Abstract
Ezetimibe is a cholesterol absorption inhibitor that blocks the intestinal absorption of both biliary and dietary cholesterol. It appears to exert its effect by blocking intestinal sterol transporters, specifically Niemann–Pick C1-like 1 proteins, thereby inhibiting the intestinal absorption of cholesterol, phytosterols and certain oxysterols. Ezetimibe monotherapy and in combination with statin therapy is primarily indicated for lowering LDL-cholesterol levels. In addition, it may favorably affect other parameters that could potentially further reduce atherosclerotic coronary heart disease risk, such as raising HDL-cholesterol and lowering levels of triglycerides, non-HDL-cholesterol, apolipoprotein B and remnant-like particle cholesterol. Further effects of ezetimibe include a reduction in circulating phytosterols and oxysterols and, when used in combination with statins, a reduction in high-sensitivity C-reactive protein. The clinical significance of the LDL-cholesterol lowering and other effects of ezetimibe is being evaluated in clinical outcome studies.
ApoB: Apolipoprotein B; CE: Cholesterol ester; CETP: Cholesteryl ester transfer protein; chol: Cholesterol; CM: Chylomicron;
CMr: Chylomicron remnant; IDL: Intermediate-density lipoprotein; LCAT: Lecithin-cholesterol acetyltranferase; NPC1L1: Niemann–Pick C1-like 1 protein; SR-BI: Scavenger receptor type BI; TG: Triglyceride; VLDL: Very-low-density lipoprotein.
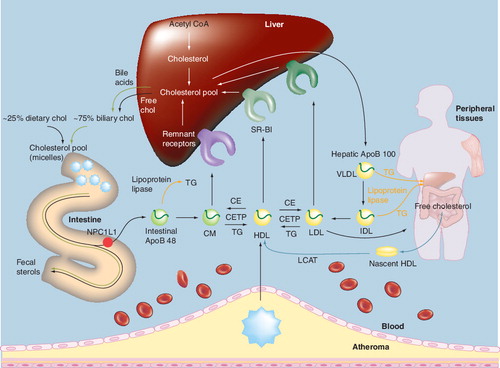
Adapted with permission from Citation[2].
![Figure 2. Hepatic cholesterol synthesis and lipoprotein packaging.Adapted with permission from Citation[2].](/cms/asset/219fe484-022c-480e-bb65-e2bfc20a3f38/ierk_a_11210377_f0002_b.jpg)
The esterification and lipoprotein packaging of cholesterol by the liver (hepatocyte) and intestine (enterocyte), occur intracellularly. Peripheral cholesterol transport via HDL particles occurs in the plasma.
ACAT: Acyl-CoA:cholesterol acyl transferase; CETP: Cholesteryl ester transfer protein; LCAT: Lecithin-cholesterol acyltransferase; MTP: Microsomal triglyceride transfer protein; VLDL: Very-low-density lipoprotein.
Reproduced with permission from Citation[2].
![Figure 3. Analogy of free cholesterol packaging into apolipoprotein B-containing lipoproteins in peripheral tissue, liver and intestine.The esterification and lipoprotein packaging of cholesterol by the liver (hepatocyte) and intestine (enterocyte), occur intracellularly. Peripheral cholesterol transport via HDL particles occurs in the plasma.ACAT: Acyl-CoA:cholesterol acyl transferase; CETP: Cholesteryl ester transfer protein; LCAT: Lecithin-cholesterol acyltransferase; MTP: Microsomal triglyceride transfer protein; VLDL: Very-low-density lipoprotein.Reproduced with permission from Citation[2].](/cms/asset/a3937dbc-aae4-4145-8035-e73a97d23e33/ierk_a_11210377_f0003_b.jpg)
ABCA1: Adenosine triphosphate-binding cassette transporter; ApoA-I : Apolipoprotein A-I; B100: Apolipoprotein B-100-containing lipoproteins; B48: Apolipoprotein B-48-containing lipoproteins; CE: Cholesterol ester; CETP: Cholesteryl ester transfer protein; CM: Chylomicron; CMr: Chylomicron remnant; FC: Free cholesterol; IDL: Intermediate-density lipoprotein; LCAT: Lecithin-cholesterol acyltransferase; LDLR: LDL receptor; NPC1L1: Niemann–Pick C1-like 1 protein; SR-BI: Scavenger receptor type BI; TG: Triglyceride; VLDL: Very-low-density lipoprotein.
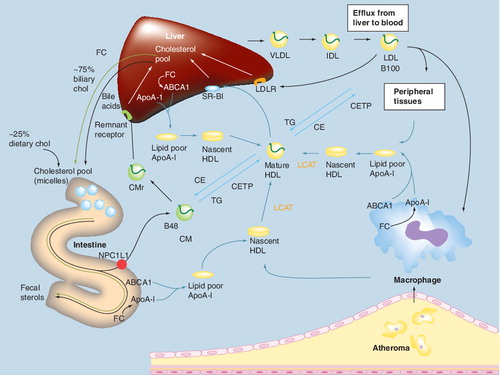
ACAT: Acyl-CoA:cholesterol acyltransferase; NPC1L1: Niemann–Pick C1-like 1 protein.
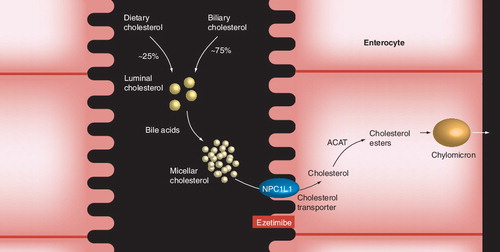
ApoA-I: Apolipoprotein A-I; B100: Apolipoprotein B-100-containing lipoproteins; B48: Apolipoprotein B-48-containing lipoproteins; CE: Cholesterol ester; CETP: Cholesteryl ester transfer protein; chol: Cholesterol; CM: Chylomicron; CMr: Chylomicron remnant; IDL: Intermediate-density lipoprotein; LCAT: Lecithin-cholesterol acetyltransferase; LDLR: LDL receptor; NPC1L1: Niemann–Pick C1-like 1 protein; SR-BI: Scavenger receptor type BI; TG: Triglyceride; VLDL: Very-low-density lipoprotein.
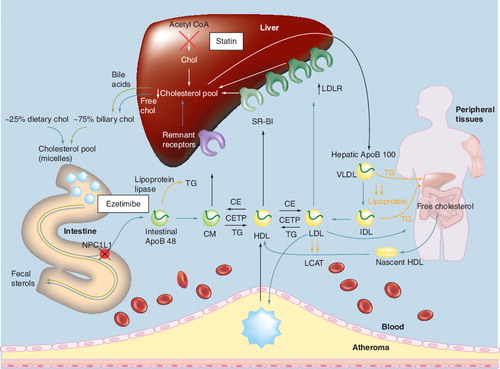
*p < 0.05 versus placebo.
EZE: Ezetimibe.
Reproduced with permission from Citation[51].
![Figure 7. Dose–response effect of ezetimibe monotherapy.*p < 0.05 versus placebo.EZE: Ezetimibe.Reproduced with permission from Citation[51].](/cms/asset/0019f423-ddd6-4f6d-9094-a719edff1894/ierk_a_11210377_f0007_b.jpg)
*p < 0.001; **p < 0.05; ***p < 0.01.
HDL-C: High-density lipoprotein-cholesterol; LDL-C: Low-density lipoprotein-cholesterol; TG: Triglyceride.
Reproduced with permission from Citation[54].
![Figure 8. Ezetimibe add-on to statin therapy.*p < 0.001; **p < 0.05; ***p < 0.01.HDL-C: High-density lipoprotein-cholesterol; LDL-C: Low-density lipoprotein-cholesterol; TG: Triglyceride.Reproduced with permission from Citation[54].](/cms/asset/57b7b454-1c5e-4219-ba1f-9259f3d7450b/ierk_a_11210377_f0008_b.jpg)
*p < 0.001 EZE/SIMVA versus same dose SIMVA.
**p < 0.05 EZE/SIMVA versus same dose SIMVA.
‡p < 0.05 EZE/SIMVA versus next highest dose SIMVA.
EZE: Ezeteimibe; RLP-C: Remnant-like particle cholesterol; SIMVA: Simvastatin.
Adapted with permission from Citation[65].
![Figure 10. Effect of ezetimibe, simvastatin and ezetimibe/simvastatin on RLP-C.*p < 0.001 EZE/SIMVA versus same dose SIMVA.**p < 0.05 EZE/SIMVA versus same dose SIMVA.‡p < 0.05 EZE/SIMVA versus next highest dose SIMVA.EZE: Ezeteimibe; RLP-C: Remnant-like particle cholesterol; SIMVA: Simvastatin.Adapted with permission from Citation[65].](/cms/asset/638c8846-c6c1-4b2d-8c6f-94c3bca74598/ierk_a_11210377_f0010_b.jpg)
Adapted with permission from Citation[122].
![Figure 11. Cardiovascular disease risk factors and association with phytosterols and cholesterol synthesis precursors.Adapted with permission from Citation[122].](/cms/asset/484a53f9-0697-490d-8347-788693d0886a/ierk_a_11210377_f0011_b.jpg)
* p < 0.001 versus placebo.
‡p < 0.001 versus pooled SIMVA.
§p < 0.05 versus EZE.
¶p < 0.001 versus EZE.
#p < 0.001 versus pooled ATORVA.
ATORVA: Atorvastatin; EZE: Ezetimibe; SIMVA: Simvastatin.
Adapted with permission from Citation[129].
![Figure 12. Ezetimibe and statin effect on phytosterol concentrations.* p < 0.001 versus placebo.‡p < 0.001 versus pooled SIMVA.§p < 0.05 versus EZE.¶p < 0.001 versus EZE.#p < 0.001 versus pooled ATORVA.ATORVA: Atorvastatin; EZE: Ezetimibe; SIMVA: Simvastatin.Adapted with permission from Citation[129].](/cms/asset/01c7e0f0-c7cf-47a3-b1e5-52ab21cd9295/ierk_a_11210377_f0012_b.jpg)
*p < 0.001 versus placebo.
‡p < 0.05 versus placebo.
§p < 0.05 versus EZE.
¶p < 0.001 versus EZE
ATORVA: Atorvastatin; EZE: Ezetimibe; SIMVA: Simvastatin.
Adapted with permission from Citation[129].
![Figure 13. Ezetimibe and statin effects on cholesterol precursors.*p < 0.001 versus placebo.‡p < 0.05 versus placebo.§p < 0.05 versus EZE.¶p < 0.001 versus EZEATORVA: Atorvastatin; EZE: Ezetimibe; SIMVA: Simvastatin.Adapted with permission from Citation[129].](/cms/asset/d8eeb0fd-a86f-4ae6-8403-d39ac48077a7/ierk_a_11210377_f0013_b.jpg)
Reducing LDL-cholesterol (LDL-C) levels by administering statins reduces atherosclerotic coronary heart disease (CHD) risk and cardiovascular disease (CVD)-associated events by approximately 20–40% Citation[1–3]. Further reduction of CHD risk in high-risk individuals probably requires more aggressive LDL-C lowering for longer periods of time than studied by existing CHD outcomes trials. It is possible that it is the degree of LDL-C lowering, and not the means by which it is achieved, that may be most important in reducing CHD events Citation[4]. Therefore, combining lipid-altering drug therapies with complementary mechanisms of action for the purpose of further LDL-C lowering has been recommended as a therapeutic approach for high CHD-risk individuals Citation[1]. Combination lipid-altering therapies may also improve other CHD-associated risk factors that go beyond LDL-C levels.
Ezetimibe is an intestinal cholesterol absorption inhibitor that, in both monotherapy and combination therapy, significantly reduces LDL-C levels Citation[5,6]. Furthermore, ezetimibe may have additional effects. This review describes the cholesterol-lowering effects of ezetimibe, as well as other effects that may potentially reduce other CHD risk factors.
Sources of plasma cholesterol
To better describe the inter-relationship of the complementary effects that lipid-altering drugs may have on CHD risk reduction, it is important to understand clinically applicable cholesterol physiology. Simply stated, plasma cholesterol is derived from two main sources in the body: cholesterol biosynthesis (peripheral and hepatic) and intestinal cholesterol absorption Citation[2,5,7]. The relative contribution of cholesterol from these sources depends upon various other factors, including genetic predisposition, diet and drug therapies Citation[5,8,9].
Cholesterol biosynthesis
Animal data suggest that most cholesterol synthesis occurs in peripheral tissues such as intestine, muscle and skin, with the greatest amount per gram of tissue produced in endocrine organs (e.g., ovary and adrenal glands) Citation[5,10,11]. This is because cholesterol is an important component of cell membrane stability and serves as the backbone structure for steroidogenesis. In addition to its critical regulatory role in cholesterol homeostasis, the liver is also an important organ with regard to cholesterol synthesis and excretion into the intestine. Finally, cholesterol derived from vascular endothelial macrophages associated with arterial plaques is a clinically important, but qualitatively minor contributor to total circulating cholesterol levels Citation[7,10,12].
In both peripheral tissues and the liver, cholesterol is synthesized through a series of enzymatic steps. The major precursor for the synthesis of cholesterol is acetyl coenzyme A (acetyl-CoA), which gives rise to hydroxyl-methylglutaryl coenzyme A (HMG-CoA) . The rate-limiting step in the cholesterol biosynthetic pathway is the conversion of HMG-CoA to mevalonic acid, which is catalyzed by HMG-CoA-reductase. Once synthesized from mevalonic acid, hepatic cholesterol is esterified by the action of acyl-CoA-cholesterol acyl transferase (ACAT) and incorporated into apolipoprotein B (ApoB)-100-containing very-low-density lipoprotein (VLDL) via microsomal triglyceride transfer protein (MTP). It is then secreted into the plasma for transport to peripheral tissues . In the blood, VLDL particles are further metabolized through hydrolysis of triglycerides (TG) by lipases, yielding intermediate-density lipoprotein (IDL) particles, some of which are cleared by the liver, with the remainder being converted to LDL particles – the major carrier of cholesterol in the plasma .
Cholesterol synthesis in peripheral tissues also contributes to the hepatic cholesterol pool through the transfer of cholesterol to the liver in a process mediated by HDL particles (peripheral, or sometimes referred to as ‘reverse’ cholesterol transport) Citation[13]. Free cholesterol is transferred from peripheral tissues to pre-β-HDL-C particles via the ATP-binding cassette transporter 1 (ABCA1) pathway. These nascent ApoA-I-containing lipoproteins undergo remodeling to become mature HDL-C in the plasma through esterification of the free cholesterol by lecithin-cholesterol acetyltransferase (LCAT). Circulating, mature HDL particles may undergo holoparticle uptake by the liver via a putative receptor, or the cholesterol from HDL particles may be transferred to the liver after interaction with the hepatic scavenger receptors (SR-BI). Once in the liver, the cholesterol delivered by HDL particles may be utilized for hepatic cholesterol needs, converted into bile acids, or excreted into bile and eliminated through the feces Citation[2,14]. In addition to delivery to the liver, circulating HDL particles may transfer cholesterol to ApoB-containing atherogenic lipoproteins (such as LDL, VLDL, IDL and chylomicron remnants) through cholesteryl ester transfer protein (CETP). The cholesterol associated with LDL particles may be taken up by the liver via hepatic LDL receptors Citation[2,14], which represents another route for peripheral tissue cholesterol and HDL-C delivery to the liver.
Although most of the cholesterol produced in the body may be derived from peripheral tissues, the major organ that regulates the amount of circulating cholesterol is the liver, through its cholesterol synthesis and packaging into lipoproteins, and through up- or downregulation of its LDL receptors Citation[5,15]. Through the process of hepatic LDL receptor-mediated endocytosis, LDL particles and other ApoB-lipoproteins are removed from the blood and taken into hepatocytes, where they are catabolized to release cholesterol. Free cholesterol can be stored in these cells as cholesterol ester, the concentration of which plays a role in regulating cholesterol synthesis and hepatic LDL receptor activity, thus helping to regulate circulating levels of LDL-C. In humans, the cholesterol eliminated by fecal excretion is equally derived from cholesterol that is converted to acidic steroids (such as bile acids) and neutral steroids (such as cholesterol itself).
Whether from lipoprotein-mediated hepatic uptake, endogenous hepatic synthesis, or delivery from intestinal absorption, the hepatic cholesterol pool directs the degree to which cholesterol is metabolized Citation[16]. During times of higher hepatic cholesterol availability, LDL receptors may become down-regulated and circulating cholesterol levels are increased. When hepatic cholesterol availability is reduced, LDL receptors may become upregulated and circulating cholesterol levels are decreased.
Intestinal cholesterol absorption
Intestinal sterol absorption is an important factor towards determining circulating cholesterol levels Citation[5,9,10]. It has been suggested that dietary sterols are not a major contributor to circulating cholesterol and other sterols, except during times of very high cholesterol consumption; however, this is not to suggest that intestinal cholesterol absorption is physiologically unimportant Citation[10]. This is because up to three-quarters of the cholesterol delivered to the intestine is derived from biliary cholesterol excretion from the liver and, thus, intestinal cholesterol absorption is a clinically relevant mechanism that significantly contributes to hepatic cholesterol.
In the intestinal lumen, both biliary and dietary cholesterol undergo micellar adaptation by bile acids, which allows for enhanced transport through the brush border membranes into intestinal epithelial cells . Free cholesterol within intestinal cells may then return to the intestinal lumen through adenosine-binding cassette (ABC) transporters G5 and G8 Citation[17], or alternatively be esterified through ACAT and packaged into chylomicron particles within intestinal epithelial cells by MTP in a metabolic process somewhat analogous to that which occurs in the liver and peripheral tissue ( & ). Chylomicrons are then transported through the lymphatic system and subsequently to the blood where cholesterol may be delivered to peripheral tissue and the liver Citation[18]. During this process, removal of TG from chylomicrons by lipases results in the formation of cholesterol-rich chylomicron remnant particles that are taken up by the liver and added to the hepatic pool.
Genetic mutations resulting in the dysfunction of ABCG5 and ABCG8 transporters are linked to sitosterolemia – an inheritable disease characterized by an accumulation of phytosterols resulting from a net increase in intestinal absorption and possibly decreased biliary removal Citation[19,20]. Hyperabsorption of both plant sterols and cholesterol in sitosterolemic patients consequently leads to increased plasma and tissue levels of plant sterols, which can result in the development of xanthomas and premature coronary heart disease, even in the absence of significant hypercholesterolemia Citation[21]. It appears that these transporters may also promote biliary secretion of cholesterol in mice Citation[22], but the relation of this to cholesterol metabolism in humans is not known.
Although the liver is recognized as the major regulator of cholesterol metabolism, the intestine also appears to play an important role in cholesterol flux and maintenance of plasma cholesterol homeostasis Citation[16]. In addition to its role in cholesterol absorption, the intestine acts as an excretion organ and may contribute directly to fecal neutral sterol (cholesterol) excretion Citation[23]. Another way wherein the intestine contributes to cholesterol flux is through HDL particle biogenesis Citation[16]. In rats, almost all of the total plasma ApoA-I (the principal apolipoprotein of HDL-C) is produced by the liver and intestine, up to 56% of which is synthesized and secreted by the intestine Citation[24,25]. The intestine also expresses significant levels of the transporter, ABCA1 (critical to the formation of HDL particles), and in mice approximately 30% of total plasma HDL-C is contributed by intestinal ABCA1 Citation[24].
Nonesterified sterols and phospholipids in enterocytes transported by ABCA1 to lipid-free ApoA-I are assembled into nascent pre-β-HDL particles. Secretion of nascent, pre-β-HDL particles by the intestine into the circulation promotes cholesterol and phospholipid efflux from peripheral cells to these particles Citation[13,16], and thus may enhance cholesterol flux to the liver.
Relationship between cholesterol synthesis & intestinal absorption
Cholesterol homeostasis is maintained through the processes of cholesterol synthesis and metabolism and is influenced by physiologic responses to fluctuations in dietary cholesterol intake. An inter-relationship exists between cholesterol absorption and biosynthesis Citation[5,8,15]. When the intestinal absorption of cholesterol is reduced, less cholesterol is delivered to the liver, resulting in a compensatory rise in the rate of hepatic cholesterol synthesis and an increase in the number and activity of hepatic LDL surface receptors. Conversely, when more intestinal cholesterol is absorbed, cholesterol synthesis and LDL receptor activity are downregulated. Some studies have suggested that when cholesterol synthesis is reduced with a statin, a reciprocal increase in cholesterol intestinal absorption appears to take place as indicated by increased blood levels of phytosterols; however, other studies have not shown this compensatory effect. This inconsistency is perhaps attributable to differing methodologies used to assess cholesterol intestinal absorption Citation[8].
Mechanisms of action
Ezetimibe monotherapy
Ezetimibe is a member of a class of lipid-altering agents known as cholesterol absorption inhibitors Citation[27]. Ezetimibe blocks the intestinal absorption of both cholesterol and phytosterols through its interaction with the sterol transporter, Niemann–Pick C1-like 1 protein (NPC1L1), located on the brush border membrane of intestinal epithelial cells Citation[26–28], although other proteins may also be involved Citation[29]. The NPC1L1 transporter is required for the transport of intestinal cholesterol and phytosterols from the intestinal lumen to the intracellular compartments of enterocytes, where sterol esterification and incorporation into chylomicron particles takes place Citation[26–28]. Ezetimibe may also interact with hepatic NPC1L1 proteins, which are highly expressed in human liver cells and thought to regulate biliary cholesterol concentration and, thus may potentially affect circulating cholesterol levels Citation[30].
In animal models, inhibition of intestinal cholesterol absorption by ezetimibe results in a reduction of the cholesterol content packaged into chylomicrons, which subsequently decreases the amount of cholesterol delivered by chylomicrons to the liver Citation[31]. This reduction in cholesterol delivered to the liver results in a compensatory upregulation of hepatic LDL receptors, thereby enhancing LDL-C clearance and lowering plasma levels of LDL-C Citation[32–34]. In monkeys fed a high-fat, high-cholesterol diet, ezetimibe treatment substantially reduced the cholesterol ester content of postprandial chylomicrons but had no effect upon the levels of ApoB-48 (the major structural protein of intestinally derived lipoprotein particles), indicating that ezetimibe depleted cholesterol from chylomicrons without reducing the number of chylomicron particles Citation[31]. Although ApoB-48 levels remained unchanged, levels of ApoB-100 (a large hepatic glycoprotein critical to the assembly, secretion, and intravascular transport of VLDL, IDL and LDL) were reduced by ezetimibe treatment, suggesting that decreased delivery of cholesterol to the liver ultimately leads to enhanced clearance Citation[35] and/or reduced production of atherogenic lipoproteins Citation[31]. These effects of ezetimibe are supported by an ApoB-48 and -100 kinetic study in men with primary hypercholesterolemia Citation[35], which has also suggested that ezetimibe monotherapy does not have a significant impact on ApoB-48 kinetics, although the catabolic rate of ApoB-100-containing VLDL, IDL and LDL may be increased.
In the LDL r-/- (homozygous null receptor) mouse model, virtually none of the circulating cholesterol is taken up by tissues, and changes in circulating LDL-C reflect only the rate of LDL production. In this model, ezetimibe reduces the production of VLDL and LDL Citation[34]. Ezetimibe also induces an increase in the expression of LDL receptors in LDL r+/+ mice. In patients with homozygous familial hypercholesterolemia, an autosomal-dominant inherited disorder generally caused by mutations in LDL receptor genes Citation[36], ezetimibe significantly reduces LDL-C levels, when added to ongoing statin therapy Citation[37]. In totality, these data support increased clearance and reduced hepatic production as important mechanisms of ezetimibe in reducing circulating LDL-C and other atherogenic lipoprotein levels.
Ezetimibe plus statin
Statins and ezetimibe represent two complementary lipid-altering drugs that, through dual inhibition, lower LDL-C levels. Extensive evidence supports the use of statins for LDL-C lowering and CVD event reduction Citation[38]. Statins inhibit hepatic cholesterol synthesis, which upregulates LDL receptors, resulting in increased clearance of ApoB-containing atherogenic lipoproteins, such as LDL and VLDL, from the plasma Citation[32,39]. Enhanced receptor clearance of other lipoproteins may also mediate reductions in ApoB-48-containing chylomicrons Citation[39,40]. Decreased hepatic cholesterol synthesis also reduces VLDL synthesis and secretion Citation[15,39], and the amount of biliary cholesterol delivered to the intestine, which, in turn, may upregulate intestinal cholesterol absorption Citation[39,41–43].
Ezetimibe inhibits intestinal cholesterol absorption and results in increased intestinal and liver cholesterol synthesis in mice, which could potentially lead to a partial attenuation of LDL-C lowering Citation[26,34,44]. Thus, reducing cholesterol absorption with ezetimibe and reducing cholesterol synthesis with statins provide complementary mechanisms of action. Several studies have demonstrated that when administered with a statin, ezetimibe provides at least additive improvements in lowering LDL-C, non-HDL-C, TG and ApoB, and in raising HDL-C levels over that of statins alone Citation[6,45].
The combination of ezetimibe with statins upregulates LDL receptors and significantly reduces LDL-C and ApoB levels Citation[46]. In a miniature pig model of cholesterol metabolism, coadministration of simvastatin resulted in significant reductions of ezetimibe-induced hepatic cholesterol synthesis and a marked increase in hepatic LDL receptor expression Citation[46]. The combination also decreased ApoB-100 levels, attributed to reduced VLDL production and enhanced LDL r-mediated clearance of LDL-C. Ezetimibe-induced expression of LDL receptors and the subsequent increased clearance of both ApoB-100- and -48-containing lipoproteins may account for lowering of both cholesterol and tri-glyceride levels, particularly when combined with statin therapy.
LDL-C-lowering effects of ezetimibe monotherapy & combination with statins in clinical trials
In clinical studies, ezetimibe (10 mg) monotherapy significantly reduces LDL-C levels in hypercholesterolemic patients by -17.2 to -22.3% (p < 0.01 to < 0.001) compared with placebo Citation[47–50]. Doses of 20 and 40 mg ezetimibe were well tolerated in early trials; however, these doses provided minimal additional lipid-altering benefit Citation[51,52]. Hence, ezetimibe 10 mg once a day is the dose indicated for the treatment of hypercholesterolemic patients Citation[53].
In combination therapy, when added to statins, ezetimibe reduces LDL-C levels significantly beyond those attainable with statins alone (-5.9 to -21.0%; p < 0.05 to < 0.001) in hypercholesterolemic patients ( & ) Citation[54–64]. The ezetimibe/simvastatin combination tablet (Vytorin®) also significantly reduces LDL-C levels in an additive manner, -4.2 to -14.0% (p < 0.001) beyond that of simvastastin, atorvastatin or rosuvastatin alone Citation[65–67]. The enhanced LDL-C lowering observed when ezetimibe is added to statins is due to the aforementioned complementary action of the two agents.
Finally, ezetimibe significantly reduces LDL-C levels when added to ongoing, stable, statin therapy in patients with metabolic syndrome (-16.7 and -21.4%) and Type 2 diabetes mellitus (T2DM) (-26.1 and -24.8%) compared with placebo (p < 0.001 for both) Citation[68,69]. Similarly, in T2DM patients, the ezetimibe/simvastatin combination tablet significantly lowers LDL-C from baseline compared with atorvastatin at all dose comparisons (-6.6 to -15.3%; p < 0.001) Citation[70].
Ezetimibe: lipid effects beyond LDL-C lowering
Optimal CHD risk reduction may best be achieved by modification of multiple CHD risk factors Citation[20]. Nonattainment of optimal LDL-C levels, coupled with unfavorable HDL-C and/or TG levels, increases the adjusted risk of CHD events Citation[20]. In addition to LDL-C lowering, ezetimibe favorably alters the levels of other parameters considered to be associated with CHD risk, such as TG, HDL-C, non-HDL-C, ApoB, high-sensitivity C-reactive protein (hsCRP) and RLP-C Citation[1,71].
Non-HDL-C
Non-HDL-C sums cholesterol carried by atherogenic lipoproteins such as ApoB-100-containing LDL, VLDL, IDL, RLP, lipoprotein [Lp(a)] and, in certain instances, ApoB-48-containing chylomicrons Citation[72]. Thus, non-HDL-C, a measure of total cholesterol minus HDL, is thought to be a better predictor of CHD risk than LDL-C alone Citation[72]. As all these lipoproteins have the potential to deliver cholesterol into the arterial wall, non-HDL-C reflects a degree of atherogenic risk not quantified by measurement of LDL-C alone Citation[73]. It is for this reason that the National Cholesterol Education Program (NCEP) ATP III has recommended non-HDL-C treatment goals, set at levels 30 mg/dl above the respective LDL-C treatment goals, as a secondary target for high-risk patients with TG of more than 200 mg/dl Citation[1,74]. Similarly, AHA/ACC guidelines recommend that non-HDL-C should be reduced to less than 130 mg/dl in patients with TG of more than 200–499 mg/dl, and further reduction to less than 100 mg/dl in these patients is reasonable Citation[71].
Data from animal studies have shown that reduced intestinal absorption with ezetimibe monotherapy decreases non-HDL-C levels Citation[73]. In a pooled analysis, ezetimibe itself and in the monotherapy arms of combination studies reduced non-HDL-C significantly more than placebo (-19.7%; p < 0.001) in patients with mild-to-moderate hypercholesterolemia Citation[75]. Ezetimibe has also shown favorable effects upon non-HDL-C when added to statins . In add-on to statin therapy studies, ezetimibe reduced non-HDL-C from baseline significantly more (-19.9% to -25.3%; p < 0.001) than placebo Citation[54–56]. Ezetimibe added in combination with statins also significantly reduced non-HDL-C (-4.6 to -19.0%; p < 0.05 to < 0.001) compared with statins alone at various doses and across pooled doses Citation[57–61,64]. The ezetimibe/simvastatin combination tablet reduced non-HDL-C levels from baseline significantly more than simvastatin and ro-suvastatin (-12.7 and -4.1%, respectively; p < 0.001) Citation[65,67].
Measurement of non-HDL-C in patients with metabolic syndrome and T2DM is particularly relevant as these patients tend to have high amounts of atherogenic-type lipoproteins often found with elevated TG, TG-rich lipoproteins (TRL), and ApoB and small, dense LDL particles Citation[76], which may not be fully realized by measuring LDL-C levels alone. When ezetimibe was added to ongoing, stable statin therapy in patients with metabolic syndrome, non-HDL-C was significantly reduced by -15.4 and -19.5% (p < 0.001) and in T2DM patients by -23.8 and -21.8% (p < 0.001) compared with statins alone across pooled doses Citation[68,69]. In T2DM patients, the combination ezetimibe/simvastatin 10/20 mg provided significant additional reductions in non-HDL-C levels of -13.1 and -6.7% (p < 0.001 compared with atorvastatin 10 and 20 mg, respectively) and of -5.6% with ezetimibe 10/40 mg versus atorvastatin 40 mg (p < 0.001) Citation[70].
Reductions in LDL-C, VLDL-C, and RLP-C levels account for the ezetimibe-induced decreases in plasma non-HDL-C levels Citation[65,76], attributed to its mechanism of action described for lowering of levels of LDL-C and TG (below), and of ApoB (below). Ezetimibe has not been shown to reduce Lp(a) levels Citation[49,54,57,58,60,64].
HDL cholesterol
Levels of HDL-C correlate with CHD risk Citation[1,74]. Therefore, raising HDL is recommended by some organizations in patients at higher risk for CHD Citation[74,77].
Ezetimibe monotherapy elicits modest increases in HDL-C levels. In several monotherapy trials, ezetimibe 5 and 10 mg provided additional increases in HDL-C from baseline levels compared with placebo (2.2%; p > 0.05 and 2.3 to 3.5%; p < 0.05 to < 0.01; ) Citation[47–50]. Ezetimibe in combination with statins is also associated with modest further improvements in HDL-C levels, typically greater than those achieved with statins alone . Addition of ezetimibe 10 mg to stable, ongoing statin therapy in patients with primary hypercholesterolemia resulted in significant additional increases from baseline in HDL-C pooled across doses in comparison to placebo (1.7 and 2.1%, p < 0.05 to < 0.001; and 2.6%, p > 0.05) Citation[54–56]. Coadministration of ezetimibe and statins increases HDL-C levels more than statins alone at various doses and across pooled doses, with several data points achieving statistical significance (0.6 to 5.8%; p < 0.05 to < 0.001) Citation[57–64]. Increases in HDL-C from baseline were somewhat higher for pooled doses of the combination tablet ezetimibe/simvastatin compared with simvastatin (0.4%; p > 0.05) Citation[65]. When averaged across doses, ezetimibe/simvastatin significantly increased HDL-C levels versus atorvastatin (3.6%; p < 0.001) Citation[66] and versus rosuvastatin, increases were comparable Citation[67].
When added to stable, ongoing statin therapy, ezetimibe significantly increased HDL levels in patients with metabolic syndrome by 2.6% (p < 0.05), versus placebo addition, and provided comparable HDL-C increases in T2DM patients Citation[68]. In another study, ezetimibe added to ongoing statin therapy significantly increased levels of HDL-C by 2.8 and 2.7%, compared with placebo (p = 0.002 and p < 0.001) in patients with metabolic syndrome and T2DM, respectively Citation[69]. Treatment with ezetimibe/simvastatin 10/20 mg significantly increased HDL-C levels by 3.6 and 3.4% more than atorvastatin 10 and 20 mg (p ≤ 0.001), and with ezetimibe 10/40 mg by 4.0% v-ersus atorvastatin 40 mg (p < 0.001) in T2DM patients Citation[70].
It is possible that the modest increases in HDL-C levels induced by ezetimibe may be attributed to its reduction in the delivery of cholesterol from the intestine to the liver, which subsequently enhances the clearance of TRL. A reduction in the levels of TRL, which are acceptors of cholesterol transferred from HDL particles by CETP, may contribute to a reduced rate of cholesterol transfer, thereby increasing levels of HDL-C Citation[78,79].
Ezetimibe inhibition of cholesterol absorption may also enhance peripheral cholesterol transport through potential mechanisms, which may not be readily linked to HDL-C raising. The intestine and liver both contribute to plasma levels of ApoA-I Citation[24] and synthesize significant levels of ABCA1, which mediates the flux of free cholesterol and phospholipids toward nascent ApoA-I in the formation of HDL particles. In mice deficient in intestinal-specific ABCA1, plasma HDL-C levels were reduced and ABCA1 was shown to be responsible for the direct lipidation of lipid-poor ApoA-1-containing particles Citation[24,80]. Moreover, the absence of intestinal ABCA1 resulted in a decreased transport of dietary cholesterol into HDL particles. Since nascent HDL particles secreted by the intestine contain nonesterified sterols and free cholesterol transported by ABCA1 from the enterocyte, ezetimibe inhibition of cholesterol absorption may increase the proportion of lipid-free intestinal ApoA-I available, creating HDL particles with greater capacity to take up more cholesterol, thereby promoting cholesterol and phospholipid efflux from peripheral cells Citation[13,16].
In a similar manner, chylomicrons may also play a role in promoting HDL-mediated flux of cholesterol from peripheral tissues Citation[81]. These large, TG-rich particles deliver cholesterol to the liver via ApoE receptors. It is possible that ezetimibe-induced reduction of the cholesterol content of the chylomicrons may enhance the potency of chylomicrons as acceptors of cholesterol ester in the HDL-mediated flux of cholesterol. Potentially, enhanced HDL flux resulting in greater delivery of cholesterol to the liver is supported by findings that ezetimibe and ezetimibe/simvastatin treatment resulted in increased fecal sterol excretion in humans Citation[82]. Ezetimibe also promoted the excretion of macrophage and HDL-derived cholesterol in the feces of mice, but had no effect on HDL metabolism Citation[83]. This may be attributed not only to its blockade of intestinal cholesterol and sterol transport, but also to enhanced HDL-mediated cholesterol flux.
Triglycerides
Hypertriglyceridemia is considered to be an important risk factor, although it is not clear if elevated triglyceride levels are always an independent CHD risk factor and whether the lowering of TG levels is beneficial in reducing CVD risk Citation[38,84,85]. If after LDL-C goals are reached, and TG levels are still elevated (>200 mg/dl), then it is recommended that non-HDL-C levels be assessed and specific non-HDL-C treatment goals be achieved Citation[1,74].
In monotherapy studies, ezetimibe (10 mg) generally decreased TG levels compared with placebo (-2.7 and -4.1%, p > 0.05; and -11.4%, p < 0.01; ) Citation[47,49,50]. When coadministered with various doses of statins, ezetimibe resulted in greater additional reductions in TGs levels compared with placebo (-11.4 to -13.8%; p < 0.001) and statins alone across pooled doses (-7.4% to -12.8%; p < 0.01 to < 0.001) and at milligram-equivalent doses (0.0 to -11.0%; p > 0.05 to < 0.001) Citation[57–64]. The combination ezetimibe/simvastatin tablet al.o reduces TG levels versus simvastatin (-3.5%, p < 0.001), atorvastatin (-1.4%; p > 0.05), and rosuvastatin (-2.7%; p < 0.001) when compared across pooled doses Citation[65–67].
TG levels are often elevated in patients with metabolic syndrome and T2DM. The coadministration of ezetimibe and statins provides additional significant reductions in TG levels from baseline in these patients. When ezetimibe was added to stable, ongoing statin therapy, TG levels were significantly reduced compared with statins alone in patients with metabolic syndrome by an additional -6.5 to -10.7% (p < 0.001) and in T2DM patients by -10.9 to -12.3% (p < 0.001) Citation[68,69]. The combination tablet, ezetimibe/simvastatin 10/20 mg, reduced TG levels significantly more than atorvastatin at 10 mg (-4.4%; p = 0.02), with comparable reductions at 10/20 mg ezetimibe/simvastatin versus atorvastatin 20 mg and 10/40 mg ezetimibe/simvastatin versus atorvastatin 40 mg in T2DM patients Citation[70].
The mechanism of ezetimibe-induced reduction of TG levels is unclear. Reductions in plasma and hepatic TG are observed in mice and hamsters administered ezetimibe Citation[34]. Given that ezetimibe does not block fat absorption, decreased TG levels are likely attributed to a secondary effect resulting from the reduced delivery of cholesterol from the intestine to the liver, which in turn decreases VLDL production. As noted before, the clearance of TRLs is potentially increased through up-regulation of ApoB-100 and ApoB-48 receptors, resulting in an increased clearance of VLDL, chylomicrons and their respective remnants.
ApoB-100
As the majority of the total ApoB-containing lipoproteins in the plasma are associated with LDL particles, measurement of ApoB primarily reflects ApoB-100 levels Citation[86]. Similar to non-HDL-C, it has been suggested that measurement of plasma ApoB levels is a better predictor of CHD risk than LDL-C Citation[86–89] and concentrations less than 90 mg/dl may be considered an alternative secondary target for high-risk patients by the ATP III and CMA/Canadian guidelines Citation[74,90]. Non-HDL-C atherogenic lipoproteins contain ApoB, and both measurements correlate to increased CHD risk Citation[72,86], although the concordance of this in various population distributions remains unclear Citation[87,91]. Measurement of ApoB is thought by some to provide a more accurate assessment of lipoprotein atherogenicity than non-HDL-C Citation[86–89] because unlike non-HDL-C, which measures the cholesterol content of particles, ApoB provides a direct assessment of atherogenic particle number. Particle number is thought to be an important determinant of atherogenic burden and CHD risk, although the association of particle size and composition with CHD risk remains controversial Citation[72,86,88,89]. Chylomicron particles contain 1 molecule of ApoB-48, and VLDL, IDL and LDL-C particles contain one molecule each of ApoB-100. Thus, plasma ApoB levels represent the best estimate of the total number of these atherogenic lipoproteins, in particular, ApoB-100-containing lipoproteins Citation[86].
In monotherapy studies, ezetimibe was shown to reduce ApoB from baseline significantly more than placebo (-14.1 and 14.3%; p < 0.01; ) Citation[49,50]. In the monotherapy arm of a pooled analysis, ezetimibe (10 mg) decreased ApoB significantly more than placebo (-15.7%; p < 0.05) Citation[62]. When added to ongoing statin therapy, ezetimibe significantly reduced ApoB compared with placebo (-15.5 and -16.3%; p < 0.001; ) Citation[54,55]. Long-term ezetimibe treatment added to ongoing simvastatin therapy (48 weeks) was also associated with an improvement in ApoB levels compared with placebo (-13.9%, significance not assessed) Citation[56]. Similarly, ezetimibe coadministered with statins was significantly more effective in reducing ApoB levels from baseline at various dose comparisons and when pooled across doses compared with statins alone (-2.9 to -17.0%; p < 0.05 to < 0.001; ) Citation[57–64]. The ezetimibe/simvastatin combination tablet al.o reduced ApoB significantly more (p < 0.001) from baseline compared with simvastatin (-10.7%) and rosuvastatin (-2.9%) Citation[65,67].
In patients with metabolic syndrome and in patients with T2DM, measurement of ApoB is considered an important indicator of CVD risk for atherogenic lipoprotein particles Citation[1,72]. Adding ezetimibe to ongoing statin therapy significantly reduces ApoB levels compared with placebo in metabolic syndrome and T2DM patients, respectively (-13.2 and -18.1%; p < 0.001) Citation[68]. The ratio of ApoB/ApoAI, thought to be one of the most correlative predictors of CVD risk Citation[88], was significantly reduced by the addition of ezetimibe compared with placebo to ongoing statin therapy in both metabolic syndrome and T2DM patients (-16.6 and -17.7%; p < 0.001) Citation[69].
Ezetimibe may lower plasma levels of ApoB lipoproteins through its reduction of hepatic cholesterol concentrations, leading to decreased atherogenic ApoB lipoprotein production Citation[92]. Additionally, kinetic studies have shown that ezetimibe reduction of cholesterol delivery to the liver increases the hepatic uptake and catabolism of ApoB-100-containing lipoproteins VLDL, IDL and LDL Citation[46], which would further reduce ApoB levels.
High-sensitivity C-reactive protein
It is recognized that CHD is largely an inflammatory process, and inflammation is often assessed by measurements of the inflammatory marker hsCRP. Elevated hsCRP is indicative of increased CHD risk Citation[93]. CHD risk factors may contribute to inflammation and subsequent vascular injury, which eventually lead to atherosclerotic disease . CRP is an acute phase reactant protein produced primarily in the liver, which is released in response to acute injury, infection or other inflammatory stimuli, and is considered to be an emerging marker for the diagnosis and management of CHD, according to ATP III guidelines and a scientific statement from the AHA/CDC Citation[74,94].
The reduced progression of CHD associated with intensive statin treatment correlates with reductions in hsCRP levels Citation[95,96]. While ezetimibe monotherapy reduces hsCRP compared with placebo, these differences (-6.1 to 10.3%) have generally not been found to be statistically significant Citation[62,63,65,97,98]. By contrast, ezetimibe added to ongoing statin therapy for 6–8 weeks significantly reduces hsCRP (-9.7 and -10.0%; p < 0.05) compared with added placebo when pooled across doses Citation[54,55] and when assessed long-term after 48 weeks of treatment (-14.3%; significance not assessed) Citation[56]. Similarly, in a pooled analysis, ezetimibe added to a baseline of statin therapy significantly reduced hsCRP (-10.4%; p < 0.001) compared with statins alone Citation[98].
When coadministered in combination with simvastatin or atorvastatin, ezetimibe provided significant additional reductions in hsCRP compared with either statin alone (-10.0 to -24.6%; p < 0.01 to < 0.001) Citation[59,62–64], which was similar to the findings when the ezetimibe/simvastatin combination tablet was compared with simvastatin pooled across doses (-14.3%; p < 0.001) Citation[65]. The combination tablet ezetimibe/simvastatin produced additional hsCRP reductions (-0.3 and -2.9%), which were comparable to atorvastatin or rosuvastatin, respectively Citation[66,67]. In a pooled analysis of several studies, combination therapy with ezetimibe/simvastatin produced significantly greater reductions in hsCRP when compared with simvastatin (-16.7%; p < 0.001) across doses and at individual dose comparisons (-14.1 to -19.1%; p < 0.05 to < 0.01) Citation[97]. Reductions of similar magnitude were observed when compared with atorvastatin at individual doses (-4.2 to 3.2%) and across doses (-0.3%) Citation[97]. Overall, these results suggest an additional anti-inflammatory action of combining ezetimibe plus statins Citation[63].
Elevated levels of hsCRP are also associated with an increased risk of CHD in T2DM patients Citation[99]. In patients with metabolic syndrome and T2DM, ezetimibe added to a baseline of statin therapy reduced hsCRP significantly more (-6.5 and -15.4%) compared with addition of placebo (p < 0.05) Citation[68]. In another study, ezetimibe added to ongoing statin therapy reduced hsCRP significantly more (-14.8%; p < 0.001) than placebo in T2DM patients (p < 0.001), and in metabolic syndrome patients, hsCRP was further reduced by -3.2%; however, this was nonsignificant Citation[69]. In T2DM patients, ezetimibe/simvastatin 10/20 mg significantly reduced hsCRP versus atorvastatin 10 mg (-10.2%; p < 0.05) and was comparable at the other doses Citation[70].
The mechanism by which ezetimibe potentiates statin reduction of hsCRP is not known. Indirectly, this could be due to improvements in various lipid parameters, which attenuate the inflammatory process, as reflected by a reduction in markers of inflammation such as hsCRP. In single studies, statin-induced lowering of LDL-C and reductions in hsCRP correlate weakly Citation[100], though a recent meta-analysis of LDL-C lowering intervention trials showed a strong correlation between LDL-C and hsCRP Citation[101]. This suggests that different individuals may vary in achieving the amount of LDL-C lowering required to reduce inflammatory responses reflected by hsCRP Citation[102].
Although the clinical trial evidence strongly supports that the vast majority of benefit with the use of lipid-altering drugs relates to the alteration of lipids, potential nonlipid anti-inflammatory effects on hsCRP levels cannot be ruled out Citation[102]. It also cannot be discounted that hsCRP may potentially have some direct role in the pathogenesis of atherosclerosis, such as enhancing the uptake of oxidized LDL by endothelial cells and macrophages, decreasing nitric oxide production and inducing the expression of vascular cell adhesion molecules Citation[95]. Studies have indicated that ezetimibe and statins interact directly with monocytes and with macrophages, which may lead to further reductions in the pathological effects of inflammation on CHD Citation[103,104].
Remnant-like lipoproteins & lipoprotein subclass distribution
Remnant lipoproteins
Elevated levels of TRL are associated with an increased risk of CHD Citation[72,73,77]. In particular, remnant-like lipoproteins (RLPs), a subspecies of smaller, partially catabolized TRL, are thought to be atherogenic and have consequently been identified as a potential risk factor for CHD Citation[105–108]. Elevated plasma levels of RLP-cholesterol (RLP-C) are characteristic of many patient groups at increased risk for CVD Citation[107,108]. RLP-C is included in the measurement of non-HDL-C levels, a secondary treatment target after LDL-C lowering in hypertriglyceridemic patients (>200 mg/dl), as mentioned previously Citation[74]. Additionally, following isolation from plasma, RLPs can be further characterized by various methods based on density, size, charge, apolipoprotein composition and immunoabsorption Citation[108,109].
RLPs are the result of intravascular remodeling of TG-rich chylomicron and VLDL metabolic by-products into smaller and denser particles, which have higher atherogenic potential than larger, more buoyant, particles Citation[107,108]. In the bloodstream, both chylomicrons and VLDL undergo lipolysis, primarily through the action of lipoprotein lipases, in a process that removes most of the associated TG and ApoC proteins. Cholesterol ester may then be added by CETP. Remnant chylomicrons return to the liver via LDL receptor-mediated endocytosis and VLDL can be further metabolized to form IDL, which can also be taken up by the liver. The remaining IDL particles in the bloodstream are further degraded to LDL particles. Studies have indicated that the increased atherogenicity of these partially lipolyzed TRLs is attributed to an increase in arterial intima diffusion and retention, oxidative modification, enhanced endothelial cell layer permeability and longer residence time in the plasma due to reduced affinity for LDL receptors Citation[107,108].
In patients with hypercholesterolemia, ezetimibe treatment had a more pronounced effect on reducing RLP-C than placebo (-21%), although the statistical significance of this was not assessed Citation[65]. Treatment with the ezetimibe/simvastatin combination resulted in significantly greater reductions in RLP-C levels (-11.3%; p < 0.001) across pooled doses compared with the corresponding pooled doses of simvastatin Citation[65]. The ezetimibe-mediated decrease in RLP-C levels may also be related to increased catabolism of TRL, as previously noted for non-HDL-C and ApoB.
Lipoprotein subclass
Each of the five major classes of plasma lipoproteins (chylomicrons, VLDL, IDL, LDL and HDL particles) may be defined on the basis of density and/or size, apolipoprotein composition, and differing proportions of associated cholesterol and TG. These lipoprotein subclasses are further comprised of distinct, particle subclass distributions that differ in physicochemical properties, certain of which are characteristic of the atherogenic phenotype Citation[72,77,108]. Several studies have demonstrated that patients with mixed dyslipidemia often have high levels of small, dense, LDL particles, large TG-rich VLDL, small, dense, HDL particles and low levels of HDL-C Citation[110,111]. There is considerable heterogeneity in the size and density of LDL particles that range from large, buoyant to small, dense particles (the latter considered to have the most atherogenic potential) Citation[111–113]. On this basis, the LDL profile of an individual can be classified as either pattern A phenotype, characterized by a predominance of large, buoyant particles or pattern B, characterized by a predominance of small, dense LDL particles Citation[114].
Limited studies have assessed the effect of ezetimibe on lipoprotein subclass distribution. In the monotherapy arms of coadministration studies, ezetimibe itself reduced the cholesterol content of lipid subfractions (VLDL-C, IDL-C, LDL-CR, VLDL-C1+2, VLDL-C3, LDL-C subclasses) when evaluated by vertical autoprofile (VAP) analysis in patients with mixed hyperlipidemia and primary hypercholesterolemia Citation[115–117]. When combined with simvastatin, these effects were significant Citation[117]. However, ezetimibe and ezetimibe/simvastatin had minimal effect on the size and distribution of LDL particles, with modest shifts in size from small, dense to larger, more buoyant particles, assessed by segmented gradient gel electrophoresis (S3GGE) in these patients.
Because the size of TRL pools are related to lipid subfraction patterns Citation[118,119], similar to statins, the ezetimibe effect on particle size will be most pronounced in hypertriglyceridemic patients. In a recent study of patients with primary dyslipidemias, ezetimibe reduced the concentration of all LDL subfractions (tube gel, Lipoprint), with significant increases in the reduction of small, dense LDL particles and a shift toward larger and less atherogenic LDL particles, especially in patients with hypertriglyceridemia (TG > 200 mg/dl) Citation[118]. Similarly, ezetimibe coadministered with fenofibrate produced greater reductions in lipoprotein subfractions (VAP), in addition to a preferential loss in small, dense LDL particles (S3GGE), compared with fenofibrate alone Citation[115], although this was largely attributed to the TG-lowering effect of fenofibrate.
Taken together, these studies indicate that ezetimibe and the combination of ezetimibe and simvastatin reduce the cholesterol content of lipid subfractions, but have little effect on subfraction distribution, aside from a pronounced reduction of small, dense LDL particles in patients with primary dyslipidemias and elevated TG. As mentioned previously, the mechanism by which ezetimibe reduces levels of atherogenic lipoproteins is attributed to its reduction of the intestinal delivery of cholesterol to the liver, leading to increased LDL receptor activity, which correlates with diminished VLDL synthesis and enhanced clearance and catabolism of TRL Citation[35,39]. Correspondingly, if the amount of cholesterol in chylomicrons is decreased, then the amount of cholesterol in the respective remnants of chylomicrons following ezetimibe treatment may also be reduced. Ezetimibe, combined with statin inhibition of HMG CoA reductase, which also results in diminished VLDL production, and increased catabolism and clearance of TRL, can lead to an overall enhanced reduction of atherogenic lipoproteins and RLP.
Ezetimibe: phytosterol effects
Phytosterols and phytostanols are minor components of vegetable oils, cereals, fruits, vegetables, seeds and nuts that are effectively absorbed into the enterocyte, and then very efficiently pumped back into the intestinal lumen. Thus, dietary phytosterols and stanols undergo minimal systemic absorption from the GI tract; however, significant variability in intestinal absorption of phytosterols can occur based on genetic background and other factors. Elevated blood levels of plant sterols may increase CHD risk, although the evidence regarding this association is mixed Citation[120–127]. In particular, the excessive intestinal systemic absorption and impaired biliary removal that occurs in hypersitosterolemia patients promotes abnormally high blood levels of these phytosterols and a tendency to develop CHD as early as the teen years Citation[21]. The net hyperabsorption of both plant sterols and cholesterol in sitosterolemia patients usually (but not always) leads to hypercholesterolemia.
In these patients, high blood sterol levels cause characteristic development of tendon xanthomas (cholesterol deposits in skin and tendons), and red blood cell abnormalities that lead to chronic anemia. Sitosterolemia patients have normal or only moderately elevated levels of total sterols, but have a very high ratio of plant sterol to total cholesterol. Historically, sitosterolemia has been treated by strict limitation of dietary cholesterol and plant sterols and with cholestyramine resin administration to promote sterol excretion from the body. Due to its proven efficacy to reduce plant sterol intestinal absorption, ezetimibe is the only lipid-altering agent approved as adjunctive therapy for the treatment of this disorder Citation[53].
A variety of techniques can be used to estimate cholesterol absorption and synthesis, including measurement of mass absorption of cholesterol, plasma isotope ratio, and circulating levels of phytosterols (e.g., sitosterol and campesterol) and cholesterol synthesis precursors (e.g., desmosterol and lathosterol) Citation[8,43]. In addition to substantially inhibiting fractional cholesterol absorption, ezetimibe monotherapy produces significant, progressive reductions in plasma concentrations of phytosterols in patients with hypersitosterolemia, consistent with the hypothesis that ezetimibe inhibits intestinal absorption of both plant sterols and cholesterol Citation[21]. In patients with hypercholesterolemia, ezetimibe reduced circulating levels of campesterol by -48% and sitosterol by -41%, compared with placebo (p < 0.001 for both) Citation[128], again supporting a marked reduction in plant sterol absorption. In both studies, the reduction of cholesterol absorption by ezetimibe was accompanied by an increased ratio of lathosterol to cholesterol, suggesting enhanced hepatic cholesterol synthesis Citation[43].
In a study that compared the effect of ezetimibe, statins, and ezetimibe/statins on noncholesterol sterols, ezetimibe monotherapy significantly lowered plasma concentrations of sitosterol and campesterol from baseline compared with placebo (p < 0.001) Citation[129]. By contrast, these phytosterols were modestly increased by atorvastatin and mildly decreased by simvastatin . These changes were significantly different compared with the decreases in phytosterols induced by ezetimibe alone (p < 0.05 to p < 0.001). As anticipated, statins also reduced cholesterol synthesis precursors, lathosterol and desmosterol , whereas ezetimibe increased the levels of these cholesterol precursors, suggesting an enhancement of cholesterol synthesis by ezetimibe. However, combination therapy with ezetimibe and simvastatin or atorvastatin reduced both the concentrations of phytosterols (campesterol and sitosterol) and cholesterol precursors (lathosterol and desmosterol) . These data reflect a complementary benefit of simultaneous inhibition of both sterol absorption and synthesis.
Ezetimibe: oxysterol effects
Oxysterols include oxygenated derivatives of cholesterol and plant sterols. Enzymatic and nonenzymatic oxygenation of cholesterol results in a number of circulating and intracellular derivatives, which may have a variety of biologic activities, such as roles in sphingolipid metabolism, platelet aggregation, apoptosis and protein prenylation Citation[130]. Cholesterol is also converted to a variety of oxysterols as a precursor to bile acid synthesis. Due to processing, heating or prolonged storage of animal-derived foods, the typical Western diet contains substantial quantities of oxidized cholesterol Citation[131,132]. Studies in feeding animal models and epidemiological studies in humans have indicated that the addition of oxidized cholesterol to the diet increases the development of atherosclerosis Citation[132]. In addition, plant sterols can also be nonenzymatically auto-oxidized, especially during food storage.
Oxysterols are absorbed by the small intestine and incorporated into chylomicrons and LDL-C. Identified in the arterial wall, oxysterols may contribute to increased levels of oxidized lipoproteins in the intima Citation[132]. Ezetimibe (10 mg) reduces the serum levels of oxysterols, and the incorporation of oxidized cholesterol into postprandial chylomicrons and LDL particles Citation[133]. The absence of changes in postprandial TGs indicated that the decrease in oxysterol levels was attributed to the inhibition of oxidized sterol absorption rather than enhanced clearance.
Conclusion & expert commentary
Ezetimibe 10 mg/day significantly reduces LDL-C from baseline levels compared with placebo, and when added to statin therapy, provides significant further reductions in LDL-C levels. Comparable results are obtained with the combination tablet, ezetimibe/simvastatin (Vytorin). The LDL-lowering effects of ezetimibe and ezetimibe/simvastatin have been consistently demonstrated in different populations including patients with metabolic syndrome and T2DM.
Existing data support the hypothesis that ezetimibe has complementary and additive therapeutic lipid effects when combined with statins in the treatment of patients with dyslipidemia. In addition to LDL-C lowering, ezetimibe significantly improves other aspects of the lipid profile. Ezetimibe monotherapy reduces levels of TG and ApoB and increases HDL-C in comparison with placebo. When added to statins, ezetimibe provides significantly greater improvements in TG, HDL-C, non-HDL-C, ApoB and hsCRP. Similar effects on these parameters are consistently observed in different patient populations, including those with metabolic syndrome and T2DM. Plasma levels of RLP-C and lipoprotein subfractions are also favorably altered. These effects (and others) may impact cholesterol and lipid metabolism, as well as help to stimulate peripheral cholesterol transport. Ezetimibe itself lowers plasma phytosterol levels. It has also been shown to reduce serum levels of oxysterols and the incorporation of oxidized oxysterols into postprandial chylomicrons and LDL particles.
The potential clinical benefits of ezetimibe’s LDL-C lowering, and its other effects, await further assessment in clinical trials. The Ezetimibe and Simvastatin in Hypercholesterolemia Enhances Atherosclerosis Regression (ENHANCE) trial was a surrogate outcome trial that assessed changes in intima media thickness (IMT) in a familial hypercholesterolemic patient population. In this unique study, treatment with ezetimibe/simvastatin (10/80 mg) for 2 years did not demonstrate significant differences in IMT when compared with simvastatin 80 mg alone. Citation[134,201]. However, ENHANCE was not a CHD outcomes study. Thus, the potential clinical benefits of ezetimibe, as assessed by traditional determinations of actual CHD events, and in a different patient population, await the results of specifically designed CHD outcomes studies .
From a safety perspective, ezetimibe administered as monotherapy or as combination therapy is generally well tolerated. Ezetimibe itself has an adverse event profile similar to that of placebo for up to 12 weeks of treatment Citation[47–51]. Similarly, coadministration of ezetimibe (10 mg) with statins (10–80 mg doses) results in no significant differences in the incidences of laboratory and clinical adverse events, including gastrointestinal, liver or muscle effects during 6–24 weeks of treatment Citation[53–61,64]. The safety profile of the combination tablet ezetimbe/simvastatin at doses up to 10/80 mg also was found to be well tolerated in clinical studies when compared with atorvastatin (20 to 80 mg) and rosuvastatin (10 to 40 mg) Citation[65–67]. In patients with metabolic syndrome and T2DM, safety profiles were also similar to those demonstrated in other patient populations Citation[68–70].
Having said this, while ezetimibe monotherapy does not appear to increase liver enzymes, pooled data suggest that the frequency of liver enzyme elevation may be slightly (∼1%) higher when ezetimibe is added to statins. Thus, the prescribing information recommends that when ZETIA® is coadministered with a statin, that liver enzyme tests should be performed at initiation of therapy and according to the recommendations of the statin Citation[53]. From a practical standpoint, an interpretation of this guidance could be that adding ezetimibe to a statin may be considered as being analogous to increasing the dose of a statin. In other words, if it is recommended that increasing a statin dose from 40 to 80 mg should be accompanied by subsequent liver enzyme monitoring, then it would seem to be prudent to do the same if ezetimibe is added to a statin. Finally, while it is true that muscle adverse experiences have not been reported in controlled clinical trials of ezetimibe, isolated case reports of myopathy have been described with ezetimibe. Thus, the prescribing information recommends that all patients starting therapy with ezetimibe should be advised of the risk of myopathy and told to report promptly any unexplained muscle pain, tenderness or weakness. If myopathy is diagnosed or suspected, ezetimibe and any statin and/or fibrate should be discontinued immediately.
Five-year view
Whether LDL-C lowering through dual inhibition of reduced cholesterol absorption and synthesis translates to enhanced clinical benefit for reducing CHD events awaits further assessment in longer term, outcome-based clinical trials Citation[135–137]. During the next several years, the results of these CHD outcome studies will help to better clarify the clinical importance of the metabolic effects of ezetimibe, with respect to LDL-C lowering and beyond.
Table 1. ABC transporters and disease.
Table 2. Ezetimibe monotherapy studies.
Table 3. Ezetimibe combination studies.
Table 4. Clinical outcome studies.
Key issues
• | Ezetimibe is an inhibitor of cholesterol absorption indicated to improve lipid levels in hypercholesterolemic patients. At a 10 mg dose, ezetimibe effectively reduces LDL-C levels compared with placebo. Ezetimibe also reduces levels of triglyceride and apolipoprotein (Apo)B and modestly increases HDL-C. | ||||
• | When combined with statins, ezetimibe’s complementary mechanism of action provides further improvements in the overall lipid profile. When ezetimibe is concomitantly administered with statins, LDL-C levels may be reduced up to approximately 60%. Ezetimibe added to statins significantly reduces TG, HDL-C, non-HDL-C, ApoB and high-sensitivity C-reactive protein compared with statins alone. Ezetimibe reduces RLP-cholesterol levels and, in hypertriglyceridemic patients, may improve lipoprotein subfractions. These effects (and others) may impact cholesterol and lipid metabolism, as well as help to stimulate peripheral cholesterol transport. | ||||
• | Ezetimibe administered as monotherapy or as combination therapy is generally well tolerated. Ezetimibe administration results in no significant increases in clinical and laboratory adverse experiences, including gastrointestinal, hepatic and muscle effects. | ||||
• | Assessment of the clinical benefit of ezetimibe in reducing cardiovascular disease in patients awaits further evaluation in coronary heart disease outcome-based clinical trials. Several clinical trials are underway to evaluate the effect of ezetimibe coadministered with simvastatin on cardiovascular events and outcomes. |
Financial & competing interests disclosure
Harold Bays has served as a Clinical Investigator for (and has received research grants from) pharmaceutical companies such as Abbott, Amylin, Alteon, Arena, AstraZeneca, Aventis, Bayer, Boehringer Ingelheim, Boehringer Mannheim, Bristol Myers Squibb, Ciba Geigy, Daiichi Sankyo, Eli Lilly, Esperion, Fujisawa, GelTex, Genetech, GlaxoSmithKline, Hoechst Roussel, Hoffman LaRoche, InterMune, KOS, Kowa, Lederle, Marion Merrell Dow, Merck, Merck Schering Plough, Miles, Microbia, Novartis, Obecure, Orexigen, Parke Davis, Pfizer, Pliva, Purdue, Reliant, Roche, Rorer, Regeneron, Sandoz, Sanofi, Sciele, Searle, Shionogi, Schering Plough, SmithKline Beacham, Takeda, TAP, UpJohn, Upsher Smith, Warner Lambert, and Wyeth-Ayerst. He has also served as a consultant, speaker and/or advisor to and for pharmaceutical companies such as Abbott, Arena, AstraZeneca, Aventis, Bayer, Bristol Myers Squibb, Daiichi Sankyo, KOS, Merck, Merck Schering Plough, Metabasis Therapeutics, Microbia, Novartis, Nicox, Ortho-McNeil, Parke Davis, Pfizer, Reliant, Roche, Sandoz, Sanofi Aventis, Schering Plough, SmithKline Beacham, Takeda, UpJohn, and Warner Lambert. Drs Neff, Tershakovec and Tomassini are employees of Merck and own stock and stock options.
No writing assistance was utilized in the production of this manuscript.
References
- Grundy SM, Cleeman JI, Merz CN et al. Implications of recent clinical trials for the national cholesterol education program adult treatment panel III guidelines. Circulation110(2), 227–239 (2004).
- Bays H, McKenney J, Davidson M. Torcetrapib/atorvastatin combination therapy. Expert Rev. Cardiovasc. Ther.3(5), 789–820 (2005).
- Heart Protection Study Collaborative Group. MRC/BHF Heart Protection Study of cholesterol lowering with simvastatin in 20,536 high-risk individuals: a randomised placebo-controlled trial. Lancet360(9326), 7–22 (2002).
- Robinson JG, Smith B, Maheshwari, N, Schrott H. Pleiotropic effects of statins beyond cholesterol reduction? J. Am. Coll. Cardiol.46(10), 1855–1862 (2005).
- Bays H. Ezetimibe. Expert Opin. Investig. Drugs11(11), 1587–1604 (2002).
- Robinson JG, Davidson MH. Combination therapy with ezetimibe and simvastatin to achieve aggressive LDL reduction. Expert Rev. Cardiovasc. Ther.4(4), 461–476 (2006).
- Burnett JR, Huff MW. Cholesterol absorption inhibitors as a therapeutic option for hypercholesterolaemia. Expert Opin. Investig. Drugs15(11), 1337–1351 (2006).
- Santosa S, Varady KA, AbuMweis S, Jones PJ. Physiological and therapeutic factors affecting cholesterol metabolism: does a reciprocal relationship between cholesterol absorption and synthesis really exist? Life Sci.80(6), 505–514 (2007).
- Turley SD, Dietschy JM. Sterol absorption by the small intestine. Curr. Opin. Lipidol.14(3), 233–240 (2003).
- Bays H, Dujovne C. Colesevelam HCl: a non-systemic lipid-altering drug. Expert Opin. Pharmacother.4(5), 779–790 (2003).
- Mayes PA. Cholesterol synthesis, transport and excretion. In: Harper’s Biochemistry (25th Edition). Murray RK, Granner DK, Mayes PA, Rodwell VW (Eds). Appleton & Lange, CT, USA, 285–305 (2000).
- Dietschy JM, Turley SD, Spady DK. Role of liver in the maintenance of cholesterol and low density lipoprotein homeostasis in different animal species, including humans. J. Lipid. Res.34(10), 1637–1659 (1993).
- Lewis GF, Rader DJ. New insights into the regulation of HDL metabolism and reverse cholesterol transport. Circ. Res.96(12), 1221–1232 (2005).
- Ikonen E. Mechanisms for cellular cholesterol transport: defects and human disease. Physiol. Rev.86(4), 1237–1261 (2006).
- Gylling H. Cholesterol metabolism and its implications for therapeutic interventions in patients with hypercholesterolaemia. Int. J. Clin. Pract.58(9), 859–866 (2004).
- Kruit JK, Groen AK, van Berkel TJ, Kuipers F. Emerging roles of the intestine in control of cholesterol metabolism. World J. Gastroenterol.12(40), 6429–6439 (2006).
- Lee MH, Lu K, Hazard S et al. Identification of a gene, ABCG5, important in the regulation of dietary cholesterol absorption. Nat. Genet.27(1), 79–83 (2001).
- Wang, Q-H. Regulation of intestinal cholesterol absorption. Annu. Rev. Physiol.69, 221–48 (2007).
- Berge KE, Tian H, Graf GA et al. Accumulation of dietary cholesterol in sitosterolemia caused by mutations in adjacent ABC transporters. Science290(5497), 1771–1775 (2000).
- Stanek EJ, Sarawate C, Willey VJ, Charland SL, Cziraky MJ. Risk of cardiovascular events in patients at optimal values for combined lipid parameters. Curr. Med. Res. Opin.23(3), 553–563 (2007).
- Salen G, von Bergmann K, Lutjohann D et al. Ezetimibe effectively reduces plasma plant sterols in patients with sitosterolemia. Circulation109(8), 966–971 (2004).
- Yu LQ, Li-Hawkins J, Hammer RE et al. Overexpression of ABCG5 and ABCG8 promotes biliary cholesterol secretion and reduces fractional absorption of dietary cholesterol. J. Clin. Invest.110(5), 671–680 (2002).
- Kruit JK, Plosch T, Havinga R et al. Increased fecal neutral sterol loss upon liver X receptor activation is independent of biliary sterol secretion in mice. Gastroenterology128(1), 147–156 (2005).
- Brunham LR, Kruit JK, Iqbal J et al. Intestinal ABCA1 directly contributes to HDL biogenesis in vivo. J. Clin. Invest.116(4), 1052–1062 (2006).
- Wu AL, Windmueller HG. Relative contributions by liver and intestine to individual plasma apolipoproteins in the rat. J. Biol. Chem.254(45), 7316–7322 (1979).
- Davis HR, Zhu LJ, Hoos LM et al. Niemann–Pick C1 like 1 (NPC1L1) is the intestinal phytosterol and cholesterol transporter and a key modulator of whole-body cholesterol homeostasis. J. Biol. Chem.279(32), 33586–33592 (2004).
- Garcia-Calvo M, Lisnock JM, Bull HG et al. The target of ezetimibe is Niemann-Pick Cl-Like 1 (NPC1L1). Proc. Natl. Acad. Sci. USA102(23), 8132–8137 (2005).
- Lammert F, Wang DQ. New insights into the genetic regulation of intestinal cholesterol absorption. Gastroenterology129(2), 718–734 (2005).
- Catapano AL. The pharmacologic elegance of inhibiting cholesterol absorption and synthesis while providing a homeostatic balance. Fundam. Clin. Pharmacol.21 (2), 21–26, (2007).
- Ternel RE, Tang W, Ma Y et al. Hepatic Niemann–Pick C1-like 1 regulate biliary concentration and is a target of ezetimibe. J. Clin Invest.117 (7), 1968–1978 (2007).
- van Heek M, Compton DS, Davis HR. The cholesterol absorption inhibitor, ezetimibe, decreases diet-induced hypercholesterolemia in monkeys. Eur. J. Pharmacol.415(1), 79–84 (2001).
- Bays H, Stein EA. Pharmacotherapy for dyslipidaemia – current therapies and future agents. Expert Opin. Pharmacother.4(11), 1901–1938 (2003).
- Davis HR, Compton DS, Hoos LM, Tetzloff GG. Ezetimibe reduces plasma cholesterol and inhibits the development of atherosclerosis in apo E knockout mice with and without LDL receptors. Circulation102(18), 186 (2000).
- Repa JJ, Turley SD, Quan G, Dietschy JM. Delineation of molecular changes in intrahepatic cholesterol metabolism resulting from diminished cholesterol absorption. J. Lipid. Res.46(4), 779–789 (2005).
- Tremblay AJ, Lamarche B, Cohn JS, Hogue JC, Couture P. Effect of ezetimibe on the in vivo kinetics of apoB-48 and apoB-100 in men with primary hypercholesterolemia. Arterioscler. Thromb. Vasc. Biol.26(5), 1101–1106 (2006).
- Hachem SB, Mooradian AD. Familial dyslipidaemias: an overview of genetics, pathophysiology and management. Drugs66(15), 1949–1969 (2006).
- Gagne C, Gaudet D, Bruckert E. Efficacy and safety of ezetimibe coadministered with atorvastatin or simvastatin in patients with homozygous familial hypercholesterolemia. Circulation105(21), 2469–2475 (2002).
- Baigent C, Keech A, Kearny PM et al.; Cholesterol Treatment Trialists’ (CTT) Collaborators. Efficacy and safety of cholesterol-lowering treatment: prospective meta-analysis of data from 90,056 participants in 14 randomised trials of statins. Lancet366(9493), 1267–1278 (2005).
- Lamon-Fava S, Diffenderfer MR, Barrett PH et al. Effects of different doses of atorvastatin on human apolipoprotein B-100, B-48, and A-I metabolism. J. Lipid Res.48(8), 1746–1753 (2007).
- Holdgate GA, Ward WHJ, McTaggart F. Molecular mechanism for inhibition of 3-hydroxy-3-methylglutaryl CoA (HMG-CoA) reductase by rosuvastatin. Biochem. Soc. Trans.31(Pt 3), 528–531 (2003).
- Toth PP, Davidson MH. Cholesterol absorption blockade with ezetimibe. Curr. Drug Targets Cardiovasc. Haematol. Disord.5(6), 455–462 (2005).
- Miettinen TA, Gylling H. Ineffective decrease of serum cholesterol by simvastatin in a subgroup of hypercholesterolemic coronary patients. Atherosclerosis164(1), 147–152 (2002).
- Miettinen TA, Gylling H. Synthesis and absorption markers of cholesterol in serum and lipoproteins during a large dose of statin treatment. Eur. J. Clin. Invest.33(11), 976–982 (2003).
- Altmann SW, Davis HR, Zhu LJ et al. Niemann-Pick C1 like 1 protein is critical for intestinal cholesterol absorption. Science303(5661), 1201–1204 (2004).
- Vasudevan AR, Jones PH. Effective use of combination lipid therapy. Curr. Atheroscler. Rep.8(1), 76–84 (2006).
- Telford DE, Sutherland BG, Edwards JY, Andrews JD, Barrett PH, Huff MW. The molecular mechanisms underlying the reduction of LDL apoB-100 by ezetimibe plus simvastatin. J. Lipid. Res.48(3), 699–708 (2007).
- Sudhop T, Lutjohann D, Kodal A et al. Inhibition of intestinal cholesterol absorption by ezetimibe in humans. Circulation106(15), 1943–1948 (2002).
- Bays HE, Moore PB, Drehobl MA et al. Effectiveness and tolerability of ezetimibe in patients with primary hypercholesterolemia: pooled analysis of two Phase II studies. Clin. Ther.23(8), 1209–1230 (2001).
- Dujovne CA, Ettinger MP, McNeer JF et al. Efficacy and safety of a potent new selective cholesterol absorption inhibitor, ezetimibe, in patients with primary hypercholesterolemia. Am. J. Cardiol.90(10), 1092–1097 (2002).
- Knopp RH, Gitter H, Truitt T et al. Effects of ezetimibe, a new cholesterol absorption inhibitor, on plasma lipids in patients with primary hypercholesterolemia. Eur. Heart J.24(8), 729–741 (2003).
- Stein E. Results of phase I/II clinical trials with ezetimibe, a novel selective cholesterol absorption inhibitor. Eur. Heart J.3(Suppl. E), E11–E16 (2001).
- Mandema JW, Hermann D, Wang W et al. Model-based development of gemcabene, a new lipid-altering agent. AAPS J.7(3), E513–E522 (2005).
- Zetia. Product insert. 2001.
- Gagne C, Bays HE, Weiss SR et al. Efficacy and safety of ezetimibe added to ongoing statin therapy for treatment of patients with primary hypercholesterolemia. Am. J. Cardiol.90(10), 1084–1091 (2002).
- Pearson TA, Denke MA, McBride PE, Battisti WP, Brady WE, Palmisano J. A community-based, randomized trial of ezetimibe added to statin therapy to attain NCEP ATP III goals for LDL cholesterol in hypercholesterolemic patients: the ezetimibe add-on to statin for effectiveness (EASE) trial. Mayo Clin. Proc.80(5), 587–595 (2005).
- Masana L, Mata P, Gagne C et al. Long-term safety and, tolerability profiles and lipid-modifying efficacy of ezetimibe coadministered with ongoing simvastatin treatment: a multicenter, randomized, double-blind, placebo-controlled, 48-week extension study. Clin. Ther.27(2), 174–184 (2005).
- Davidson MH, Ballantyne CM, Kerzner B et al. Efficacy and safety of ezetimibe coadministered with statins: randomised, placebo-controlled, blinded experience in 2382 patients with primary hypercholesterolemia. Int. J. Clin. Pract.58(8), 746–755 (2004).
- Davidson MH, McGarry T, Bettis R et al. Ezetimibe coadministered with simvastatin in patients with primary hypercholesterolemia. J. Am. Coll. Cardiol.40(12), 2125–2134 (2002).
- Goldberg AC, Sapre A, Liu J, Capece R, Mitchel YB. Efficacy and safety of ezetimibe coadministered with simvastatin in patients with primary hypercholesterolemia: a randomized, double-blind, placebo-controlled trial. Mayo Clin. Proc.79(5), 620–629 (2004).
- Feldman T, Koren M, Insull W Jr et al. Treatment of high-risk patients with ezetimibe plus simvastatin co-administration versus simvastatin alone to attain National Cholesterol Education Program Adult Treatment Panel III low-density lipoprotein cholesterol goals. Am. J. Cardiol.93(12), 1481–1486 (2004).
- Ballantyne CM, Blazing MA, King TR, Brady WE, Palmisano J. Efficacy and safety of ezetimibe co-administered with simvastatin compared with atorvastatin in adults with hypercholesterolemia. Am. J. Cardiol.93(12), 1487–1494 (2004).
- Sager PT, Melani L, Lipka L et al.for the Ezetimibe Study Group. Effect of coadministration of ezetimibe and simvastatin on high-sensitivity C-reactive protein. Am. J. Cardiol.92(12), 1414–1418 (2003).
- Sager PT, Capece R, Lipka L et al. Effects of ezetimibe coadministered with simvastatin on C-reactive protein in a large cohort of hypercholesterolemic patients. Atherosclerosis179(2), 361–367 (2005).
- Ballantyne CM, Houri J, Notarbartolo A et al. Effect of ezetimibe coadministered with atorvastatin in 628 patients with primary hypercholesterolemia: a prospective, randomized, double-blind trial. Circulation107(19), 2409–2415 (2003).
- Bays HE, Ose L, Fraser N et al. A multicenter, randomized, double-blind, placebo-controlled, factorial design study to evaluate the lipid-altering efficacy and safety profile of the ezetimibe/simvastatin tablet compared with ezetimibe and simvastatin monotherapy in patients with primary hypercholesterolemia. Clin. Ther.26(11), 1758–1773 (2004).
- Ballantyne CM, Abate N, Yuan Z, King TR, Palmisano J. Dose-comparison study of the combination of ezetimibe and simvastatin (Vytorin) versus atorvastatin in patients with hypercholesterolemia: the Vytorin Versus Atorvastatin (VYVA) study. Am. Heart J.149(3), 464–473 (2005).
- Catapano AL, Davidson MH, Ballantyne CM et al. Lipid-altering efficacy of the ezetimibe/simvastatin single tablet versus rosuvastatin in hypercholesterolemic patients. Curr. Med. Res. Opin.22(10), 2041–2053 (2006).
- Simons L, Tonkon M, Masana L et al. Effects of ezetimibe added to on-going statin therapy on the lipid profile of hypercholesterolemic patients with diabetes mellitus or metabolic syndrome. Curr. Med. Res. Opin.20(9), 1437–1445 (2004).
- Denke M, Pearson T, McBride P, Gazzara RA, Brady WE, Tershakovec AM. Ezetimibe added to ongoing statin therapy improves LDL-C goal attainment and lipid profile in patients with diabetes or metabolic syndrome. Diab. Vasc. Dis. Res.3(2), 93–102 (2006).
- Goldberg RB, Guyton JR, Mazzone T et al. Ezetimibe/Simvastatin vs atorvastatin in patients with Type 2 diabetes mellitus and hypercholesterolemia: The VYTAL study. Mayo Clin. Proc.81(12), 1579–1588 (2006).
- Smith SC, Jr, Allen J, Blair SN et al. AHA/ACC guidelines for secondary prevention for patients with coronary and other atherosclerotic vascular disease: 2006 update: endorsed by the National Heart, Lung, and Blood Institute. Circulation113(19), 2363–2372 (2006).
- Grundy SM. Low-density lipoprotein, non-high-density lipoprotein, and apolipoprotein B as targets of lipid-lowering therapy. Circulation106(20), 2526–2529 (2002).
- Chapman MJ and Caslake M. Non-high-density lipoprotein cholesterol as a risk factor: addressing risk associated with apolipoprotein B-containing lipoproteins. Eur. Heart J. Supplements6, A43-A48 (2004).
- National Cholesterol Education Program (NCEP) Expert Panel on Detection, Evaluation, and Treatment of High Blood Cholesterol in Adults (Adult Treatment Panel III) final report. Circulation106(25), 3143–3421 (2002).
- Robinson JG, Davidson MH, Shah A et al. Efficacy and safety of ezetimibe and ezetimibe plus statin therapy in patients aged under 65, 65–74 and 75 years and older Aging Health3(6) 691–705 (2007).
- American Diabetes Association. Standards of medical care in diabetes – 2006. Diabetes Care29(Suppl. 1), S4–S42 (2006).
- Grundy SM, Cleeman JI, Daniels SR et al. Diagnosis and Management of the Metabolic Syndrome: An American Heart Association/National Heart, Lung, and Blood Institute Scientific Statement Executive Summary. Circulation112(17), e285–e290 (2005).
- Guerin M, Lassel TS, Le Goff W, Farnier M, Chapman MJ. Action of atorvastatin in combined hyperlipidemia – preferential reduction of cholesteryl ester transfer from HDL to VLDL1 particles. Arterioscler. Thromb. Vasc. Bio.20(1), 189–197 (2000).
- Schaefer EJ, Asztalos BF. The effects of statins on high-density lipoproteins. Curr. Atheroscler. Rep.8(1), 41–49 (2006).
- Timmins JM, Lee JY, Boudyguina E et al. Targeted inactivation of hepatic Abca1 causes profound hypoalphalipoproteinemia and kidney hypercatabolism of apoA-I. J. Clin. Invest.115(5), 1333–1342 (2005).
- Chung BH, Liang P, Doran S, Cho BH, Franklin F. Postprandial chylomicrons: potent vehicles for transporting cholesterol from endogenous LDL+HDL and cell membranes to the liver via LCAT and CETP. J. Lipid Res.45(7), 1242–1255 (2004).
- Taggart W, Lutjohann D, Musliner T, Sudhop T, Shah A, von Bergmann K. Inhibition of intestinal cholesterol absorption and endogenous cholesterol production by ezetimibe/simvastatin in man. J. Clin. Lipid.1(5), 437 (2007).
- Briand F, Millar J, Billheimer J, Rothblat G, Rader D. Inhibition of cholesterol absorption with ezetimibe promotes macrophage to feces reverse cholesterol transport in mice. Circulation116, II159– II160 (2007).
- Pedersen TR, Faergeman O, Kastelein JJP et al. for the Incremental Decrease in End Points Through Aggressive Lipid Lowering (IDEAL) Study Group High-Dose Atorvastatin vs Usual-Dose Simvastatin for Secondary Prevention After Myocardial Infarction. The IDEAL Study: a randomized controlled trial. JAMA294, 2437–2445 (2005).
- Waters DD, Guyton JR, Herrington DM, McGowan MP, Wenger NK, Shear C; TNT Steering Committee Members and Investigators Treating to New Targets (TNT) Study. Does lowering low-density lipoprotein cholesterol levels below currently recommended guidelines yield incremental clinical benefit? Am. J. Cardiol.93, 154–158 (2004).
- Barter PJ, Ballantyne CM, Carmena R et al. Apo B versus cholesterol in estimating cardiovascular risk and in guiding therapy: report of the thirty-person/ten-country panel. J. Intern. Med.259(3), 247–258 (2006).
- Sniderman AD. Apolipoprotein B versus non-high-density lipoprotein cholesterol: and the winner is… Circulation112(22), 3366–3367 (2005).
- Stein EA, Sniderman A, Laskarzewski P. Assessment of reaching goal in patients with combined hyperlipidemia: low-density lipoprotein cholesterol, non-high-density lipoprotein cholesterol, or apolipoprotein B. Am. J. Cardiol.96(9A), 36K–43K (2005).
- Pischon T, Girman CJ, Sacks FM, Rifai N, Stampfer MJ, Rimm EB. Non-high-density lipoprotein cholesterol and apolipoprotein B in the prediction of coronary heart disease in men. Circulation112(22), 3375–3383 (2005).
- Genest J, Frohlich J, Fodor G, McPherson R. Recommendations for the management of dyslipidemia and the prevention of cardiovascular disease: summary of the 2003 update. CMAJ169(9), 921–924 (2003).
- Denke MA. Weighing in before the fight: low-density lipoprotein cholesterol and non-high-density lipoprotein cholesterol versus apolipoprotein B as the best predictor for coronary heart disease and the best measure of therapy. Circulation112(22), 3368–3370 (2005).
- Basso F, Freeman LA, Ko C et al. Hepatic ABCG5/G8 overexpression reduces apoB-lipoproteins and atherosclerosis when cholesterol absorption is inhibited. J. Lipid Res.48(1), 114–126 (2007).
- Ridker PM, Rifai N, Rose L, Buring JE, Cook NR. Comparison of C-reactive protein and low-density lipoprotein cholesterol levels in the prediction of first cardiovascular events. N. Engl. J. Med.347(20), 1557–1565 (2002).
- Pearson TA, Mensah GA, Alexander RW et al. Markers of inflammation and cardiovascular disease: application to clinical and public health practice: a statement for healthcare professionals from the Centers for Disease Control and Prevention and the American Heart Association. Circulation107(3), 499–511 (2003).
- Nissen SE, Tuzcu EM, Schoenhagen P et al. Statin therapy, LDL cholesterol, C-reactive protein, and coronary artery disease. N. Engl. J. Med.352(1), 29–38 (2005).
- Ridker PM, Cannon CP, Morrow D et al. C-reactive protein levels and outcomes after statin therapy. N. Engl. J. Med.352(1), 20–28 (2005).
- Pearson T, Ballantyne CM, Sisk C, Shah A, Veltri ER, Maccubbin D. Comparison of effects of ezetimibe/simvastatin versus simvastatin versus atorvastatin in reducing C-reactive protein and low-density lipoprotein cholesterol levels. Am. J. Cardiol.99(12), 1706–1713 (2007).
- Pearson T, Ballantyne CM, Veltri ER et al. CRP and D LDL-C response to ezetimibe: monotherapy and add-on to baseline statin. Triennial international symposium on drugs affecting lipid metabolism. J. Clin. Lipidol.1(5), 466 (2007).
- Malik S, Wong ND, Franklin S, Pio J, Fairchild C, Chen R. Cardiovascular disease in US patients with metabolic syndrome, diabetes, and elevated C-reactive protein. Diabetes Care28(3), 690–693 (2005).
- Ray KK, Cannon CP, Cairns R et al. Relationship between uncontrolled risk factors and C-reactive protein levels in patients receiving standard or intensive statin therapy for acute coronary syndromes in the PROVE IT-TIMI 22 Trial. J. Am. Coll Cardiol.46(8), 1417–1424 (2005).
- Kinlay S. Low-density lipoprotein-dependent and -independent effects of cholesterol-lowering therapies on C-reactive protein – a meta-analysis. J. Am. Coll. Cardiol.49(20), 2003–2009 (2007).
- Arnaud C, Burger F, Steffens S et al. Statins reduce interleukin-6-induced C-reactive protein in human hepatocytes – new evidence for direct antiinflammatory effects of statins. Arterioscler. Thromb. Vasc. Bio.25(6), 1231–1236 (2005).
- Seedorf U, Engel T, Lueken A, Bode G, Lorkowski S, Assmann G. Cholesterol absorption inhibitor Ezetimibe blocks uptake of oxidized LDL in human macrophages. Biochem.Biophys. Res. Comm.320(4), 1337–1341 (2004).
- Orso E, Werner T, Wolf Z, Bandulik S, Kramer W, Schmitz G. Ezetimibe influences the expression of raft-associated antigens in human monocytes. Cytometry A69(3), 206–208 (2006).
- McNamara JR, Shah PK, Nakajima K et al. Remnant-like particle (RLP) cholesterol is an independent cardiovascular disease risk factor in women: results from the Framingham Heart Study. Atherosclerosis154(1), 229–236 (2001).
- Karpe F, Boquist S, Tang R, Bond GM, de Faire U, Hamsten A. Remnant lipoproteins are related to intima-media thickness of the carotid artery independently of LDL cholesterol and plasma triglycerides. J. Lipid Res.42(1), 17–21 (2001).
- Imke C, Rodriguez BL, Grove JS et al. Are remnant-like particles independent predictors of coronary heart disease incidence? The Honolulu Heart study. Arterioscler. Thromb. Vasc. Biol.25(8), 1718–1722 (2005).
- Twicker TB, Dallinga-Thie GM, Cohn JS, Chapman MJ. Elevated remnant-like particle cholesterol concentration – a characteristic feature of the atherogenic lipoprotein phenotype. Circulation109(16), 1918–1925 (2004).
- Cohn JS, Marcoux C, Davignon J. Detection, quantification, and characterization of potentially atherogenic triglyceride-rich remnant lipoproteins. Arterioscler. Thromb. Vasc. Biol.19(10), 2474–2486 (1999).
- Harchaoui KE,van der steeg WA, Stores ES. Value of Low-density lipoprotein particle number and size as predictor of coronary artery disease in apparently healthy men and women. The EPIC-Norfolk Prospective population study. J. Am. Coll. Cardiol.49, 547–553 (2007).
- Packard CJ, Ford I, Robertson M et al. Plasma lipoproteins and apolipoproteins as predictors of cardiovascular risk and treatment benefit in the PROspective Study of Pravastatin in the Elderly at Risk (PROSPER). Circulation112(20), 3058–3065 (2005).
- St Pierre AC, Cantin B, Dagenais GR et al. Low-density lipoprotein subfractions and the long-term risk of ischemic heart disease in men: 13-year follow-up data from the Quebec Cardiovascular Study. Arterioscler. Thromb. Vasc. Biol.25(3), 553–559 (2005).
- Rosenson RS, Otvos JD, Freedman DS. Relations of lipoprotein subclass levels and low-density lipoprotein size to progression of coronary artery disease in the Pravastatin Limitation of Atherosclerosis in the Coronary Arteries (PLAC-I) trial. Am. J. Cardiol.90(2), 89–94 (2002).
- Austin MA, King MC, Vranizan KM, Krauss RM. Atherogenic lipoprotein phenotype. A proposed genetic marker for coronary heart disease risk. Circulation82(2), 495–506 (1990).
- Farnier M, Freeman MW, Macdonell G et al. Efficacy and safety of the coadministration of ezetimibe with fenofibrate in patients with mixed hyperlipidaemia. Eur. Heart J.26(9), 897–905 (2005).
- Tribble D, Farnier M, Freeman MW et al. Effect of fenofibrate and ezetimibe on lipoprotein subclasses and LDL subclass pattern in patients with mixed hyperlipidemia. Circulation110(17, Suppl.), III143–III144 (2004).
- Ose L, Olegario KQ, Reyes R, Johnson-Levonas AO, Sapre A, Tribble D. Effects of ezetimibe/simvastatin on lipoprotein subclasses in patients with primary hypercholesterolemia: an exploratory analysis of archived samples using two commercially available techniques. Clin. Ther.29(11), 2419–2432 (2007).
- Kalogirou M, Tsimihodimos V, Gazi I et al. Effect of ezetimibe monotherapy on the concentration of lipoprotein subfractions in patients with primary dyslipidaemia. Curr. Med. Res. Opin.23(5), 1169–1176 (2007).
- Kolovou GD, Anagnostopoulou KK, Salpea KD, Daskalopoulou SS, Mikhailidis DP. The effect of statins on postprandial lipemia. Curr. Drug Targets8(4), 551–560 (2007).
- Chan YM, Varady KA, Lin Y et al. Plasma concentrations of plant sterols: physiology and relationship with coronary heart disease. Nutr. Rev.64(9), 385–402 (2006).
- Normen L, Shaw CA, Fink CS, Awad AB. Combination of phytosterols and omega-3 fatty acids: a potential strategy to promote cardiovascular health. Curr. Med. Chem. Cardiovasc. Hematol. Agents2(1), 1–12 (2004).
- Matthan N, Larocque JM, Pencina M et al. Increased cholesterol absorption and decreased cholesterol synthesis characterize Framingham Offspring Study participants with coronary heart disease. Circulation112(17 Suppl. S), U892–U893 (2005).
- Fassbender K, Lutjohann D, Dik MG et al. Moderately elevated plant sterol levels are associated with reduced cardiovascular risk-The LASA study. Atherosclerosis (doi:10.1016/j.atherosclerosis.2006.10. 032) (2006).
- Pinedo S, Vissers MN, von Bergmann K et al. Plasma levels of plant sterols and the risk of coronary artery disease: the prospective EPIC-Norfolk Population Study. J. Lipid Res.48(1), 139–144 (2007).
- Thiery J, Ceglarek U, Fiedler G et al. Elevated campesterol serum levels – a significant predictor of incident myocardial infarction: Results of the population-based MONICA/KORA follow-up study 1994 to 2005. Circulation114(18 Suppl. S), 884 (2006).
- Wilund KR, Yu L, Xu F et al. No association between plasma levels of plant sterols and atherosclerosis in mice and men. Arterioscler. Thromb. Vasc. Biol.24(12), 2326–2332 (2004).
- John S, Sorokin AV, Thompson PD. Phytosterols and vascular diseases. Curr. Opin. Lipidol.18(1), 35–45 (2007).
- Sudhop T, Lutjohann D, Reber D et al. Inhibition of intestinal cholesterol absorption and endogenous cholesterol synthesis by ezetimibe/simvastatin in humans. J. Am. Coll. Cardiol45(3 Suppl A), 392A (2005).
- Assmann G, Kannenberg F, Musliner T, Ramey D, Veltri ER. Effects of ezetimibe, simvastatin, atorvastatin, and ezetimibe-statin therapies on non-cholesterol sterols in patients with primary hypercholesterolemia. Curr. Med. Res. Opin.24(1), 249–259 (2008).
- Schroepfer GJ Jr. Oxysterols: modulators of cholesterol metabolism and other processes. Physiol. Rev.80(1), 361–554 (2000).
- Staprans I, Pan XM, Rapp JH, Grunfeld C, Feingold KR. Oxidized cholesterol in the diet accelerates the development of atherosclerosis in LDL receptor- and apolipoprotein E-deficient mice. Arterioscler. Thromb. Vasc. Biol.20(3), 708–714 (2000).
- Staprans I, Pan XM, Rapp JH, Feingold KR. Oxidized cholesterol in the diet is a source of oxidized lipoproteins in human serum. J. Lipid Res.44(4), 705–715 (2003).
- Staprans I, Pan XM, Rapp JH, Moser AH, Feingold KR. Ezetimibe inhibits the incorporation of dietary oxidized cholesterol into lipoproteins. J. Lipid Res.47(11), 2575–2580 (2006).
- Kastelein JJ, Sager PT, de Groot E, Veltri E. Comparison of ezetimibe plus simvastatin versus simvastatin monotherapy on atherosclerosis progression in familial hypercholesterolemia. Design and rationale of the Ezetimibe and Simvastatin in Hypercholesterolemia Enhances Atherosclerosis Regression (ENHANCE) trial. Am. Heart J.149(2), 234–239 (2005).
- Rossebo A, Pedersen T, Allen C et al. Design and baseline characteristics of the Simvastatin and Ezetimibe in Aortic Stenosis (SEAS) study. Am. J. Cardiol.99(7), 970–973 (2007).
- Baigent C, Landry M. Study of heart and renal protection. Kidney Int.63(S84), 207–210 (2003).
- Cannon CP, Guigliano RP, Blazing MA et al for the IMPROVE-IT investigators. Design and Rationale of IMPROVE-IT (IMProved Reduction of Outcomes: Vytorin Efficacy International Trial): Comparison of ezetimibe/simvastatin versus simvastatin monotherapy on cardiovascular outcomes in patients with acute coronary syndrome. Am. Heart J. (2007). [Epub ahead of print].
Website
- Merck/Schering-Plough Pharmaceuticals provides results of the ENHANCE Trial. www.merck.com/newsroom/press_releases/product/2008_0114.html