Abstract
Multiple sclerosis (MS) is a multifaceted disease, in which an inflammatory autoimmune attack on the myelin in the central nervous system (CNS) leads to extensive demyelination and subsequent axonal pathology. The challenge for MS therapy is to combine effective immunomodulatory therapies with novel neuroprotective approaches that promote repair, in particular remyelination, beyond its limited spontaneous extent. Cumulative findings indicate that immunomodulatory treatments can induce neuroprotective outcomes and provide a supportive milieu for repair processes. Growing understanding of MS pathology together with biotechnological advances has resulted in promising strategies such as inhibitory molecules, monoclonal antibodies and cell therapies. Several candidates that have shown significant effects on the oligodendrocyte population and/or myelin synthesis in animal models are currently or soon to be tested in clinical trials.
The myelin sheath is essential for optimizing the nerve impulse conduction, as well as for axonal survival and regenerative capacity. In multiple sclerosis (MS), inflammatory autoimmune attack on the myelin in the central nervous system (CNS) leads to myelin destruction and formation of demyelinated lesions (plaques) in the white matter.[Citation1] Diffuse changes in normal-appearing white matter and cortical demyelination have also been recognized as components of MS pathology. The widespread demyelination results in subsequent neuroaxonal damages, which comprise the degenerative aspect of the disease. The fundamental role of inflammation in MS pathology provided the rationale for immunomodulatory disease-modifying therapies that shift the immune system from pro-inflammatory to anti-inflammatory pathways, thus effectively reducing relapse rate and new lesions appearance in relapsing-remitting (RR) MS.[Citation2] However, as yet, no treatment strategy has proven to significantly repair the CNS tissue damage and affect the progressive disease phase. The essential challenge for MS therapy is therefore to promote neuroprotective repair mechanisms, in particular remyelination.
A prerequisite for developing new treatments is to understand the cellular and molecular mechanisms underlying remyelination and the reasons for insufficient endogenous repair in MS. Animal models have been highly valuable for investigating the principal features of de- and re-myelination in the CNS. These include various forms of experimental autoimmune encephalomyelitis (EAE) in which demyelination occurs on an inflammatory background, as well as those induced by toxic agents like cuprizone which exhibit demyelination in relative absence of inflammation.[Citation3] An additional instrumental system is postnatal myelinogenesis, as some features of developmental myelination reappear during remyelination.[Citation4] In spite of the imperative contribution of these models, it is clear that remyelination in MS cannot be mirrored by a single system. Combination of several models as well as studies in MS patients are needed for more accurate prospective of this disease.
Remyelination is a complex process that involves multiple steps, precise timing and coordination among different cell types. In the CNS, the oligodendrocyte macroglia synthesize myelin, extending processes to neighboring axons and enwrapping short axonal segments with several myelin layers.[Citation5] Oligodendrocytes also provide trophic support and maintain the myelin sheaths. Importantly, myelin-producing oligodendrocytes are terminally differentiated cells with a limited capacity to respond to injury. In active demyelinating lesions they are destroyed, though to an extent that varies among patients.[Citation1] Accordingly, remyelination requires proliferation of oligodendrocyte precursor cells (OPCs), their migration/recruitment into demyelinating sites and differentiation to mature myelinating oligodendrocytes. CNS injury in itself is a potent inducer of repair,[Citation6] so subsequent to the demyelination, remyelination is triggered. Indeed, at the early stages of MS in newly evolved lesions, OPCs recruitment to demyelination sites frequently results in remyelination. However, at later disease stages, in particular in chronic lesions, remyelination is either absent or confined to a small rim at the lesion border.[Citation1] Oligodendrocyte recruitment failure and maturation defects involving several signaling pathways are implicated in the poor remyelination occurring in chronic MS.[Citation1,Citation7] It is clear that factors that direct myelination during normal development are inhibited or absent in the MS lesions.
In view of the limited extent of endogenous remyelination, there is a great need for therapeutic approaches that will improve myelin repair. To a certain extent any intervention that arrests disease progression is expected to reduce demyelination, at least through secondary indirect mechanisms. However, anti-inflammatory treatments might also block the beneficial aspects of inflammation such as neurotrophins secretion. Questions have therefore been raised whether immune-based treatments that demonstrate beneficial consequences on inflammation can also induce neuroprotection and repair. Findings from animal models revealed beneficial effects of several immunomodulatory treatments on the oligodendrocyte population as well as on remyelination.[Citation6,Citation8] For example, treatment of EAE-induced mice with glatiramer acetate (GA), applied even after extensive demyelination occurred, results in reduced myelin damage, suggesting repair.[Citation9,Citation10] Ultrastructural quantitative analysis of the remyelinated axons compared to the “naked” demyelinated axons by transmission electron microscopy (TEM) provided evidence for significant elevation of the remyelination in EAE mice following GA treatment.[Citation11] This was further confirmed by employing MRI parameters such as magnetization transfer ratio (MTR), which indicate macromolecules/myelin loss.[Citation12] In the developing nervous system, GA enhanced postnatal myelinogenesis elevating the number of myelinated axons as well as the thickness of the myelin encircling them and their resulting G-ration.[Citation13] These effects are attributed to increased OPCs proliferation and differentiation along the oligodendroglial maturation cascade.[Citation9,Citation13] GA induces elevation in brain-derived neurotrophic factor (BDNF) and insulin-like growth factor-1 (IGF-1), which are known to promote myelination, suggesting that its mode of action is linked to neurotrophic effects.[Citation13,Citation14] Another immunomodulatory treatment, fingolimod, was shown to modulate process outgrowth in immature oligodendrocytes and to enhance remyelination following lysolecithin-induced demyelination via sphingosine 1-phosphate receptors on astrocytes and oligodendrocytes.[Citation15] However, conflicting results regarding the remyelination potency of FTY720 have been recently reported.[Citation16] An additional immunomodulator, laquinimod, applied at the chronic EAE stage reduced myelin damages and induced neurotropic effect.[Citation17] These combined findings support the notion that immunomodulation can support neuroprotection and remyelination within the CNS. Whether these effects result from genuine repair mechanisms or from anti-inflammatory consequences remain to be established. Regardless, the feasibility of repairing the extensive myelin damages in MS by immunomodulatory treatments is doubted, particularly in the progressive phase. Intensive efforts are thus devoted to develop “genuine” remyelination strategies that will be used as add-ons to the immunomodulatory therapeutics.
Stimulation of remyelination can be achieved by inhibiting factors that hinder myelin repair and/or by up-regulating growth factors that support oligodendrocytes development and function. Putative targets for inhibition are myelin-associated components that negatively regulate oligodendroglial differentiation, such as the myelin-associated neurite outgrowth inhibitor Nogo-A. A monoclonal antibody to Nogo receptor interacting protein-1 (LINGO-1) is currently being tested in clinical trials.[Citation18] Some growth factors, such as erythropoietin and thyroid hormones, induce beneficial effects in various experimental models.[Citation3,Citation6,Citation8] IGF-1 first showed promising results by enhancing oligodendrocyte growth and maturation, but conflicting findings in EAE were reported and no clinical benefit was found in a small clinical study.[Citation19] Fibroblast growth factor-2 (FGF2) was found to promote the development of the oligodendrocyte lineage in vitro. However, further studies indicated that FGF2 does not induce a beneficial effect in vivo, and may even prevent myelination.[Citation20] These results emphasize the potential risk involved in growth factors therapy. In addition, this approach has potential obstacles due to the short half-life of these molecules, delivery problems to the CNS and side effects.
Several novel therapeutic candidates that have shown promising effects on the oligodendrocyte population and/or myelin synthesis in animal models are currently or soon to be tested in clinical trials.[Citation3,Citation21,Citation22] These include: rHIgM22 a recombinant autoantibody; the GNbAC1 antibody directed against the envelope protein of MS-associated retrovirus; quetiapine fumarate (chemically similar to the MS approved drug dimethyl fumarate); histamine receptor antagonists; VX15/2503, an antibody interfering with semaphorin 4D interaction with its receptor; an agonist for the retinoic acid receptor gamma (RXR-γ) and olesoxime, a cholesterol-like small-molecule compound. A number of small molecules targeting signaling cascades (such as the Notch pathway) that inhibit OPCs differentiation and remyelination in MS lesions are also under study.
Cell transplantation is also a promising strategy. Introducing exogenous pluripotent cells with differentiation and proliferation potential may replace the affected oligodendrocytes and induce myelin repair. Various cell types (e.g., neuronal progenitor cells, OPCs, oligodendrocytes, Schwann cells, mesenchimal stem cells, etc.) are potential candidates for this purpose. Indeed, in EAE mice, neuronal progenitor cells, administered by either intracerebral transplantation or systemic injection, were detected in CNS inflammatory sites, and their occurrence has been associated with remyelination and reduced disease severity.[Citation22,Citation23] Clinical trials testing the effect of these cells in MS patient are ongoing. Notably, accumulated evidence implies that the beneficial effect of the transplanted cells may be attributed to an immunomodulatory effect and trophic support, rather than to oligodendrocytes replacement. Cell administration (systemic or intracerebral) to the damage sites is a challenge due to the multifocal nature of MS. The uncertainty regarding the potential of transplanted stem cells to give rise to cancer cells is still being debated.
Important CNS elements that may be directed to affect myelin regeneration are the astrocytes forming the glial scar that impedes OPCs recruitment, as well as the microglia responsible for clearing the myelin debris from the extracellular matrix.[Citation1,Citation4] Further elucidation of the roles of these cell populations in MS may provide useful insights for remyelination failure, leading to the identification of novel therapeutic targets.
Myelin repair that will restore fiber conduction and trophic support is a major goal for MS therapy, involving a very active field of research. It should be stressed that remyelination is not the only challenge. Neuroaxonal pathology is also a significant constitute of this disease, occurring either secondary to the demyelination or independently as primary degeneration.[Citation1,Citation3] This could limit the effect of remyelination-stimulating treatments, at least in the subset of patients suffering from extensive axonal injury, particularly in the progressive stage. Currently, there is no treatment for which myelin repair, as such, has been proved to be effective and beneficial for MS patients. However, progress made in understanding the different pathologies associated with MS together with biotechnological advances will hopefully produce promising regeneration strategies. Tools to detect remyelinated lesions in the MS brain had been rather limited, but a growing number of clinical studies have incorporated novel imaging techniques to assess neuroprotective treatment outcomes. The ultimate goal is to provide a combination of effective immunomodulation together with regenerative modalities that will offer disease control and substantial reduction in the risk for disease progression.
Financial & competing interest disclosure
The author has received a research grant from Teva Pharmaceutical Industries (Israel). The author has no other affiliations or financial involvement with any organization or entity with financial interests in or financial conflicts with the subject matter or materials discussed in the article.
Additional information
Notes on contributors
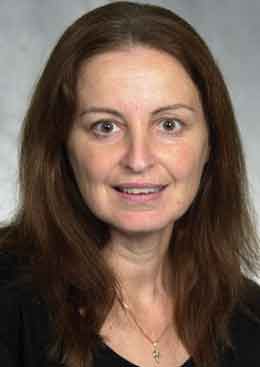
Rina Aharoni
References
- Kutzelnigg A, Lassmann H. Pathology of multiple sclerosis and related inflammatory demyelinating diseases. In: Goodin DS, editor. Handbook of clinical neurology, multiple sclerosis and related disorders. Vol. 122. New York (NY): Elsevier; 2014. p. 15–58.
- Michel L, Larochelle C, Prat A. Update on treatments in multiple sclerosis. Press Med. 2015;44:137–151.
- Aharoni R. New findings and old controversies in the research of multiple sclerosis and its model experimental autoimmune encephalomyelitis. Expert Rev Clin Immunol. 2013;9:423–440.
- Bhatt A, Fan LW, Pang Y. Strategies for myelin regeneration: lessons learned from development. Neural Regen Res. 2014;9:1347–1350.
- Simons M, Nave KA. Oligodendrocytes: myelination and axonal support. In: Barres BA, Freeman MR, Stevens B, editors. Cold spring harbor perspective in biology. Cold Spring Harbor (NY): Cold Spring Harbor Laboratory Press; 2015. p. 1–15.
- Aharoni R, Arnon R. Linkage between immunomodulation neuroprotection and neurogenesis. Drug News Perspect. 2009;22:301–312.
- Hanafy KA, Sloane JA. Regulation of remyelination in multiple sclerosis. FEBS Lett. 2011;585:3821–3828.
- Luessi F, Siffrin V, Zipp F. Neurodegeneration in multiple sclerosis: novel treatment strategies. Expert Rev Neurother. 2012;12:1061–1076.
- Aharoni R, Herschkovitz A, Eilam R, et al. Demyelination arrest and remyelination induced by glatiramer acetate treatment of experimental autoimmune encephalomyelitis. Proc Natl Acad Sci USA. 2008;105:11358–11363.
- Arnon R, Aharoni R. Neuroprotection and neurogeneration in MS and its animal model EAE affected by glatiramer acetate. J Neural Transm. 2009;116:1443–1449.
- Aharoni R, Vainshtein A, Stock A, et al. Distinct pathological patterns in relapsing-remitting and chronic models of experimental autoimmune enchephalomyelitis and the neuroprotective effect of glatiramer acetate. J Autoimmun. 2011;37:228–241.
- Aharoni R, Sasson E, Blumenfeld T. Magnetic resonance imaging characterization of different experimental autoimmune encephalomyelitis models and the therapeutic effect of glatiramer acetate. Exp Nerurol. 2013;240:130–144.
- From R, Eilam R, Bar-Lev DD, et al. Oligodendrogenesis and myelinogenesis during postnatal development effect of glatiramer acetate. GLIA. 2014;62:549–665.
- Aharoni R, Eilam R, Domev H, et al. The immunomodulator glatiramer acetate augments the expression of neurotrophic factors in brains of experimental autoimmune encephalo-myelitis in mice. Proc Natl Acad Sci USA. 2005;102:19045–19050.
- Miron VE, Ludwin SK, Darlington PJ, et al. Fingolimod (FTY720) enhances remyelination following demyelination of organotypic cerebellar slices. Am J Pathol. 2010;176:2682–2694.
- Alme MN, Nystad AE, Bø L, et al. Fingolimod does not enhance cerebellar remyelination in the cuprizone model. J Neuroimmunol. 2015;15:180–186.
- Aharoni R, Saada R, Eilam R, et al. Oral treatment with laquinimod augments regulatory T-cells and brain-derived neurotrophic factor expression and reduces injury in the CNS of mice with experimental autoimmune encephalomyelitis. J Neuroimmunol. 2012;251:14–24.
- Mi S, Pepinsky RB, Cadavid D. Blocking LINGO-1 as a therapy to promote CNS repair: from concept to the clinic. CNS Drugs. 2013;27:493–503.
- Chesik D, Wilczak N, De Keyser J. The insulin-like growth factor system in multiple sclerosis. Int Rev Neurobiol. 2007;79:203–226.
- Woodbury ME, Ikezu TJ. Fibroblast growth factor-2 signaling in neurogenesis and neurodegeneration. Neuroimmunol Pharmacol. 2014;9:92–101.
- Kremer D, Küry P, Dutta R. Promoting remyelination in multiple sclerosis: current drugs and future prospects. Mult Scler J. 2015;21:541–549.
- Olsen JA, Akirav EM. Remyelination in multiple sclerosis: cellular mechanisms and novel therapeutic approaches. J Neurosci Res. 2015;93:687–696.
- Orack JC, Deleidi M, Pitt D, et al. Concise review: modeling multiple sclerosis with stem cell biological platforms: toward functional validation of cellular and molecular phenotypes in inflammation-induced neurodegeneration. Stem Cells Transl Med. 2015;4:252–260.