Abstract
Three-dimensional (3D) printing in congenital heart disease has the potential to increase procedural efficiency and patient safety by improving interventional and surgical planning and reducing radiation exposure. Cardiac magnetic resonance imaging and computed tomography are usually the source datasets to derive 3D printing. More recently, 3D echocardiography has been demonstrated to derive 3D-printed models. The integration of multiple imaging modalities for hybrid 3D printing has also been shown to create accurate printed heart models, which may prove to be beneficial for interventional cardiologists, cardiothoracic surgeons, and as an educational tool. Further advancements in the integration of different imaging modalities into a single platform for hybrid 3D printing and virtual 3D models will drive the future of personalized cardiac medicine.
High-resolution cardiac imaging and 3D printing has ushered in an era where rapid prototyping of congenital heart defects (CHDs) is possible.[Citation1] The insight provided by 3D-printed models has the potential to increase procedural efficiency by improving interventional or surgical planning and reducing radiation exposure. The source images for 3D-printed models are usually derived from high-resolution, cross-sectional cardiac MRI (c-MRI) or computed tomography (CT).[Citation2,Citation3] Intravenous contrast is often required for CT and exposes patients to ionizing radiation. For c-MRI, intubation and sedation is necessary in many pediatric patients. 3D echocardiography, a noninvasive bedside imaging tool, has been recently demonstrated to generate 3D-printed models.[Citation4] Accurate morphology of valvular structures derived from 3D transesophageal echocardiography (TEE) integrated with “blood pool” imaging from CT has also been used in 3D printing.[Citation5] Further advancements in the integration of different imaging modalities into a single platform for virtual dynamic 3D modeling and hybrid 3D printing will drive the future of cardiac imaging.
Initially, 3D printing in medicine was primarily used in orthopedic and plastic surgery. It involved the use of 3D radiological images to generate a replica of anatomical structures. The complexity of CHDs requires high-quality image acquisition of cardiovascular anatomy and morphology. Although on-screen 3D renderings of cardiac structures are now routinely available on standard CT or MR workstations, these renderings cannot be used interactively to simulate transcatheter or surgical implantation of medical devices. The output format derived from these renderings generally does not permit conversion into tangible models. Segmentation of individual imaging data sets creates multiplanar 3D virtual models. This data set is then exported into a dedicated postprocessing software such as Mimics® (Materialise N. V., Leuven, Belgium) for computer-assisted design and rapid prototyping. The software enables semi-automated integration of multiple imaging modalities in a single platform and realignment of the integrated anatomy to generate printable 3D data sets.
Furthermore, rapid prototyping from the data sets for printing patient-specific cardiac models can be sliced in the required anatomic planes to display the region of specific interest.[Citation4–Citation6] The present state-of-the-art technology involves the use of multiple imaging modalities acquired within a short period of time, followed by segmentation and prototyping of the 3D model within 24 h. Note that as each imaging modality has different strengths – CT enhances visualization of extracardiac anatomy [Citation7]; c-MRI is superior to other imaging modalities for quantification of ventricular volumes and myocardial architecture [Citation8]; 3D ultrasound provides the best visualization of valve morphology [Citation9] – it can impact the quality and accuracy of the 3D model.
Utilization of information derived from integrating multiple imaging modalities can provide precise models of complex CHDs. It is of utmost importance that printed models closely replicate the cardiac structure itself for the benefit of the interventionalist or surgeon. This is also a significant factor for patient safety if clinical decisions are to be made from the 3D-printed model. Therefore, all efforts must focus on integrating the best aspects of each imaging modality to create highly accurate hybrid 3D models. It is recommended that the cardiologist and surgeon involved in the patient’s care be actively involved in the process of identifying the targeted cardiac phases for reconstruction, segmentation and rapid prototyping.[Citation10,Citation11] The accuracy of depiction of cardiac structures and the plane of interest for a particular condition can be determined only by providers directly involved in the patient’s care. The 3D models can be printed in rigid or flexible materials or a combination of both in one to define tissue differentiation or specific areas of calcification. We recently demonstrated the feasibility of such an approach in a patient with congenitally corrected transposition of the great arteries by integrating CT and 3D TEE for hybrid 3D printing using HeartPrint® Flex technology (Materialise N. V.).[Citation5] Currently, there is no material that can completely and accurately mimic human tissue, but the flexible model comes close to representing the properties of soft tissue.[Citation4,Citation5,Citation11] We made further progress in hybrid 3D printing (Burton Precision and Engineering 4 Life, Comstock Park, MI, USA) derived from MRI and 3D TEE in a patient with pulmonary atresia and single-ventricle palliation who subsequently underwent successful 1½ ventricle repair. Although the operative anatomy confirmed the anatomic details of double-chambered right ventricle as displayed by the 3D-printed model, it did not significantly alter the management decisions of the multidisciplinary team. As such, 3D-printed models may be used as an additional tool along with other imaging modalities for interventional and surgical planning.
The ability to perform preoperative evaluation of the size and shape of shunts or patches is one potential application of hybrid 3D-printed models. The relationship and the actual distance between vital structures can be also clearly visualized preprocedure, precluding the need for extensive dissection to confirm such structures. As a result, the 3D-printed model may indirectly reduce the duration of surgery and cardiopulmonary bypass time and prevent inadvertent complications. Clinical preprocedure decision-making has been demonstrated in patients with cardiac tumors, shunt lesions, transplantation in univentricular hearts, transcatheter aortic and pulmonary valve implantation and stenting of aortic coarctation.[Citation4,Citation6,Citation12–Citation17]
Multimodality imaging techniques have facilitated the understanding of complex morphology in CHDs. However, currently used terminologies can be quite misleading. Individuals with the same anatomic diagnosis can have varied morphology and prognosis depending on associated comorbidities. Many complex CHDs fall into this category. A typical example is that of atrioventricular septal defect (AVSD) with a common atrioventricular valve, which refers to various conditions from simple ostium primum defects to complex unbalanced AVSDs with heterotaxy syndrome. The ventricular size in unbalanced AVSDs may be severely underestimated based on common imaging modalities in current practice.[Citation18] This may have far-reaching implications for biventricular repair in “unbalanced” AVSDs. Associated defects like total anomalous pulmonary venous connection in AVSD significantly alter long-term prognosis.[Citation19] Thus, each individual with CHD is different and there is need for personalized care, rather than protocol-based management. The number of patients with distinct conditions is very small, making it difficult to make decisions solely based on experience. In this context, personalized medicine becomes very relevant and hybrid 3D printing may enhance management in these patients.[Citation20,Citation21]
The morphology and pathophysiology of complex CHDs is often difficult to comprehend for inexperienced medical professionals or students. The mental depiction of a defect created by integrating the basic knowledge of anatomy, physiological principles and flow dynamics may not reflect the actual CHD. This is further complicated by altered electrical pathways, artificial connections between vessels, scarred tissue and artificial valves. As complex CHDs are rare, new surgeons may have inadequate exposure to complex anatomy. The visual and tactile input provided by 3D-printed models markedly improves understanding of complex interrelationship between structures in the heart.[Citation23] The creation of a library of 3D CHD models can make pediatric cardiology more interesting and easier for medical professionals and students to visualize and understand complex intracardiac anatomy in training and practice.[Citation20] The field of congenital cardiac interventions is ideal for simulation-based learning because of the rarity, complexity and individual variability. High-fidelity medical simulations using 3D printing technology have been shown to effectively complement medical education in patient care settings.[Citation22–Citation24] Cardiothoracic surgeons can plan their interventions and have exact measurements of shunts or conduits as 3D-printed models are an exact depiction of intraoperative anatomy.
As we advance to a stage wherein hearts may be printed out within the duration of an outpatient visit, it may be worthwhile to consider the indications or degree of complexity of CHDs for 3D printing. This may reduce commercial misuse of this significant technological advancement. The time frame for printing a heart presently is a minimum of 15 h, which includes 2–3 h each for segmentation and rapid prototyping and 10 h for printing and finishing. The cost involved may vary from $500 for simple models [Citation4] to $3500 or more for complex models,[Citation5] depending on the size, material, and complexity of the CHD.
The main limitation of 3D-printed models is that it is a static representation of a dynamic organ. Virtual 3D anatomic models of CHDs at different phases of the cardiac cycle need to be created to get further insight into functional morphology and to evaluate dynamic changes during the cardiac cycle. The discrepancies in the data sets from gain setting, contour detection and interobserver variability can lead to drop out in the stereolithography format, creating inaccuracies in the printed model. This could be overcome by a knowledge-based virtual heart model that can handle variation in data input. The incorporation of the hemodynamic data into this dynamic virtual model and development of computer models to predict the results of interventional or surgical procedures with integrated volume changes, compliance and function will further advance management of patients. The integration of electrical functional pathways and properties will also enhance the utility of virtual 3D heart models in the management of complex CHDs. Visualizing a 3D structure on a 2D screen causes the observer to imagine it from different perspectives, leading to misinterpretation and interobserver variability. The advent of holographic projection decreases this risk, leaving no aspect of spatial relationships to the imagination, but further development is required for clinical applications.[Citation21]
Finally, tissue engineering of biocompatible materials, cells and supporting components has been successfully demonstrated.[Citation25] Deriving the appropriate scaffolds from multiple imaging modalities and incorporating stem cell cultures from the patient for 3D-bioprinting of different functional structures of the heart or great vessels may not be a distant dream in personalized cardiac medicine.[Citation11]
Financial and competing interests disclosure
J.J. Vettukattil has a non-disclosure agreement with Materialise N.V., Burton Precision and Engineering 4 Life. The authors have no other relevant affiliations or financial involvement with any organization or entity with a financial interest in or financial conflict with the subject matter or materials discussed in the manuscript apart from those disclosed.
ORCID
Bennett P Samuel http://orcid.org/0000-0002-0914-9536
Additional information
Notes on contributors
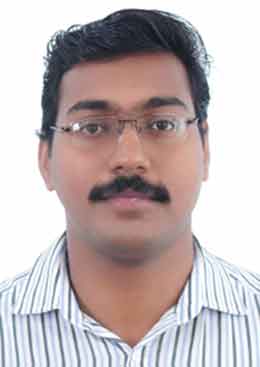
Harikrishnan KN Kurup
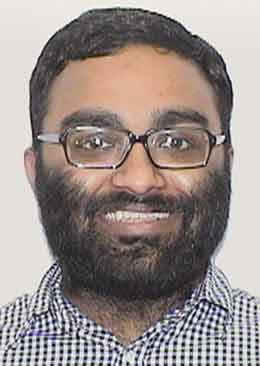
Bennett P Samuel
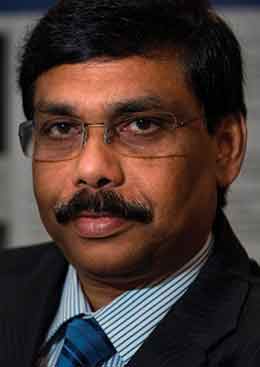
Joseph J Vettukattil
References
- Papers of special note have been highlighted as:
- • of interest
- •• of considerable interest
• Describes the feasibility of accurate reconstruction of congenital heart anomalies using data from CT and MRI.
- Mosadegh B, Xiong G, Dunham S, et al. Current progress in 3D printing for cardiovascular tissue engineering. Biomed Mater. 2015;10(3):034002. DOI:10.1088/1748-6041/10/3/034002.
- Markert M, Weber S, Lueth TC. A beating heart model 3D printed from specific patient data. Conf Proc IEEE Eng Med Biol Soc. 2007;2007:4472–4475.
- Samuel BP, Pinto C, Pietila T, et al. Ultrasound-derived three-dimensional printing in congenital heart disease. J Digit Imaging. 2015;28(4):459–461. DOI:10.1007/s10278-014-9761-5.
• Demonstrates the feasibility of printing heart models using 3D echocardiographic data set.
- Gosnell JM, Pietila T, Samuel BP, et al. Hybrid three-dimensional printing derived from multiple imaging modalities. Presented at: Catheter Interventions in Congenital, Structural, and Valvular Heart Disease; 2015 Jun 24–27; Frankfurt (Germany). Available from: https://www.researchgate.net/publication/279173553_E6._Hybrid_three-dimensional_printing_derived_from_multiple_imaging_modalities
•• Discusses proof of concept of integrating multiple imaging modalities to generate a hybrid model for 3D printing.
- Olivieri LJ, Krieger A, Loke YH, et al. Three-dimensional printing of intracardiac defects from three-dimensional echocardiographic images: feasibility and relative accuracy. J Am Soc Echocardiogr. 2015;28(4):392–397. DOI:10.1016/j.echo.2014.12.016.
- Goitein O, Salem Y, Jacobson J, et al. The role of cardiac computed tomography in infants with congenital heart disease. Isr Med Assoc J. 2014;16(3):147–152.
- Luijnenburg SE, Robbers-Visser D, Moelker A, et al. Intra-observer and interobserver variability of biventricular function, volumes and mass in patients with congenital heart disease measured by CMR imaging. Int J Cardiovasc Imaging. 2010;26(1):57–64. DOI:10.1007/s10554-009-9501-y.
- Black D, Vettukattil J. Advanced echocardiographic imaging of the congenitally malformed heart. Curr Cardiol Rev. 2013;9(3):241–252. DOI:10.2174/1573403x11309030008.
- O’Neill B, Wang DD, Pantelic M, et al. Transcatheter caval valve implantation using multimodality imaging: roles of TEE, CT, and 3D printing. JACC Cardiovasc Imaging. 2015;8(2):221–225. DOI:10.1016/j.jcmg.2014.12.006.
- O’Neill B, Wang DD, Pantelic M, et al. Reply: the role of 3D printing in structural heart disease: all that glitters is not gold. JACC Cardiovasc Imaging. 2015;8(8):968–969. DOI:10.1016/j.jcmg.2015.04.011.
• Provides insight into the deficiencies of currently available techniques in 3D printing.
- Olivieri L, Krieger A, Chen MY, et al. 3D heart model guides complex stent angioplasty of pulmonary venous baffle obstruction in a Mustard repair of D-TGA. Int J Cardiol. 2014;172(2):e297–298. DOI:10.1016/j/ijcard.2013.12.192.
- Schmauss D, Gerber N, Sodian R. Three-dimensional printing of models for surgical planning in patients with primary cardiac tumors. J Thorac Cardiovasc Surg. 2013;145(5):1407–1408. DOI:10.1016/j.jtcvs.2012.12.030.
- Schmauss D, Schmitz C, Bigdeli AK, et al. Three-dimensional printing of models for preoperative planning and simulation of transcatheter valve replacement. Ann Thorac Surg. 2012;93(2):e31–3. DOI:10.1016/j.athoracsur.2011.09.031.
- Sodian R, Weber S, Markert M, et al. Pediatric cardiac transplantation: three-dimensional printing of anatomic models for surgical planning of heart transplantation in patients with univentricular heart. J Thorac Cardiovasc Surg. 2008;136(4):1098–1099. DOI:10.1016/j.jtcvs.2008.03.055.
- Biglino G, Verschueren P, Zegels R, et al. Rapid prototyping compliant arterial phantoms for in-vitro studies and device testing. J Cardiovasc Magn Reson. 2013;15(1):2. DOI:10.1186/1532-429X-15-2.
- Valverde I, Gomez G, Coserria JF, et al. 3D printed models for planning endovascular stenting in transverse aortic arch hypoplasia. Catheter Cardiovasc Interv. 2015;85(6):1006–1012. DOI:10.1002/ccd.25810.
- Ahmad Z, Lim Z, Roman K, et al. The angulation of the septal structures impacts ventricular imbalance in atrioventricular septal defects with a common atrioventricular junction. Cardiol Young. 2015. Epub ahead of print. DOI:10.1017/S1047951115000219.
• Highlights the morphological variability in AVSDs and the need for personalized medicine in CHD.
- Sinzobahamvya N, Arenz C, Reckers J, et al. Poor outcome for patients with totally anomalous pulmonary venous connection and functionally single ventricle. Cardiol Young. 2009;19(6):594–600. DOI:10.1017/S1047951109991296.
- Noecker AM, Chen JF, Zhou Q, et al. Development of patient-specific three-dimensional pediatric cardiac models. ASAIO J. 2006;52(3):349–353. DOI:10.1097/01.mat.0000217962.98619.ab.
•• Describes the feasibility of developing pediatric heart models derived from CT data sets for use in medical device development.
- Farooqi KM, Sengupta PP. Echocardiography and three-dimensional printing: sound ideas to touch a heart. J Am Soc Echocardiogr. 2015;28(4):398–403. DOI:10.1016/j.echo.2015.02.005.
- Costello JP, Olivieri LJ, Krieger A, et al. Utilizing three-dimensional printing technology to assess the feasibility of high-fidelity synthetic ventricular septal defect models for simulation in medical education. World J Pediatr Congenit Heart Surg. 2014;5(3):421–426. DOI:10.1177/2150135114528721.
- Kim MS, Hansgen AR, Wink O, et al. Rapid prototyping: a new tool in understanding and treating structural heart disease. Circulation. 2008;117(18):2388–2394. DOI:10.1161/CIRCULATIONAHA.107.740977.