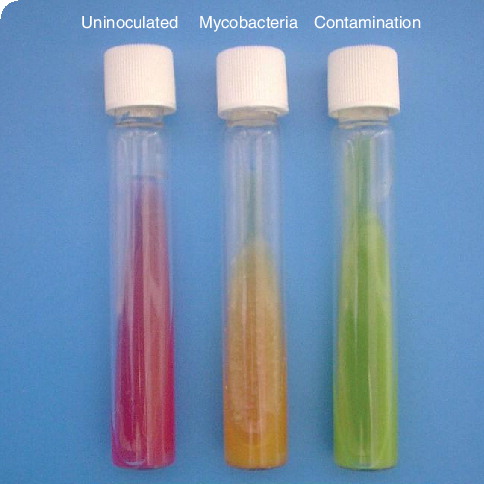
(A) Principle of reverse hybridization. (B) INNO-LiPA® assay. The INNO-LiPA test contains ten oligonucleotide probes (one specific for the Mycobacterium tuberculosis complex, five overlapping wild-type S probes, and four R probes for detecting specific mutations) that are immobilized on nitrocellulose strips. LiPA is performed by extracting DNA and amplifying the rifampicin resistance-determining region of the rpoB gene using PCR. The PCR products are then hybridized with the immobilized probes, and results are determined by colorimetric development. Image adapted from Innogenetics NV (Gent, Belgium) © 2006 Innogenetics Group.
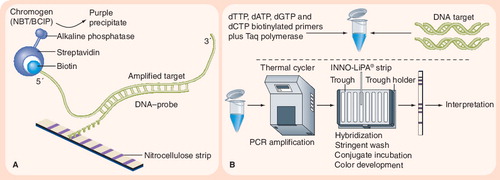
The underlying principle is the amplification of bacteriophages after their infection of M. tuberculosis, followed by detection of progeny phages as lytic plaques on a lawn of Mycobacterium smegmatis. Image courtesy of Biotec Laboratories Ltd (Ipswich, UK), and adapted and reproduced with permission.
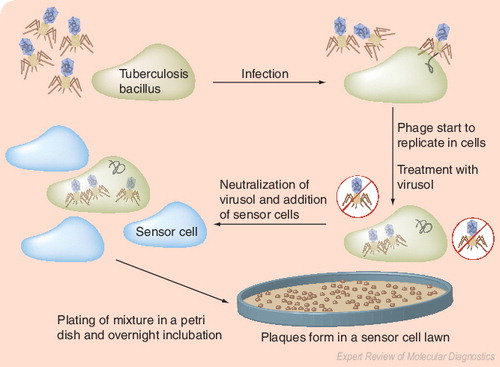
The circular disposable is inoculated in the center slot, and is then sealed for the rest of the process. The sample moves into the first six wells, which contain the appropriate antibiotic or control. Using the centrifuge integrated into the main unit, the sample is then moved to the next ring of six wells, which contain luciferase reporter phage. The phage are given time to infect any living mycobacteria, and the sample is then moved into the next ring of six wells, which contain luciferin. Phages that infect living mycobacteria will produce light, which is detected and analyzed by the main Bronx box unit. Image courtesy of Sequella, Inc. (MD, USA) and reproduced with permission.
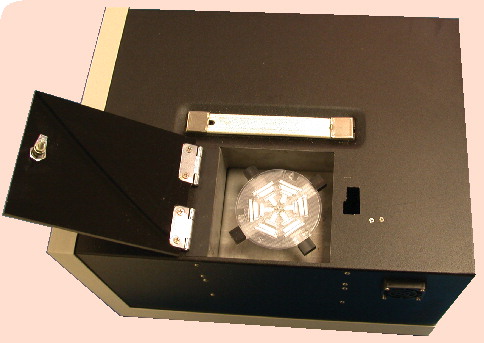
Beacons are single-stranded oligonucleotide hybridization probes that form a stem-and-loop structure. In the absence of the target sequence, the fluorophore and quencher are closely bound, and this prevents fluorescence. When the target sequence is present, hybridization of the probe (loop) with the target results in dissociation of the fluorophore and quencher, thereby restoring fluorescence. Adapted from a figure provided by Sanjay Tyagi.
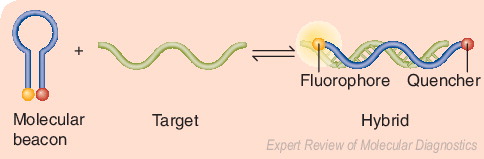
Characteristic tangling appearance of cords of Mycobacterium tuberculosis as visualized at ×20 magnification under an inverted light microscope. Image courtesy of David AJ Moore and Luz Caviedes and reproduced with permission.
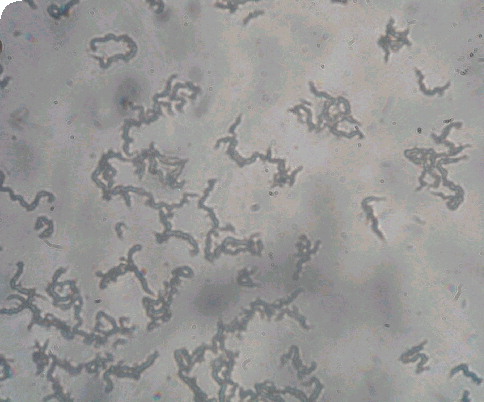
Tuberculosis (TB) is one of the world’s most important infectious causes of morbidity and mortality among adults, infecting over 2 billion people. Between 8 and 9 million people develop TB, and approximately 2 million die from TB each year Citation[1,2]. Despite this enormous global burden, case detection rates are low, posing major hurdles for TB control. Conventional TB diagnosis continues to rely on sputum smear microscopy, mycobacterial culture and chest radiography. These tests have several limitations Citation[3–5]. Conventional tests for detection of drug resistance are slow, complicated, expensive and difficult to perform in field conditions Citation[6]. The long-standing need for new, rapid, accurate and convenient tests for TB diagnosis and drug resistance is currently being addressed by several agencies and groups Citation[101–105]. In this second half of a two-part review series on new tools for TB diagnosis, the authors describe recent advances and emerging technologies in the diagnosis of active disease, and detection of drug resistance.
Diagnostics for active tuberculosis
Nucleic acid amplification tests
Nucleic acid amplification tests (NATs), also called direct amplification tests, are designed to amplify nucleic acid regions specific to the Mycobacterium tuberculosis complex. These tests can be used directly on clinical specimens (e.g., sputum). NATs are available as commercial kits. They are also widely used as in-house assays. Commercial kits include the Amplified™ M. tuberculosis Direct Test® (MTD; Gen-Probe Inc., CA, USA Citation[106]), the Amplicor®M. tuberculosis (MTB) tests (Roche Molecular Diagnostics, CA, USA Citation[107]), and the BD ProbeTec™ ET assay (BD Biosciences, MD, USA Citation[108]). The Amplicor and MTD tests are currently US FDA approved. In-house tests are laboratory-developed PCR assays, and they vary widely in their protocols and laboratory methods. In-house NATs tend to be used predominantly in research settings and in developing countries where commercial NATs are expensive.
The literature on NAT has been extensively reviewed Citation[7–9]. Several recent meta-analyses have synthesized the evidence on accuracy, and the results demonstrate that NATs have high specificity for both pulmonary and extrapulmonary TB (but data on extrapulmonary TB are relatively limited) Citation[10–14]. High specificity and positive-predictive value confer clear advantages in terms of the test’s ability to rule-in TB. A positive NAT in a patient with a high pretest probability is fairly confirmatory of TB, particularly in those with positive smears. Therefore, NAT can be used to confirm that a sputum smear with acid-fast bacilli is due to M. tuberculosisCitation[7]. However, this approach is largely unnecessary in high-burden countries where TB is treated mainly on the basis of positive sputum smears.
In contrast to specificity, sensitivity of NAT is lower and highly variable across studies. In many studies, sensitivity estimates have been lower in paucibacillary forms of TB (extrapulmonary TB and smear-negative pulmonary disease). Sensitivity of NAT has been highest in smear-positive specimens. Therefore, a negative test does not rule-out the diagnosis of TB. Therefore, NAT should not be performed if the smears are negative and if the index of clinical suspicion is low. A negative NAT in a patient with a high index of clinical suspicion should prompt continued work-up.
In general, the accuracy of in-house PCR tests has been more heterogeneous than commercial kits. Recently, Flores and colleagues published a meta-regression analysis on factors associated with heterogeneity in in-house PCR accuracy Citation[10]. The use of IS6110 as an amplification target and the use of nested PCR were associated with higher accuracy Citation[10]. However, the substantial heterogeneity in both sensitivity and specificity of the in-house NAT rendered clinically useful estimates of test accuracy difficult. On the other hand, commercial assays are standardized and appear to produce results that are more consistent.
In summary, available NATs have not realised their early promise. In addition to concerns about accuracy and reliability, their high costs and requirement for laboratory infrastructure reduce their applicability in resource-limited settings. Therefore, efforts are underway to simplify testing protocols and increase their accuracy. Loop-mediated isothermal amplification (LAMP) is one such development.
Loop-mediated isothermal amplification
LAMP is a novel, rapid and simplified NAT platform developed by Eiken Chemical Co., Ltd (Tokyo, Japan) Citation[109]. The platform is not specific to TB and has been used to detect other organisms. Its major advantages are speed, simplicity and the lack of requirement for a thermal cycler, thus facilitating high throughput. The technology uses four different primers specifically designed to recognize six distinct regions on the target gene, and the reaction process proceeds at a constant temperature (isothermal) using strand displacement reaction Citation[109]. Amplification and detection of the gene products can be completed in a single step, by incubating the mixture of samples, primers, DNA polymerase with strand-displacement activity and substrates at a constant temperature. The amplification efficiency is high, and DNA can be amplified 109–1010 times in 15–60 min Citation[109]. Due to its high specificity, the presence of amplified product can indicate the presence of target gene. Currently, there is limited evidence on the accuracy of LAMP for the detection of TB Citation[15]. Studies are underway to define the test performance and compare its performance against conventional tests.
Immunological tests, including serological assays
Immune-based tests for the detection of antibodies, antigens and immune complexes have been attempted for decades, and their performance has been extensively reviewed Citation[16–20]. These assays generally detect humoral immune response, as opposed to T-cell-based assays that detect cellular immunity (e.g., interferon-γ assays). Dozens of commercial kits are available, which are mostly focused on antibody detection. Despite the long history of serological tests and persistent attempts at improving them, no assay is currently accurate enough to replace microscopy and culture. Despite the evidence on lack of accuracy, serological tests continue to be marketed and used, mostly in developing countries.
Several authors have attempted to analyze the reasons for the apparent failure of serological tests Citation[16,20–22]. A major challenge with immunological diagnosis of TB is that TB presents a wide spectrum ranging from exposure, through latent TB infection (LTBI) and active disease, to severe disease Citation[20,22]. Furthermore, several other factors can affect the performance of immune-based tests: BCG vaccination, exposure to nontuberculous mycobacteria (NTM), M. tuberculosis strain and HIV co-infection. A good immunological test must distinguish between the various states of TB, and also distinguish between TB and other mycobacterial exposures. Early versions of serological assays used crude, nonspecific antigenic mixtures Citation[20]. This led to loss of specificity, and the inability to distinguish TB from LTBI and NTM Citation[20]. The development of well-defined, purified recombinant proteins specific to M. tuberculosis partially overcame this problem.
Antigen recognition in TB is broad and highly variable, and varies across the infection/disease spectrum Citation[16,20–22]. M. tuberculosis expresses different genes and proteins during different stages of the disease Citation[16,20–22]. For example, proteins expressed during latency may differ from those expressed during active disease. Even within active disease, proteins expressed in cavitary disease may differ from those expressed in noncavitary disease. Therefore, any test that detects immune responses must include antigens that are expressed during the various stages of disease progression. However, many serological assays use single antigens (e.g., 38-kDa protein) that may not be recognized by the host immune system during all stages. The development of cocktails of multiple specific antigens may partially address this problem Citation[16,20–22].
Currently available serological assays are based on identifying markers positively associated with a particular state (e.g., active disease). This approach may be limited because antibody profiles associated with inactive TB may differ from those associated with active disease Citation[21,22]. It has been suggested that each TB state is characterized by bacterial antigen signatures that resemble barcodes Citation[21,22]. In other words, certain combinations of the presence and absence of antigen-specific immune responses may indicate specific disease states. Therefore, it may be necessary to study negative as well as positive responses to a panel of antigens. A pattern of positive and negative responses (analogous to a barcode) may then be used to distinguish between various stages of TB infection and disease Citation[21,22]. Whether this approach has practical, clinical applicability has not been determined to date.
In addition to these approaches, efforts are also underway to develop immune-based assays that focus on antigen rather than antibody detection. For example, antigen-capture enzyme-linked immunosorbent assays for the detection of lipoarabinomannan in sputum Citation[23] and urine Citation[24] samples have demonstrated promise in early trials. Further work is necessary to determine their utility.
Overall, the lessons learnt from previous failures should facilitate the development of a new generation of immune-based tests, including ones that detect antigens and/or circulating immune complexes rather than antibodies, which will be sensitive and specific, and will be able to distinguish between latent and active TB. Such assays, if shown to be accurate, will have an impact on TB case finding, especially if they can be packaged as point-of-care strip tests that may be used in primary care settings.
MPB64 skin patch test for active tuberculosis
MPB64 is an immunogenic antigen specific to M. tuberculosis complex Citation[25]. It is secreted by M. tuberculosis, Mycobacterium bovis, and some strains of M. bovis BCG. In 1998, Nakamura and colleagues demonstrated that a skin patch with MPB64 antigen was capable of eliciting a distinctive response in individuals with active, but not latent, TB Citation[26]. In studies conducted in Japan and the Philippines, the MPB64 patch test was able to distinguish active TB from LTBI with 98% sensitivity and 100% specificity Citation[26]. In an another study in the Philippines, Nakamura and coworkers demonstrated similar results, with a sensitivity of 88% and specificity of 100% for active TB Citation[27]. However, the exact biological mechanism behind the skin response in these studies is still unclear. The skin patch test is currently being developed into a commercial test by Sequella, Inc., (MD, USA) Citation[110]. Using recombinant rMPT64, the test is being evaluated in trials to establish the accuracy, dose ranges and other operational characteristics (e.g., ability to read results in dark-skinned individuals) Citation[28]. Since the patch test is simple, noninvasive and does not require a laboratory or highly skilled personnel, it has the potential to make an impact, especially if it is shown to be accurate, unaffected by anergy (e.g., due to HIV) and capable of distinguishing between LTBI and active disease in high-burden countries.
Rapid culture systems: TK Medium
TK Medium® (Salubris, Inc., MA, USA) is a novel colorimetric system that indicates growth of mycobacteria by changing its color Citation[111]. Metabolic activity of growing mycobacteria changes the color of the culture medium, and this enables an early positive identification before bacterial colonies appear. As illustrated in , the test can distinguish between mycobacteria and contamination. TK Medium also enables susceptibility testing for drug resistance, and can allow for differentiation between M. tuberculosis and NTM. Although TK Medium promises to be a practical, low-cost, simple test, published evidence on this test is limited Citation[29,30]. The test is not currently FDA approved. Data from a multicentric study in Turkey demonstrated that TK Medium’s sensitivity for mycobacterial detection was comparable to that of the Lowenstein–Jensen (LJ) medium Citation[30]. The average time to detection with TK Medium was 2 weeks, as compared with 4 weeks with the conventional LJ medium. Larger multicentric studies are ongoing, and they should help to define the accuracy of this new rapid culture system. In addition to accuracy, it is particularly important to study practical issues, such as contamination, quality control and training requirements, under field conditions.
Rapid detection of drug resistance
Line-probe assays
Line-probe assays are a family of novel DNA strip-based tests that use PCR and reverse hybridization methods for the rapid detection of mutations associated with drug resistance . Commercially available line-probe assays include the INNO-LiPA® Rif. TB kit (Innogenetics NV, Gent, Belgium) Citation[112], and the GenoType® MTBDR assay (Hain Lifescience GmbH, Nehren, Germany) Citation[113]. These kits are not currently FDA approved for use in the USA, but are CE marked. Line-probe assays are designed to identify M. tuberculosis complex and simultaneously detect mutations associated with drug resistance.
In a recent meta-analysis, Morgan and colleagues synthesized the evidence on line-probe assay from several studies on its accuracy for rifampicin resistance Citation[31]. This meta-analysis demonstrated that line-probe assay has high sensitivity and specificity when culture isolates are used. The majority of studies had sensitivity of 95% or greater, and nearly all were 100% specific. However, the results are less accurate when the test is directly applied on clinical specimens (e.g., sputum). There is a paucity of data on the application of this test directly to clinical specimens. If further studies indicate that line-probe assays consistently and accurately detect rifampicin resistance when applied directly to clinical specimens, it could be a useful test in select patient populations in which drug resistance is strongly suspected. The GenoType MTBDR assay also appears to be promising, but published data are limited Citation[32,33].
In general, line-probe assays are expensive and require sophisticated laboratory infrastructure. Their role and utility in low-income, high-burden countries will be limited unless cost and technical issues are addressed.
Bacteriophage-based assays
Mycobacteriophage-based tests have been evaluated for diagnosis of TB Citation[34], as well as drug susceptibility testing Citation[35]. The technology uses bacteriophages to infect live M. tuberculosis and detect the bacilli using either phage-amplification method or detection of light. In the first method, the underlying principle is amplification of phages after their infection of M. tuberculosis, followed by detection of progeny phages as plaques on a lawn of Mycobacterium smegmatisCitation[36]. In the second method, the principle is detection of light (using luminometry or photography) produced by luciferase reporter phages after their infection of live M. tuberculosisCitation[37]. Amplification-based assays are commercially available, whereas luciferase reporter phage tests are under commercial development (see ‘Bronx box®’). In general, phage assays have a turnaround time of 2–3 days, and require a laboratory infrastructure similar to that required for performing mycobacterial cultures.
Phage amplification assays are available as commercial kits. For diagnosis, the FASTPlaque-TB™ (Biotec Laboratories Ltd, Ipswich, UK) assay can be directly used on sputum specimens Citation[114]. A variant, the FASTPlaque-TB-MDRi™ kit is designed to detect rifampicin resistance in culture isolates. A new version of this kit, FASTPlaque-TB-Response™, has been developed for direct use on sputum specimens Citation[114]. Drug resistance is diagnosed when M. tuberculosis is detected in samples that contain the drug (e.g., rifampicin). The FASTPlaque-TB kits are not currently FDA approved, but are CE marked for use in Europe.
The accuracy of phage assays (amplification as well as luciferase phage assays) for detection of rifampicin resistance has been synthesized in a recent meta-analysis Citation[35]. The results demonstrated that, when performed on culture isolates, phage assays have relatively high accuracy. A total of 11 out of 19 (58%) studies included in the review reported sensitivity and specificity estimates of at least 95%. Specificity estimates were slightly lower and more variable than sensitivity; five out of 19 (26%) studies reported specificity under 90%. Only two studies performed phage assays directly on sputum specimens, with inconsistent results. Therefore, current evidence is mostly restricted to the use of phage assays for the detection of rifampicin resistance in culture isolates. When applied to isolates, these assays have relatively high sensitivity and specificity. However, the requirement for primary culture isolation somewhat reduces the rapidity of this assay. When applied directly to clinical specimens, phage assays appear to have lower sensitivity, presumably due to the lower bacillary load Citation[34].
If phage-based assays can be directly used on clinical specimens, and if they are shown to be accurate, they have the potential to improve the diagnosis of drug-resistant TB Citation[35]. Efforts are ongoing to improve their performance characteristics for the direct detection of rifampicin resistance in sputum specimens Citation[38]. Preliminary results on the improved, direct phage assay demonstrate promise, but require further validation and field testing in high-burden settings Citation[39].
Bronx box®
A luciferase reporter phage-based test called the Bronx box is currently being developed as a commercial kit by Sequella, Inc. Citation[110]. In contrast to the research version of the Bronx box that uses a photographic plate to detect light produced by luciferase reporter phages, the commercial version is a semiautomated, digital version Citation[110]. Published data on the research version of the Bronx box are limited, but show promise Citation[40,41]. No data exist on the accuracy of the commercial version, but field studies are underway.
Molecular beacon assays
Molecular beacons are single-stranded oligonucleotide hybridization probes that form a stem-and-loop structure Citation[115]. The loop contains a probe sequence that is complementary to a target sequence of interest (e.g., a wild-type or mutation-containing sequence), and the stem is formed by the annealing of complementary arm sequences that are located on either side of the probe sequence. A fluorophore moiety is linked to the end of one arm, and a nonfluorescent quencher moiety is linked to the end of the other arm. As illustrated in , when the target sequence is absent, the probe cannot fluoresce, because the stem places the fluorophore very close to the quencher. When the target sequence is present, the probe and the target hybridize, and the beacon undergoes a spontaneous conformational change that forces the fluorophore and the quencher to dissociate and move away from each other, causing fluorescence that can be detected in a real-time PCR assay Citation[115]. Beacons are highly specific, and can discriminate target sequences that differ from one another by a single nucleotide substitution. They can also be used for diseases other than TB Citation[115].
Recently, novel tests that use beacons for the rapid detection of mutations associated with drug resistance have been evaluated Citation[42–46]. These studies suggest that these tests have high sensitivity (89–98%) and specificity (99–100%) for the detection of mutations associated with rifampicin resistance Citation[42–45]. Sensitivity for the detection of isoniazid resistance appears to be relatively lower Citation[43,44]. Although the test has been used in public health settings Citation[46], beacon assays are not currently FDA approved for clinical use, and they are not commercially available. Simplification of the technology and commercial development is needed to enhance the applicability of this novel tool. Currently, technological requirements and cost of the beacon assays limit their applicability in developing countries.
Microscopic observation drug-susceptibility assay
Microscopic observation drug-susceptibility assay (MODS) is a novel assay that uses an inverted light microscope and Middlebrook 7H9 broth culture to rapidly detect mycobacterial growth Citation[47,48]. MODS uses simple light microscopy to detect early growth of M. tuberculosis as strings and tangles of bacterial cells in the broth medium with or without antimicrobial drugs (for drug susceptibility testing) Citation[47,48]. In a recent, large study from Peru, MODS detected 94% of 1908 positive sputum cultures, whereas the conventional LJ culture detected only 87% Citation[48]. MODS also had a shorter time to culture positivity (average of 8 days) compared with LJ culture. The results obtained by direct susceptibility testing for isoniazid and rifampin using MODS were available at the same time as TB detection, and had high agreement with the results obtained by indirect colorimetric methods.
Overall, MODS appears to be a promising, novel, inexpensive tool that can rapidly detect TB and drug resistance directly from sputum specimens. Multicentric studies are ongoing to evaluate this assay, which may have applicability in high-burden, resource-limited settings.
Expert commentary & five-year view
Despite the enormous global burden of TB, case detection rates continue to be low, jeopardizing global TB control efforts, particularly in areas with high HIV prevalence. Presently, TB diagnosis relies on old tools with known limitations. The long-felt need for new tools is currently being addressed by several global health agencies, nonprofit groups, industry, funding agencies, public–private partnerships and academic institutions Citation[101–105].
For diagnosis of active TB, several new tools are in the pipeline, including newer immune-based assays, simplified NAT, skin patch test and rapid, simplified culture systems. Some of these tests may become commercially available within the next 5–10 years. While existing serological assays have not been shown to be highly accurate, there is a definite need to continue development and refinement of newer versions. Similarly, the known limitations of existing NAT assays should be overcome with newer, simplified versions.
With respect to detection of drug resistance, several accurate molecular tests (e.g., line-probe assay, molecular beacon and NAT) are already available. Efforts are needed to reduce their cost and simplify them for resource-limited settings. Novel, relatively simple phenotypic tests (e.g., MODS, TK Medium, Bronx box and FASTPlaque-TB-Response) are also under development, and it is important to evaluate their performance against molecular and conventional tests. These simpler phenotypic tests may have a greater impact in resource-limited settings than molecular tools.
One concern with the development of new tools is that they may involve expensive technologies beyond the reach of developing countries affected by the dual TB/HIV epidemics. High-tech tools are unlikely to make an impact in these countries, even if they are accurate. By contrast, simpler, developing world-friendly formats, such as skin tests (e.g., skin patch test), rapid, point-of-care immunodiagnostics (e.g., lateral flow assays), innovative use of microscopy (e.g., MODS) and rapid, simplified cultures (e.g., TK Medium), might have greater applicability.
Another concern with new tool development is that these tests often perform well in early controlled trials, but fail in routine practice Citation[49]. Lack of rigor in TB diagnostic studies is an important concern that must be addressed Citation[49]. All of the emerging technologies should initially undergo rigorous clinical trials, and, if found to be accurate, undergo demonstration projects in the field, preferably in settings with high TB/HIV incidence, before their introduction into practice. Promising tests should also undergo review by regulatory agencies prior to marketing Citation[49].
In conclusion, although the ideal tests for TB diagnosis and drug resistance are still not in sight, substantial progress has been made in the past decade. With the resurgence of interest in the development of new tools for TB, the next decade should bring tangible benefits Citation[50]. However, the challenge will be to ensure that new tools undergo rigorous evaluations in field conditions, and also to make sure that benefits of promising new tools reach the populations that need it most, but can least afford them. Agencies such as such the Stop TB Partnership, Foundation for Innovative New Diagnostics, TB Diagnostics Initiative of the Special Programme for Research and Training in Tropical Diseases, WHO, are well placed to address these challenges Citation[51]. Funding and international support for the new Global Plan to Stop TB, 2006–2015 will also enhance the development and implementation of new tools for TB control Citation[116].
Conflict of interest statement
None declared.
Acknowledgements
This work was supported in part by the National Institutes of Health, Fogarty AIDS International Training Program (1-D43-TW00003-17), and National Institutes of Health/National Institute of Allergy and Infectious Diseases (R01 AI 34238). The authors thank several individuals who provided images and/or additional information: Katherine Sacksteder, Grace Lin, Rebecca Millecamps, Tanil Kocagoz, Thomas Silier, Richard Mole, Sanjay Tyagi, David AJ Moore and Luz Caviedes. The authors are grateful to Katherine Sacksteder, Sequella, Inc., and Andrew Ramsay, TDR/WHO, for critical feedback on a draft of this article. The authors thank the following companies for the information they provided: Innogenetics NV, Sequella, Inc., Eiken Chemical Co., Ltd and Salubris, Inc.
Key issues
• | Conventional diagnostics for tuberculosis (TB) disease and drug resistance have known limitations, and their performance in the context of the HIV epidemic has been suboptimal. | ||||
• | Development of new TB diagnostics is critically important to improve case detection and TB control. | ||||
• | Several new tests are being developed for the diagnosis of active TB and detection of drug resistance. These include simplified nucleic acid amplification tests, newer immune-based tests, rapid culture systems, phage-based assays, patch test, molecular beacons and microscopic observation drug-susceptibility assay. It is likely that some of these novel assays will become commercially available within the next 5–10 years. | ||||
• | With the resurgence of interest in the development of new tools for TB, it is likely that the next decade will see the introduction of novel diagnostics that can have an impact on the TB epidemic. | ||||
• | However, the challenge will be to ensure that new tools undergo rigorous evaluations in field conditions, and to ensure that benefits of new tools actually reach the populations that need it most, but can least afford them. | ||||
• | To accomplish this, greater emphasis must be placed on simple, inexpensive, low-tech tools that will be applicable in countries that carry a significant proportion of the global TB and HIV burden. |
References
- Corbett EL, Watt CJ, Walker N et al. The growing burden of tuberculosis: global trends and interactions with the HIV epidemic. Arch. Intern. Med.163(9), 1009–1021 (2003).
- World Health Organization. Global tuberculosis control. Surveillance, Planning, Financing. WHO Report 2005. World Health Organization, Geneva, Switzerland, 1–247 (2005).
- Foulds J, O’Brien R. New tools for the diagnosis of tuberculosis: the perspective of developing countries. Int. J. Tuberc. Lung Dis.2(10), 778–783 (1998).
- Perkins MD. New diagnostic tools for tuberculosis. Int. J. Tuberc. Lung Dis.4(12 Suppl. 2), S182–S188 (2000).
- Perkins MD, Small PM. Admitting defeat. Int. J. Tuberc. Lung Dis.10(1), 1 (2006).
- Fisher M. Diagnosis of MDR-TB: a developing world problem on a developed world budget. Expert Rev. Mol. Diagn.2(2), 151–159 (2002).
- Brodie D, Schluger NW. The diagnosis of tuberculosis. Clin. Chest Med.26(2), 247–271, vi (2005).
- Pai M. The accuracy and reliability of nucleic acid amplification tests in the diagnosis of tuberculosis. Natl Med. J. India17(5), 233–236 (2004).
- American Thoracic Society. Rapid diagnostic tests for tuberculosis: what is the appropriate use? American Thoracic Society Workshop. Am. J. Respir. Crit. Care Med.155(5), 1804–1814 (1997).
- Flores LL, Pai M, Colford JM Jr, Riley LW. In-house nucleic acid amplification tests for the detection of Mycobacterium tuberculosis in sputum specimens: meta-analysis and meta-regression. BMC Microbiol.5, 55 (2005).
- Pai M, Flores LL, Hubbard A, Riley LW, Colford JM Jr. Nucleic acid amplification tests in the diagnosis of tuberculous pleuritis: a systematic review and meta-analysis. BMC Infect. Dis.4(1), 6 (2004).
- Pai M, Flores LL, Pai N et al. Diagnostic accuracy of nucleic acid amplification tests for tuberculous meningitis: a systematic review and meta-analysis. Lancet Infect. Dis.3(10), 633–643 (2003).
- Piersimoni C, Scarparo C. Relevance of commercial amplification methods for direct detection of Mycobacterium tuberculosis complex in clinical samples. J. Clin. Microbiol.41(12), 5355–5365 (2003).
- Sarmiento OL, Weigle KA, Alexander J, Weber DJ, Miller WC. Assessment by meta-analysis of PCR for diagnosis of smear-negative pulmonary tuberculosis. J. Clin. Microbiol.41(7), 3233–3240 (2003).
- Iwamoto T, Sonobe T, Hayashi K. Loop-mediated isothermal amplification for direct detection of Mycobacterium tuberculosis complex, M. avium, and M. intracellulare in sputum samples. J. Clin. Microbiol.41(6), 2616–2622 (2003).
- Gennaro ML. Immunologic diagnosis of tuberculosis. Clin. Infect. Dis.30(Suppl. 3), S243–S246 (2000).
- Daniel TM. Antibody and antigen detection for the immunodiagnosis of tuberculosis: why not? What more is needed? Where do we stand today? J. Infect. Dis.158(4), 678–680 (1988).
- Bothamley GH. Serological diagnosis of tuberculosis. Eur. Respir. J. Suppl.20, 676s–688s (1995).
- Chan ED, Heifets L, Iseman MD. Immunologic diagnosis of tuberculosis: a review. Tuber. Lung Dis.80(3), 131–140 (2000).
- Laal S, Skeiky YAW. Immune-based methods. In: Tuberculosis and the Tubercle Bacillus. Cole ST, Eisenach KD, McMurray DN, Jacobs WR Jr (Eds), ASM Press, DC, USA, 71–83 (2005).
- Davidow A, Kanaujia GV, Shi L et al. Antibody profiles characteristic of Mycobacterium tuberculosis infection state. Infect. Immun.73(10), 6846–6851 (2005).
- Gennaro ML. Serologic tests for TB: is there hope? Int. J. Tuberc. Lung Dis.9(11), S18 (2005).
- Pereira Arias-Bouda LM, Nguyen LN, Ho LM et al. Development of antigen detection assay for diagnosis of tuberculosis using sputum samples. J. Clin. Microbiol.38(6), 2278–2283 (2000).
- Boehme C, Molokova E, Minja F et al. Detection of mycobacterial lipoarabinomannan with an antigen-capture ELISA in unprocessed urine of Tanzanian patients with suspected tuberculosis. Trans. R Soc. Trop. Med. Hyg.99(12), 893–900 (2005).
- Harboe M, Nagai S, Patarroyo ME et al. Properties of proteins MPB64, MPB70, and MPB80 of Mycobacterium bovis BCG. Infect. Immun.52(1), 293–302 (1986).
- Nakamura RM, Velmonte MA, Kawajiri K et al. MPB64 mycobacterial antigen: a new skin-test reagent through patch method for rapid diagnosis of active tuberculosis. Int. J. Tuberc. Lung Dis.2(7), 541–546 (1998).
- Nakamura RM, Einck L, Velmonte MA et al. Detection of active tuberculosis by an MPB-64 transdermal patch: a field study. Scand. J. Infect. Dis.33(6), 405–407 (2001).
- Geisbrecht BV, Nikonenko B, Samala R et al. Design and optimization of a recombinant system for large-scale production of the MPT64 antigen from Mycobacterium tuberculosis. Protein Expr. Purif.46, 64–72 (2006).
- Baylan O, Kisa O, Albay A, Doganci L. Evaluation of a new automated, rapid, colorimetric culture system using solid medium for laboratory diagnosis of tuberculosis and determination of anti-tuberculosis drug susceptibility. Int. J. Tuberc. Lung Dis.8(6), 772–777 (2004).
- Kocagoz T, O’Brien R, Perkins M. A new colorimetric culture system for the diagnosis of tuberculosis. Int. J. Tuberc. Lung Dis.8(12), 1512; author reply 1513 (2004).
- Morgan M, Kalantri S, Flores L, Pai M. A commercial line probe assay for the rapid detection of rifampicin resistance in Mycobacterium tuberculosis: a systematic review and meta-analysis. BMC Infect. Dis.5, 62 (2005).
- Hillemann D, Weizenegger M, Kubica T, Richter E, Niemann S. Use of the genotype MTBDR assay for rapid detection of rifampin and isoniazid resistance in Mycobacterium tuberculosis complex isolates. J. Clin. Microbiol.43(8), 3699–3703 (2005).
- Makinen J, Marttila HJ, Marjamaki M, Viljanen MK, Soini H. Comparison of two commercially available DNA line probe assays for detection of multidrug-resistant Mycobacterium tuberculosis. J. Clin. Microbiol.44(2), 350–352 (2006).
- Kalantri S, Pai M, Pascopella L, Riley L, Reingold A. Bacteriophage-based tests for the detection of Mycobacterium tuberculosis in clinical specimens: a systematic review and meta-analysis. BMC Infect. Dis.5(1), 59 (2005).
- Pai M, Kalantri S, Pascopella L, Riley LW, Reingold AL. Bacteriophage-based assays for the rapid detection of rifampicin resistance in Mycobacterium tuberculosis: a meta-analysis. J. Infect.51(3), 175–187 (2005).
- Wilson SM, al-Suwaidi Z, McNerney R, Porter J, Drobniewski F. Evaluation of a new rapid bacteriophage-based method for the drug susceptibility testing of Mycobacterium tuberculosis. Nature Med.3(4), 465–468 (1997).
- Jacobs WR Jr, Barletta RG, Udani R et al. Rapid assessment of drug susceptibilities of Mycobacterium tuberculosis by means of luciferase reporter phages. Science260(5109), 819–822 (1993).
- Mole RJ, Trollip AP, Seaman T, Abrahams C, Albert H. Improved contamination control using a new antimicrobial supplement developed for rapid phage-based rifampicin susceptibility testing. Int. J. Tuberc. Lung Dis.9(11 Suppl. 1), S271 (2005).
- Guerra H, Rojas G, Chauca J et al. Evaluation of a phage amplification assay for detection of MDR-TB in smear-positive patients in Lima, Peru (preliminary report). Int. J. Tuberc. Lung Dis.9(11 Suppl. 1), S196 (2005).
- Riska PF, Su Y, Bardarov S et al. Rapid film-based determination of antibiotic susceptibilities of Mycobacterium tuberculosis strains by using a luciferase reporter phage and the Bronx box. J. Clin. Microbiol.37(4), 1144–1149 (1999).
- Hazbon MH, Guarin N, Ferro BE et al. Photographic and luminometric detection of luciferase reporter phages for drug susceptibility testing of clinical Mycobacterium tuberculosis isolates. J. Clin. Microbiol.41(10), 4865–4869 (2003).
- El-Hajj HH, Marras SA, Tyagi S, Kramer FR, Alland D. Detection of rifampin resistance in Mycobacterium tuberculosis in a single tube with molecular beacons. J. Clin. Microbiol.39(11), 4131–4137 (2001).
- Lin SYG, Probert W, Lo M, Desmond E. Rapid detection of isoniazid and rifampin resistance mutations in Mycobacterium tuberculosis complex from cultures or smear-positive sputa by use of molecular beacons. J. Clin. Microbiol.42(9), 4204–4208 (2004).
- Piatek AS, Telenti A, Murray MR et al. Genotypic analysis of Mycobacterium tuberculosis in two distinct populations using molecular beacons: implications for rapid susceptibility testing. Antimicrob. Agents Chemother.44(1), 103–110 (2000).
- Varma-Basil M, El-Hajj H, Colangeli R et al. Rapid detection of rifampin resistance in Mycobacterium tuberculosis isolates from India and Mexico by a molecular beacon assay. J. Clin. Microbiol.42(12), 5512–5516 (2004).
- Lin SYG, Mase S, Desmond E et al. Utilization of molecular beacons for rapid detection of MDR-TB in a public health setting. 45th Annual Interscience Conference on Antimicrobial Agents and Chemotherapy, DC, USA, (2005).
- Caviedes L, Lee TS, Gilman RH et al. Rapid, efficient detection and drug susceptibility testing of Mycobacterium tuberculosis in sputum by microscopic observation of broth cultures. The Tuberculosis Working Group in Peru. J. Clin. Microbiol.38(3), 1203–1208 (2000).
- Moore DA, Mendoza D, Gilman RH et al. Microscopic observation drug susceptibility assay, a rapid, reliable diagnostic test for multidrug-resistant tuberculosis suitable for use in resource-poor settings. J. Clin. Microbiol.42(10), 4432–4437 (2004).
- Small PM, Perkins MD. More rigour needed in trials of new diagnostic agents for tuberculosis. Lancet356(9235), 1048–1049 (2000).
- Nahid P, Pai M, Hopewell PC. Advances in the diagnosis and treatment of tuberculosis. Proc. Am. Thorac. Soc.3, 103–110 (2006).
- Perkins MD, Roscigno G, Zumla A. Progress towards improved tuberculosis diagnostics for developing countries. Lancet367, 942–943 (2006).
Websites
- Stop TB Working Group on New Diagnostics www.stoptb.org/wg/new_diagnostics
- Foundation for Innovative New Diagnostics www.finddiagnostics.org
- Tuberculosis Diagnostics Initiative, Special Programme for Research and Training in Tropical Diseases, WHO www.who.int/tdr/diseases/tb/tbdi.htm
- Aeras Global TB Vaccine Foundation www.aeras.org
- Global Alliance for Tuberculosis Drug Development www.tballiance.org
- Gen-Probe Inc. www.gen-probe.com
- Roche Diagnostics www.roche-diagnostics.com
- Becton Dickinson www.bd.com
- Eiken Genome Site http://loopamp.eiken.co.jp/e/index.html
- Sequella, Inc. http://sequella.com
- Salubris, Inc. www.salubrisinc.com
- Innogenetics NV www.innogenetics.com
- Hain Lifescience GmbH www.hain-lifescience.de
- Biotec Laboratories Ltd www.biotec.com
- Molecular Beacons, Public Health Research Institute www.molecular-beacons.org
- Global Plan to Stop TB, 2006–2015 www.stoptb.org/globalplan