Abstract
The lack of vaccines against several important viral diseases necessitates the development of therapeutics to save lives and control epidemics. In recent years, therapeutic antibodies have received considerable attention due to their good safety profiles and clinical success when used against viruses such as respiratory syncytial virus, Ebola virus and Hendra virus. The binding affinity of these antibodies can directly impact their therapeutic efficacy. However, we and others have also demonstrated that the subtype of Fc-gamma receptors (FcγRs) engaged influences the stoichiometric requirement for virus neutralization. Hence, the development of therapeutic antibodies against infectious diseases should consider the FcγRs engaged and Fc-effector functions involved. This review highlights the current state of knowledge about FcγRs and FcγR effector functions involved in virus neutralization, with emphasis on factors that can affect FcγR engagement. A better understanding of Fc-FcγR interactions during virus neutralization will allow development of therapeutic antibodies that are efficacious and can be administered with minimal side effects.
While vaccination now protects against several important infectious diseases, many others still lack effective vaccines. The ability of viruses to rapidly mutate and alter their antigenic makeup (e.g., influenza and HIV), as well as the potential interference between different virus serotypes (e.g., dengue virus) has made vaccine development a challenging task. Given that infection with such viruses can result in a significant number of cases and high mortality rates, rapid development of potent therapeutic measures can potentially save lives and limit the magnitude of outbreaks. With technological advances in the field of immunoglobulin G (IgG) research and generally good safety profiles observed during antibody-based therapies, therapeutic antibodies have become attractive candidates for treating infectious diseases. The recent Ebola outbreak in West Africa is a good example of the potential of mAb cocktails as an anti-infective strategy Citation[1]. Other examples include the use of mAb m102.4 against Hendra virus Citation[2] and palivizumab that targets respiratory syncytial virus (RSV) Citation[3], resulting in significant reduction in the incidence of hospitalization. Given the need for new antiviral therapies and considerable advances in IgG research, there is a growing interest in developing therapeutic antibodies against infectious diseases.
The binding affinity of antibodies to viruses can directly impact the efficacy of mAbs Citation[4], suggesting that target-specific mechanisms likely account for much of the efficacy of therapeutic mAbs. However, many studies have also highlighted the contribution of Fc-mediated immune effector functions in modulating the efficacy of these mAbs Citation[5]. Antigen-presenting cells such as dendritic cells, monocytes and macrophages can internalize antibody-opsonized viruses via Fc-receptor-mediated phagocytosis. This results in antigen processing and presentation to effector cells of the adaptive immune system, eventually leading to virus clearance. On the other hand, neutrophils, monocytes, macrophages, mast cells and natural killer (NK) cells kill infected cells opsonized with antibodies in a process described as antibody-dependent cell-mediated cytotoxicity (ADCC). While immune complexes are expected to be phagocytosed by Fc-receptor bearing cells and cleared from the blood circulation, delivering viruses to monocytes or macrophages at subneutralizing antibody levels can result in antibody-enhanced infection due to their ability to serve as a host for viral replication. In support of this theory, subneutralizing levels of antibodies have been shown to result in increased infection of viruses such as dengue virus (DENV), Ross river virus, influenza, HIV-1 and Epstein–Barr virus (EBV) Citation[6–10]. Hence, therapeutic antibodies ought to be delivered at doses high enough to prevent antibody-enhanced virus infection and avoid the cytokine storm that can result from excessive stimulation of Fc-gamma receptors (FcγRs). Given the delicate balance between antibody efficacy and adverse side effects, there is a need to develop methods that can better assess Fc-mediated effector functions triggered by therapeutic antibodies. This could be beneficial in the future development of antibodies engineered to possess enhanced therapeutic activity Citation[11,12]. Here, we review the significance of the various aspects of Fc-mediated effector functions involved in virus neutralization and factors that can potentially affect FcγR engagement. An improved understanding of these processes would allow identification of predictive factors to better assess the efficacy of therapeutic antibodies and facilitate development of engineered therapeutic antibodies with improved efficacy.
Fc-receptor subtypes in virus neutralization
Among the different classes of immunoglobulins (IgA, IgD, IgE, IgG and IgM), IgG is the most commonly used and hence, will be the focus of this review. In general, antibodies consist of two distinct functional units: the Fab and the Fc regions . The Fab portion of the antibody contains the variable region comprising three hypervariable complementarity-determining regions that bind specific antigenic epitopes. The binding affinity of the Fab to viruses can be determined by binding assays such as ELISA or by more specialized and precise methods such as BIAcore surface plasmon resonance. Fab binding to viruses can block receptor interaction with the host, thereby hindering attachment and subsequent virus entry. In addition, several studies have suggested that antibodies that target regions other than the receptor-binding sites can also be neutralizing. For instance, antibodies that bind gp41, a HIV-1 fusogenic transmembrane protein, did not inhibit virus-cell binding but was able to neutralize HIV-1 infection by recognizing fusion-intermediate forms of gp41 at a postattachment step Citation[13,14]. Similarly, neutralizing antibodies that bind to West Nile virus and DENV have been shown to block infection at the postattachment step Citation[15,16]. Altogether, these investigations suggest that the Fab portion of neutralizing antibodies can function by either blocking virus attachment or inhibiting virus fusion.
Figure 1. Structure of an antibody and its associated Fc-mediated effector functions that can be activated by the Fc region. The Fab region is responsible for specific antigen binding whereas the Fc region binds to FcγRs in immune cells, resulting in phagocytosis, cytokine release, ADCC and CDC.
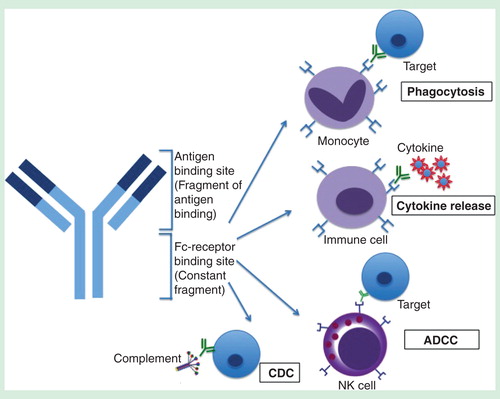
By contrast, the Fc portion of the antibody binds to FcγR-bearing immune cells of the mononuclear phagocyte system such as monocytes, macrophages and dendritic cells. FcγRs have been shown to be important in modulating the efficacy of therapeutic mAbs Citation[5] due to their involvement in FcγR-mediated phagocytosis, cytokine production, ADCC and complement-dependent cytotoxicity (CDC) that aids in virus neutralization . However, whether these Fc effector functions are activated or inhibited depends on the subtypes of FcγRs engaged.
Among the human-activating FcγRs, FcγRIA has the highest affinity for both monomeric IgG and immune complexes. FcγRIIA and FcγRIIIA, on the other hand, bind strongly to immune complexes but not to monomeric IgG Citation[17]. Both FcγRIA and FcγRIIIA require an accessory immunoreceptor tyrosine-based activation motif (ITAM) bearing gamma-chain for signal transduction. By contrast, the ITAM motif of FcγRIIA is located within its cytoplasmic tail . When activating FcγRs are clustered, src-related tyrosine kinases (e.g., Src, Fyn, Fgr, Hck and Lyn) are activated and phosphorylated, resulting in ITAM phosphorylation that mediates recruitment and activation of protein kinases of the spleen tyrosine kinase (Syk) and ZAP-70 family kinases Citation[18]. These activated kinases then catalyze the activation of downstream kinases, initiating a signaling cascade in several pathways to trigger immune effector functions. On the other hand, clustering of the inhibitory receptor FcγRIIB with activating FcγRs results in phosphorylation of immunoreceptor tyrosine-based inhibitory motifs (ITIMs) that recruit and activate phosphatases such as src homology 2 (SH2)-domain-containing inositol phosphatases SHIP-1, SHIP-2 and SH2-containing tyrosine phosphatases SHP-1 Citation[17,19,20]. These phosphatases then dephosphorylate the kinases downstream of ITAM-mediated signal transduction to inhibit activating FcγR signaling . Thus, coligation of inhibitory FcγRs with activating FcγRs results in abrogation of ITAM-mediated immune effector functions Citation[21,22].
Figure 2. Different FcγRs present in myeloid cells. Unlike FcγRII, FcγRI and FcγRIII require the common gamma chain for ITAM-mediated signaling. FcγRI binds strongly to IgG while FcγRII and FcγRIII bind strongly to immune complexes but not monomeric IgG. FcγRIIB is an inhibitory receptor bearing ITIM that counteracts ITAM-mediated signaling. FcRn can recycle IgG as it binds strongly to IgG at lower pH, resulting in increased half-life of antibodies in blood.
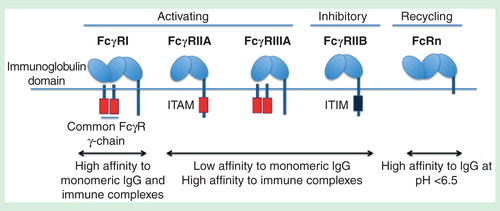
Interaction of activating FcγRs with antibodies can directly affect efficacy of therapeutic antibodies. For instance, the efficacy of clinically approved anticancer mAbs such as rituximab (anti-CD20 mAb) and trastuzumab (humanized anti-HER2 mAb) was significantly reduced in mice deficient in activating FcγRs compared to wild-type mice Citation[23,24]. This is in contrast to mice deficient in the inhibitory FcγRs, in which increased antibody-mediated cytolytic activity was observed Citation[25]. In addition, common single-nucleotide polymorphisms in FcγRIIA gene at position 131 (rs1801274) and in FcγRIIIA gene at position 158 (rs396991) can lead to significant reduction in binding to IgG Citation[26] and response to clinically approved mAbs such as trastuzumab, rituximab and cetuximab (anti-EGF mAb) Citation[27–31]. Furthermore, Fc modifications and the use of F(ab)2 that alter the interaction between Fc and FcγRs can directly affect Fc-effector functions and hence therapeutic outcome Citation[32]. For example, Hiatt et al. demonstrated that different glycan variants of palivizumab mediated different levels of ADCC, affecting in vivo efficacy of palivizumab against RSV Citation[33]. Overall, these studies suggest that therapeutic mAbs directed against similar antigenic targets may differ in their clinical profile depending on Fc–FcγR interaction.
The neonatal Fc receptor (FcRn) is a structurally distinct Fc-receptor expressed in various cell types (e.g., vascular endothelium cells, monocytes and macrophages) Citation[34,35]. FcRn binds to IgG within the acidic environment of endocytic vacuoles but not at physiological pH. Immune complexes that bind to FcRn are sorted to antigen-processing endosomes or recycling endosomes. However, monomeric IgGs are sorted into recycling endosomes, which recycle IgGs back to the cell surface following endocytosis, thus preventing intracellular degradation of IgGs. This allows antibodies or immune complexes to persist in the blood circulation for up to several weeks after treatment. Mutations at positions 250 (Thr250Gln) and 428 (Met428Leu) can significantly increase binding to FcRn and half-life of antibodies without affecting antigen-binding and Fc-mediated effector functions Citation[36,37]. While improved affinity to FcRn can extend the half-life of antibodies Citation[38]; this has never been linked with increased clinical efficacy. However, in vivo studies with a hFcRn/Rag1 knockout mouse model suggest that the antitumor activity of bevacizumab (anti-VEGF) and cetuximab (anti-EGF) can be significantly improved when binding to FcRn was increased Citation[39]. Furthermore, recent studies have shown that administration of a broadly neutralizing antibody with enhanced FcRn affinity can improve protection against HIV-1 infection in nonhuman primates, not only by increasing serum half-life but also by enhancing transcytosis of antibodies to mucosal surfaces Citation[40,41]. With slower clearance of antibodies and improved protection, less frequent dosing schedules would be expected, potentially reducing compliance issues. Moreover, the extended half-life would ensure that the levels of circulating antibodies are kept sufficiently high for virus neutralization. This would also minimize the risk of antibody-dependent enhancement (ADE) of virus infection, which would be particularly useful for viruses such as DENV, where ADE is hypothesized to result in increased viremia and increased risk of severe dengue Citation[6,42–44]. However, the effects of improved half-life of these antibodies should be tested to avoid any potential toxic adverse effects.
Given the importance of FcγRs in mediating virus neutralization and Fc effector functions, a better understanding of how therapeutic antibodies neutralize virus infections in FcγR-bearing cells will impact implementation of dosing regiments and allow development of improved therapeutic antibodies against infectious diseases. Here, we discuss the approaches that can be used to test for various Fc-mediated effector functions and describe how these processes could contribute to efficient neutralization of viruses.
Virus neutralization in the presence of FcγR-mediated phagocytosis
FcγR-mediated phagocytosis plays a critical role in clearing IgG-opsonized pathogens from the systemic circulation and augmenting antigen presentation Citation[45]. Cross-linking of FcγRs on phagocytes initiates downstream signaling that results in phosphorylation and activation of kinases such as phosphatidyl inositol 3-kinase, p70S6 kinase and Akt Citation[46,47], which are directly involved in reorganization of the actin cytoskeleton and membrane remodeling Citation[48]. Antibody-opsonized viruses are then directed to lysosomes, where the antigens are processed into peptides. In human monocytes, FcγRI has been shown to be involved in neutralization of virus immune complexes. For instance, reduction of antibody-opsonized HIV-1 infection in monocyte-derived macrophages was observed upon inhibition of FcγRI Citation[49]. Reducing FcγRI but not FcγRIIA expression using small interfering RNA also resulted in reduced antibody required for DENV neutralization in monocytes, reinforcing the role of FcγRI in DENV neutralization Citation[50]. While the exact mechanism involved remains to be fully elucidated, activation of FcγRI has been demonstrated to induce expression of proinflammatory mediators and is associated with trafficking of immune complexes that directly targets virus immune complexes to the late endosomes or lysosomes for enhanced antigen processing and antigen presentation to CD4+ T-cells Citation[51]. This can occur as early as 10 min after FcγRI cross-linking. In contrast, cross-linking of FcγRIIA resulted in slower trafficking of immune complexes, as trafficking to late endosomes or lysosomal compartments was not observed during the 10-min time interval Citation[51].
In the late endosomes or lysosomes, the viral proteins are degraded to peptide fragments that bind to MHC class II molecules and presented to the plasma membrane for CD4+ T-cell activation. Besides CD4+ responses, immune complexes have also been shown to increase CD8+ responses Citation[52,53] as immune complexes can be shuttled to antigen-processing endosomes to stimulate the MHC class I cross-presentation machinery Citation[54,55]. At present, the precise activating FcγRs involved in MHC class I cross-presentation still remain speculative. Besides neutralization in antigen-processing endosomes, antibody-opsonized viruses internalized into the cell may also bind to tripartite motif-containing protein 21, a cytosolic IgG receptor that targets antibody-opsonized viruses for proteasomal degradation via the E3 ubiquitin ligase activity Citation[56,57]. Of interest, this mode of neutralization has been shown to be particularly effective against nonenveloped viruses such as adenovirus Citation[58,59]. The significance of antigen-presentation in the presence of antigen uptake has also been demonstrated in vivo, where protection mediated by antibodies was eliminated in mice with dendritic cells deprived of β-2 microglobulin, transporter associated with antigen processing (TAP) or MHC-II Citation[60].
However, IgG-mediated uptake of viruses is not always favorable for the host. Monocytes and macrophages are important sites of replication for viruses such as DENV, Ross river virus, influenza, HIV-1 and Epstein–Barr virus (EBV). Increased internalization of antibody-opsonized viruses via FcγRs may, therefore, result in enhanced infection if these antibodies are not present at sufficient levels required for virus neutralization. This can occur via extrinsic or intrinsic ADE Citation[61]. In extrinsic ADE, immune complexes formed between viruses and subneutralizing levels of antibodies can lead to an increased number of infected cells due to increased uptake by FcγR-mediated phagocytosis. Besides increased uptake, FcγR-mediated signaling can also increase production of anti-inflammatory cytokines such as IL-10 that acts via the suppressor of cytokine signaling system to suppresses innate immunity, resulting in increased virus replication and output by infected cells Citation[61]. In addition, some viruses can also directly ligate other inhibitory receptors to suppress innate immunity. For instance, we have recently demonstrated that antibody-opsonized dengue can coligate the inhibitory receptor, leukocyte immunoglobulin-like receptor subfamily B member 1 (LILRB1), to activate the phosphatase SHP-1 that inhibits activation of signal transducer and activator of transcription 1 (STAT-1) and subsequent interferon-stimulated gene (ISG) expression Citation[62]. LILRB1 has also been shown to interact with other viral antigens such as human cytomegalovirus glycoprotein UL-18 Citation[63,64]. Although both FcγRI and FcγRIIA can mediate ADE of virus infection, FcγRIIA appears to do so more effectively Citation[50,65]. Hence, for pathogens that can replicate efficiently in monocytes and macrophages, it is important to ensure that therapeutic antibodies can neutralize these viruses even in the presence of FcγR-mediated phagocytosis to minimize the risk of ADE.
FcγR-mediated phagocytosis is affected by the size of immune aggregates, as immune complex size can affect the types of Fc-receptors engaged Citation[16]. Aggregation refers to the self-association of a number of molecules to form dimers, oligomers or even submicron to micron-sized complexes, which can be assessed by dynamic light scattering or Nanoparticle Tracking Analysis Citation[66]. Antibody concentration, affinity and stability can influence the size of immune complexes. Our previous observations indicate that while small immune complexes coligate activating FcγRs, increased antibody concentrations can result in the formation of large immune aggregates that coligate the lowly expressed inhibitory receptor FcγRIIB to inhibit phagocytosis Citation[16]. In line with our observations, Morefield et al. observed that dendritic cells preferentially phagocytose smaller aggregates of ∼3 μm compared with aggregates greater than 10 μm Citation[67]. In another study by Fifis et al. that used model polystyrene particles, smaller particles of <0.5 um (especially 40–50 nm) were delivered more efficiently to DEC205+ dendritic cells, triggered the production of higher antibody titers and promoted better type I CD8 and CD4 T cell responses when compared with larger particles Citation[68]. Finally, antibody-mediated protection against Cryptococcus neoformans was shown to be protective at optimal doses, but administration of large amounts of antibodies abrogates protection as phagocytic index was shown to decline at higher antibody concentrations Citation[69]. As such, determining whether the virus immune complexes can be neutralized intracellularly could potentially predict efficacy of the therapeutic antibody. These antibodies should preferably neutralize virus infections at levels that do not form viral aggregates, as this would ensure that virus neutralization occurs in the presence of FcγR-mediated entry to result in enhanced antigen presentation and activation of adaptive immune responses.
Figure 3. The different FcγRs involved in virus neutralization at various antibody concentrations. At high antibody concentrations, immune aggregates formed can coligate the lowly expressed inhibitory FcγRIIB to inhibit FcγR-mediated phagocytosis. Small sized immune complexes, in contrast, utilize the activating FcγRs signaling for phagocytosis.
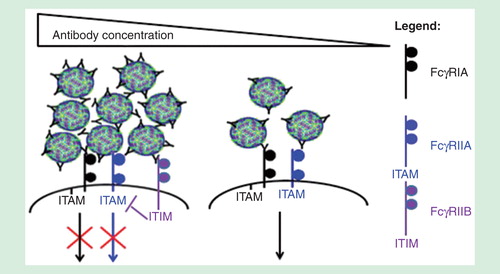
Cytokine production for antigen presentation & antiviral responses
Cytokine production triggered by activating FcγR signaling events promotes antiviral responses and activation of DCs, leading to increased antigen uptake and processing. In addition, these cytokines allow recruitment of monocytes and DCs to the site of infection so as to aid in virus clearance Citation[70]. Activating FcγR signaling has been shown to phosphorylate spleen tyrosine kinase (Syk) that results in up-regulation and activation of STAT-1, leading to up-regulation of cytokines and ISGs critical for antiviral responses. While the intermediate signaling molecules have yet to be completely elucidated, we and others have shown that the activation of STAT-1 can occur independently of interferon signaling Citation[62,71]. The significance of STAT-1 in immunity has been shown in STAT1 knockout studies, where STAT1 knockout results in significant defects in ISGs production and dysregulation of T-cell, macrophage and DC differentiation, resulting in TH2-biased immune responses that delay viral clearance Citation[72]. Besides STAT-1, protein kinase C expression and activation have been shown to be important in MHC class II stimulation and presentation Citation[73], a process pivotal for optimal CD4+ T-cell activation.
The strength and nature of immune responses triggered by activating FcγRs, however, are determined by pairing of activating and inhibitory responses. Activating FcγRs signal through ITAM to recruit and activate kinases to trigger immune effector functions. By contrast, inhibitory receptors signal through immunoreceptor tyrosine-based inhibitory motif (ITIM) to activate phosphatases that can inhibit ITAM function. For example, immune aggregates can coligate FcγRIIB, resulting in recruitment of phosphatases such as SHIP-1 and SHP-1 that inhibit FcγR-mediated phagocytosis Citation[16,74]. As described previously, some viruses can also coligate inhibitory receptors such as LILRB1 to activate SHP-1 to reduce cytokine and ISG production. Inhibitory receptors hence control a range of cellular responses mediated by activating FcγR-signaling, which include Fc-effector functions such as FcγR-mediated phagocytosis, cytokine production, ADCC and CDC. While inhibitory receptor coligation can potentially reduce the therapeutic efficacy of mAbs, not all of these effects are undesirable. For instance, intravenous immunoglobulin can signal through FcγRIIB to induce anti-inflammatory responses that can potentially reduce the risk of cytokine release syndrome in mAb therapy Citation[75–77]. Given the importance of kinases and phosphatases in mediating innate and adaptive immune responses, determining kinase and phosphatase profiles in FcγR-bearing cells mediated by therapeutic antibodies could hence predict the efficacy of these antibodies in controlling virus infection.
Although kinases triggered by IgG and immune complexes are important for Fc-mediated effector functions, overproduction of cytokines (e.g., IL-1b, TNFa, IL-6 and IL-8) due to immune complexes can result in immunogenicity-related adverse events Citation[78]. For instance, the Phase I trial of the anti-CD28 mAb TGN1412 resulted in immune-mediated cytokine storm and multiorgan failure in healthy volunteers, highlighting the importance of assessing the likelihood of such adverse events Citation[79]. The need to assess the risk of such adverse events is even more crucial for engineered antibodies that have modified Fc regions and increased binding to activating FcγRs. While improved Fc–FcγR interactions can increase therapeutic efficacy of antibodies, this can also lead to increased risk of adverse events. Hence, a cytokine signature encompassing the relevant proinflammatory and anti-inflammatory genes can potentially be used as a biomarker for immune responses to immune complexes, which would in turn be useful for predicting the clinical immunogenic risk of therapeutic antibodies. Cytokine signatures can be evaluated based on: breadth of cytokine response; magnitude of response; number of individuals having the cytokine response; and statistical significance of the response. These cytokine signatures can be determined by ELISAs, Luminex or other cytokine arrays.
ADCC & CDC clears virus infected cells
ADCC is critical for clearance of antibody-opsonized cell surface receptor targets. When surface antigens are opsonized with antibodies, Fc-receptor interaction with the host immune cells such as NK cells, monocytes, macrophages, neutrophils and mast cells can result in direct lysis of the infected cells, eventually leading to virus clearance. As many of these cell types express both activating and inhibitory FcγRs, FcγRIIB may hence affect activating FcγR-dependent ADCC. Among the activating FcγRs, FcγRIIIA is the most abundant FcγR expressed on NK cells, and ADCC signaling is better characterized with FcγRIIIA relative to other activating FcγRs Citation[80]. The importance of FcγRIIIA in mediating ADCC has been highlighted by studies showing a lack in clinical efficacy for several antibodies (e.g., infliximab and rituximab) in individuals with different FcγRIIIA polymorphisms Citation[30]. These observations were correlated with in vitro ADCC analysis where peripheral blood mononuclear cells obtained from FcγRIIA H/H and/or FcγRIIIA V/V genotype showed higher ADCC activity compared with those of other genotypes with lesser affinity to IgG Citation[28]. Previous research has also demonstrated the importance of ADCC in viral defense and clearance. For instance, studies have shown both in vivo and in vitro the importance of ADCC in controlling influenza, RSV, and HIV-1 infections in vivo and in human studies Citation[81–85].
Complement-mediated activation by antibodies, on the other hand, can cause direct cell lysis by formation of the membrane attack complex (MAC). This process, also termed as CDC, is initiated by binding of C1q to antibody complexes. This results in the formation of membrane-bound C3 and C5 convertases that cleave and activate C3 and C5, respectively. C5 cleavage by C5 convertase then forms C5a and C5b, where C5b mediates the formation of membrane attack complex (MAC) that can directly cause CDC by forming membrane pores on targeted cells. Simultaneously, the cleavage of C3 results in the formation of C3b that can be rapidly degraded into fragments iC3b and C3dg. Deposition of these fragments on the target cells can then bind to CR3 expressed on leukocytes, leading to phagocytosis and ADCC Citation[86], resulting in the neutralization of viruses. Several clinically approved mAbs have been shown to mediate their functions by activating complement. For instance, antibodies such as edrecolomab (antiepithelial cell adhesion molecule mouse mAb) and alemtuzumab (anti-CD52 mAb) can activate complement and mediate ADCC Citation[87,88].
Figure 4. Immune complexes can trigger the complement pathway to result in either eventual lysis or phagocytosis of target antigen. C1q binding to immune complexes results in formation of C3 and C5 convertases that cleave C3 and C5, respectively. Cleavage of C3 results in fragments iC3b and C3dg that binds to CR3 present in leukocytes or NK-cells, resulting in phagocytosis and ADCC. C5 cleavage, on the other hand, results in formation of MAC that forms membrane pores and eventual lysis of target. Phagocytosis, ADCC and lysis assays can be used to assess involvement of complements.
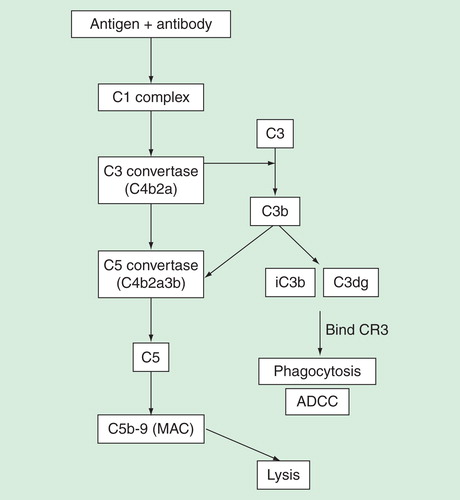
Fc modifications that improve FcγR interaction
Several modifications to IgG can directly affect binding to activating FcγRs and subsequent cellular activation. For instance, the IgG1 triple mutant (S298A/E333A/L334A) has been shown to increase binding to FcγRIIIA and ADCC activity Citation[89]. Fc variants (S239D/I332E and S238D/I332E/A330L mutations) designed computationally possessed increased binding to FcγRIIIA and reduced binding to FcγRIIB, resulting in increased effector functions Citation[90]. The S238D/I332E/A330L mutation, however, led to significant reduction in CDC Citation[90]. An anti-HER2 antibody engineered to bear multiple substitutions (L235V, F243L, R292P, Y300L and P396L) also showed increased binding to FcγRIIIA and enhanced killing of HER2-expressing cancer cells Citation[91]. Finally, given the critical role of Fc-linked glycans in FcγR-binding, differences in glycan structure can affect binding and function of therapeutic antibodies. For example, afucosylated antibodies bind FcγRIIIA with better affinity and have enhanced ability to mediate ADCC Citation[92]. While these modified antibodies can have improved efficacy, it is essential to ensure that they do not cause immunogenicity or adverse events when administered in patients. As such, assays to assess antidrug antibody responses are now a mainstay at most drug regulatory authorities for evaluating therapeutic antibodies during preclinical development. By improving binding to activating FcγRs and enhancing effector functions, Fc modifications could benefit patients with FcγR polymorphisms that render them less responsive to therapeutic antibody treatment. Enhanced ADCC and CDC effector functions could also engage the immune system in a synergistic manner for more potent virus neutralization.
Conclusion
The treatment of viral infections using therapeutic antibodies remains a challenging task. Besides generating high-affinity antibodies against viruses, therapeutic antibodies should also trigger Fc-mediated effector functions that facilitate virus neutralization. These effector functions include increased uptake via FcγR-mediated phagocytosis, increased cytokine production leading to enhanced antigen presentation and antiviral responses, increased ADCC and CDC. Combinatorial therapeutic strategies that trigger Fc-mediated effector functions while occluding the interaction with immune inhibitory receptors could also enhance the clinical efficacy of therapeutic antibodies. Finally, for viruses that infect and replicate within FcγR-bearing cells, therapeutic antibodies should mediate virus neutralization even in the presence of FcγR-mediated uptake, thus mitigating the risks of ADE.
Expert commentary
With the increased focus on antibody-based therapeutics, there is a burgeoning need to develop robust methods that can rapidly and accurately assess antibody binding and Fc-receptor-mediated effector functions. Routine testing and measurement of Fc-mediated effects should hence be considered for characterization of therapeutic antibodies, as this would allow selection and development of therapeutic antibodies with improved efficacy and reduced side effects. In addition, as the antiviral antibody responses are polyclonal and comprise different antibody isotypes, the effects of therapeutic antibodies in the presence of these antibodies should also be tested.
Given the importance of Fc–FcγR interaction in antibody-mediated effector functions, Fc modification could lead to the development of therapeutic antibodies with improved interaction to activating FcγRs. This could enhance FcγR-mediated uptake, cytokine production, antigen presentation, ADCC and CDC. Future optimization to improve interaction with FcRn may also increase half-life of therapeutic antibodies, thereby reducing the risk of ADE associated with the use of therapeutic antibodies against viruses that infect FcγR-bearing cells. With a growing number of methods to assess Fc-mediated effector functions and technologies in antibody modification, we believe that more therapeutic antibodies against viral diseases will be developed in the near future. This will be useful for disease management, especially during epidemics.
Five-year view
Given the absence of licensed vaccines for many viruses, we believe that therapeutic antibodies will likely be important for treatment of viral infections, particularly during epidemics. We speculate that future advancement in antibody engineering resulting in improved Fc–FcγR interaction will continue to aid the development of therapeutic antibodies that possess improved clinical efficacy. Better and more robust methods that can predict Fc functionality and Fc-associated effects clinically will also continue to be essential to select for therapeutic antibodies with good efficacy but with minimal side effects.
The lack of effective vaccines against multiple infectious diseases necessitates the development of new therapeutics.
Therapeutic antibodies are increasingly used for the treatment of infectious diseases as they are well-established and well-tolerated by humans.
Therapeutic antibodies targeted against similar antigenic targets may differ in clinical profile, depending on the type of FcγRs that are engaged.
Interaction between antibody Fc region and activating FcγRs can trigger Fc-mediated effector functions that are essential for virus neutralization.
For viruses that can infect FcγR-bearing cells, therapeutic antibodies should be able neutralize viruses intracellularly to minimize the risk of ADE.
Cytokine production from activating FcγR signaling is essential for enhanced antigen presentation and antiviral responses.
Antibody-dependent cell-mediated cytotoxicity and complement-dependent cytotoxicity mediated by therapeutic antibodies can directly kill virus infected cells.
A better understanding of how therapeutic antibodies neutralize virus infections can allow development of modified therapeutic antibodies with improved therapeutic efficacy and reduced side effects.
Financial & competing interests disclosure
The authors have no relevant affiliations or financial involvement with any organization or entity with a financial interest in or financial conflict with the subject matter or materials discussed in the manuscript. This includes employment, consultancies, honoraria, stock ownership or options, expert testimony, grants or patents received or pending, or royalties.
Notes
References
- Qiu X, Wong G, Audet J, et al. Reversion of advanced Ebola virus disease in nonhuman primates with ZMapp. Nature 2014;514(7520):47-53
- Zhu Z, Bossart KN, Bishop KA, et al. Exceptionally potent cross-reactive neutralization of Nipah and Hendra viruses by a human monoclonal antibody. J Infect Dis 2008;197(6):846-53
- Palivizumab, a humanized respiratory syncytial virus monoclonal antibody, reduces hospitalization from respiratory syncytial virus infection in high-risk infants. Pediatrics 1998;102(3):531-7
- Thakkar S, Nanaware-Kharade N, Celikel R, et al. Affinity improvement of a therapeutic antibody to methamphetamine and amphetamine through structure-based antibody engineering. Sci Rep 2014;4:3673
- Jiang XR, Song A, Bergelson S, et al. Advances in the assessment and control of the effector functions of therapeutic antibodies. Nat Rev Drug Discov 2011;10(2):101-11
- Halstead SB. Pathogenesis of dengue: challenges to molecular biology. Science 1988;239(4839):476-81
- Linn ML, Aaskov JG, Suhrbier A. Antibody-dependent enhancement and persistence in macrophages of an arbovirus associated with arthritis. J Gen Virol 1996;77(Pt 3):407-11
- Tamura M, Webster RG, Ennis FA. Subtype cross-reactive, infection-enhancing antibody responses to influenza A viruses. J Virol 1994;68(6):3499-504
- Robinson WEJr, Montefiori DC, Mitchell WM, et al. Antibody-dependent enhancement of human immunodeficiency virus type 1 (HIV-1) infection in vitro by serum from HIV-1-infected and passively immunized chimpanzees. Proc Natl Acad Sci USA 1989;86(12):4710-14
- Robinson WEJr, Montefiori DC, Mitchell WM. Antibody-dependent enhancement of human immunodeficiency virus type 1 infection. Lancet 1988;1(8589):790-4
- DiLillo DJ, Ravetch JV. Fc-Receptor Interactions Regulate Both Cytotoxic and Immunomodulatory Therapeutic Antibody Effector Functions. Cancer Immunol Res 2015;3(7):704-13
- Nimmerjahn F, Gordan S, Lux A. FcgammaR dependent mechanisms of cytotoxic, agonistic, and neutralizing antibody activities. Trends Immunol 2015;36(6):325-36
- Ugolini S, Mondor I, Parren PW, et al. Inhibition of virus attachment to CD4+ target cells is a major mechanism of T cell line-adapted HIV-1 neutralization. J Exp Med 1997;186(8):1287-98
- de Rosny E, Vassell R, Jiang S, et al. Binding of the 2F5 monoclonal antibody to native and fusion-intermediate forms of human immunodeficiency virus type 1 gp41: implications for fusion-inducing conformational changes. J Virol 2004;78(5):2627-31
- Thompson BS, Moesker B, Smit JM, et al. A therapeutic antibody against west nile virus neutralizes infection by blocking fusion within endosomes. PLoS Pathog 2009;5(5):e1000453
- Chan KR, Zhang SL, Tan HC, et al. Ligation of Fc gamma receptor IIB inhibits antibody-dependent enhancement of dengue virus infection. Proc Natl Acad Sci USA 2011;108(30):12479-84
- Nimmerjahn F, Ravetch JV. Fcgamma receptors as regulators of immune responses. Nat Rev Immunol 2008;8(1):34-47
- Iwashima M, Irving BA, van Oers NS, et al. Sequential interactions of the TCR with two distinct cytoplasmic tyrosine kinases. Science 1994;263(5150):1136-9
- Huang ZY, Hunter S, Kim MK, et al. The effect of phosphatases SHP-1 and SHIP-1 on signaling by the ITIM- and ITAM-containing Fcgamma receptors FcgammaRIIB and FcgammaRIIA. J Leuk Biol 2003;73(6):823-9
- Kant AM, De P, Peng X, et al. SHP-1 regulates Fcgamma receptor-mediated phagocytosis and the activation of RAC. Blood 2002;100(5):1852-9
- Boruchov AM, Heller G, Veri MC, et al. Activating and inhibitory IgG Fc receptors on human DCs mediate opposing functions. J Clin Invest 2005;115(10):2914-23
- Clynes RA, Towers TL, Presta LG, Ravetch JV. Inhibitory Fc receptors modulate in vivo cytotoxicity against tumor targets. Nat Med 2000;6(4):443-6
- Zhang M, Zhang Z, Garmestani K, et al. Activating Fc receptors are required for antitumor efficacy of the antibodies directed toward CD25 in a murine model of adult t-cell leukemia. Cancer Res 2004;64(16):5825-9
- Bulliard Y, Jolicoeur R, Windman M, et al. Activating Fc gamma receptors contribute to the antitumor activities of immunoregulatory receptor-targeting antibodies. J Exp Med 2013;210(9):1685-93
- Wernersson S, Karlsson MC, Dahlstrom J, et al. IgG-mediated enhancement of antibody responses is low in Fc receptor gamma chain-deficient mice and increased in Fc gamma RII-deficient mice. J Immunol 1999;163(2):618-22
- Bruhns P, Iannascoli B, England P, et al. Specificity and affinity of human Fcgamma receptors and their polymorphic variants for human IgG subclasses. Blood 2009;113(16):3716-25
- Weng WK, Levy R. Two immunoglobulin G fragment C receptor polymorphisms independently predict response to rituximab in patients with follicular lymphoma. J Clin Oncol 2003;21(21):3940-7
- Musolino A, Naldi N, Bortesi B, et al. Immunoglobulin G fragment C receptor polymorphisms and clinical efficacy of trastuzumab-based therapy in patients with HER-2/neu-positive metastatic breast cancer. J Clin Oncol 2008;26(11):1789-96
- Cheung NK, Sowers R, Vickers AJ, et al. FCGR2A polymorphism is correlated with clinical outcome after immunotherapy of neuroblastoma with anti-GD2 antibody and granulocyte macrophage colony-stimulating factor. J Clin Oncol 2006;24(18):2885-90
- Canete JD, Suarez B, Hernandez MV, et al. Influence of variants of Fc gamma receptors IIA and IIIA on the American College of Rheumatology and European League Against Rheumatism responses to anti-tumour necrosis factor alpha therapy in rheumatoid arthritis. Ann Rheum Dis 2009;68(10):1547-52
- Bibeau F, Lopez-Crapez E, Di Fiore F, et al. Impact of Fc{gamma}RIIa-Fc{gamma}RIIIa polymorphisms and KRAS mutations on the clinical outcome of patients with metastatic colorectal cancer treated with cetuximab plus irinotecan. J Clin Oncol 2009;27(7):1122-9
- Liu Z, Gunasekaran K, Wang W, et al. Asymmetrical Fc engineering greatly enhances antibody-dependent cellular cytotoxicity (ADCC) effector function and stability of the modified antibodies. J Biol Chem 2014;289(6):3571-90
- Hiatt A, Bohorova N, Bohorov O, et al. Glycan variants of a respiratory syncytial virus antibody with enhanced effector function and in vivo efficacy. Proc Natl Acad Sci USA 2014;111(16):5992-7
- Akilesh S, Christianson GJ, Roopenian DC, Shaw AS. Neonatal FcR expression in bone marrow-derived cells functions to protect serum IgG from catabolism. J Immunol 2007;179(7):4580-8
- Zhu X, Meng G, Dickinson BL, et al. MHC class I-related neonatal Fc receptor for IgG is functionally expressed in monocytes, intestinal macrophages, and dendritic cells. J Immunol 2001;166(5):3266-76
- Hinton PR, Xiong JM, Johlfs MG, et al. An engineered human IgG1 antibody with longer serum half-life. J Immunol 2006;176(1):346-56
- Datta-Mannan A, Witcher DR, Tang Y, et al. Monoclonal antibody clearance. Impact of modulating the interaction of IgG with the neonatal Fc receptor. J Biol Chem 2007;282(3):1709-17
- Roopenian DC, Christianson GJ, Sproule TJ, et al. The MHC class I-like IgG receptor controls perinatal IgG transport, IgG homeostasis, and fate of IgG-Fc-coupled drugs. J Immunol 2003;170(7):3528-33
- Zalevsky J, Chamberlain AK, Horton HM, et al. Enhanced antibody half-life improves in vivo activity. Nat Biotechnol 2010;28(2):157-9
- Ko SY, Pegu A, Rudicell RS, et al. Enhanced neonatal Fc receptor function improves protection against primate SHIV infection. Nature 2014;514(7524):642-5
- Armitage CW, O’Meara CP, Harvie MC, et al. Divergent outcomes following transcytosis of IgG targeting intracellular and extracellular chlamydial antigens. Immunol Cell Biol 2014;92(5):417-26
- Simmons CP, Chau TN, Thuy TT, et al. Maternal antibody and viral factors in the pathogenesis of dengue virus in infants. J Infect Dis 2007;196(3):416-24
- Halstead SB, O’Rourke EJ. Dengue viruses and mononuclear phagocytes. I. Infection enhancement by non-neutralizing antibody. J Exp Med 1977;146(1):201-17
- Kliks SC, Nimmanitya S, Nisalak A, Burke DS. Evidence that maternal dengue antibodies are important in the development of dengue hemorrhagic fever in infants. Am J Trop Med Hygiene 1988;38(2):411-19
- Guilliams M, Bruhns P, Saeys Y, et al. The function of Fcgamma receptors in dendritic cells and macrophages. Nature reviews. Immunology 2014;14(2):94-108
- Araki N, Hatae T, Furukawa A, Swanson JA. Phosphoinositide-3-kinase-independent contractile activities associated with Fcgamma-receptor-mediated phagocytosis and macropinocytosis in macrophages. J Cell Sci 2003;116(Pt 2):247-57
- Ganesan LP, Wei G, Pengal RA, et al. The serine/threonine kinase Akt Promotes Fc gamma receptor-mediated phagocytosis in murine macrophages through the activation of p70S6 kinase. J Biol Chem 2004;279(52):54416-25
- Huang ZY, Barreda DR, Worth RG, et al. Differential kinase requirements in human and mouse Fc-gamma receptor phagocytosis and endocytosis. J Leuk Biol 2006;80(6):1553-62
- Holl V, Hemmerter S, Burrer R, et al. Involvement of Fc gamma RI (CD64) in the mechanism of HIV-1 inhibition by polyclonal IgG purified from infected patients in cultured monocyte-derived macrophages. J Immunol 2004;173(10):6274-83
- Chawla T, Chan KR, Zhang SL, et al. Dengue virus neutralization in cells expressing Fc gamma receptors. PLoS ONE 2013;8(5):e65231
- Dai X, Jayapal M, Tay HK, et al. Differential signal transduction, membrane trafficking, and immune effector functions mediated by FcgammaRI versus FcgammaRIIa. Blood 2009;114(2):318-27
- Furlan SN, Mandraju R, Brewer T, et al. Enhancement of anti-tumor CD8 immunity by IgG1-mediated targeting of Fc receptors. mAbs 2014;6(1):108-18
- Regnault A, Lankar D, Lacabanne V, et al. Fcgamma receptor-mediated induction of dendritic cell maturation and major histocompatibility complex class I-restricted antigen presentation after immune complex internalization. J Exp Med 1999;189(2):371-80
- Amigorena S. Fc gamma receptors and cross-presentation in dendritic cells. J Exp Med 2002;195(1):F1-3
- Platzer B, Stout M, Fiebiger E. Antigen cross-presentation of immune complexes. Front Immunol 2014;5:140
- McEwan WA, Mallery DL, Rhodes DA, et al. Intracellular antibody-mediated immunity and the role of TRIM21. Bioessays 2011;33(11):803-9
- Mallery DL, McEwan WA, Bidgood SR, et al. Antibodies mediate intracellular immunity through tripartite motif-containing 21 (TRIM21). Proc Nat Acad Sci USA 2010;107(46):19985-90
- Vaysburd M, Watkinson RE, Cooper H, et al. Intracellular antibody receptor TRIM21 prevents fatal viral infection. Proc Nat Acad Sci USA 2013;110(30):12397-401
- McEwan WA, Tam JC, Watkinson RE, et al. Intracellular antibody-bound pathogens stimulate immune signaling via the Fc receptor TRIM21. Nat Immunol 2013;14(4):327-36
- Rafiq K, Bergtold A, Clynes R. Immune complex-mediated antigen presentation induces tumor immunity. J Clin Invest 2002;110(1):71-9
- Halstead SB, Mahalingam S, Marovich MA, et al. Intrinsic antibody-dependent enhancement of microbial infection in macrophages: disease regulation by immune complexes. Lancet Infect Dis 2010;10(10):712-22
- Chan KR, Ong EZ, Tan HC, et al. Leukocyte immunoglobulin-like receptor B1 is critical for antibody-dependent dengue. Proc Nat Acad Sci USA 2014;111(7):2722-7
- Yang Z, Bjorkman PJ. Structure of UL18, a peptide-binding viral MHC mimic, bound to a host inhibitory receptor. Proc Natl Acad Sci USA 2008;105(29):10095-100
- Cosman D, Fanger N, Borges L, et al. A novel immunoglobulin superfamily receptor for cellular and viral MHC class I molecules. Immunity 1997;7(2):273-82
- Rodrigo WW, Jin X, Blackley SD, et al. Differential enhancement of dengue virus immune complex infectivity mediated by signaling-competent and signaling-incompetent human Fcgamma RIA (CD64) or FcgammaRIIA (CD32). J Virol 2006;80(20):10128-38
- Filipe V, Hawe A, Jiskoot W. Critical evaluation of Nanoparticle Tracking Analysis (NTA) by NanoSight for the measurement of nanoparticles and protein aggregates. Pharmaceut Res 2010;27(5):796-810
- Morefield GL, Sokolovska A, Jiang D, et al. Role of aluminum-containing adjuvants in antigen internalization by dendritic cells in vitro. Vaccine 2005;23(13):1588-95
- Fifis T, Gamvrellis A, Crimeen-Irwin B, et al. Size-dependent immunogenicity: therapeutic and protective properties of nano-vaccines against tumors. J Immunol 2004;173(5):3148-54
- Macura N, Zhang T, Casadevall A. Dependence of macrophage phagocytic efficacy on antibody concentration. Infect Immun 2007;75(4):1904-15
- Lev A, Sigal L. Getting in front and behind the enemy lines to counter virus infection. Cell Host Microbe 2013;13(2):121-2
- Dhodapkar KM, Banerjee D, Connolly J, et al. Selective blockade of the inhibitory Fcgamma receptor (FcgammaRIIB) in human dendritic cells and monocytes induces a type I interferon response program. J Exp Med 2007;204(6):1359-69
- Zornetzer GA, Frieman MB, Rosenzweig E, et al. Transcriptomic analysis reveals a mechanism for a prefibrotic phenotype in STAT1 knockout mice during severe acute respiratory syndrome coronavirus infection. J Virol 2010;84(21):11297-309
- Majewski M, Bose TO, Sille FC, et al. Protein kinase C delta stimulates antigen presentation by Class II MHC in murine dendritic cells. Int Immunol 2007;19(6):719-32
- Tridandapani S, Siefker K, Teillaud JL, et al. Regulated expression and inhibitory function of Fcgamma RIIb in human monocytic cells. J Biol Chem 2002;277(7):5082-9
- Samuelsson A, Towers TL, Ravetch JV. Anti-inflammatory activity of IVIG mediated through the inhibitory Fc receptor. Science 2001;291(5503):484-6
- Kaneko Y, Nimmerjahn F, Ravetch JV. Anti-inflammatory activity of immunoglobulin G resulting from Fc sialylation. Science 2006;313(5787):670-3
- Nimmerjahn F, Ravetch JV. The antiinflammatory activity of IgG: the intravenous IgG paradox. J Exp Med 2007;204(1):11-15
- Lee DW, Gardner R, Porter DL, et al. Current concepts in the diagnosis and management of cytokine release syndrome. Blood 2014;124(2):188-95
- Suntharalingam G, Perry MR, Ward S, et al. Cytokine storm in a phase 1 trial of the anti-CD28 monoclonal antibody TGN1412. N Eng J Med 2006;355(10):1018-28
- Mandelboim O, Malik P, Davis DM, et al. Human CD16 as a lysis receptor mediating direct natural killer cell cytotoxicity. Proc Natl Acad Sci USA 1999;96(10):5640-4
- Hashimoto G, Wright PF, Karzon DT. Antibody-dependent cell-mediated cytotoxicity against influenza virus-infected cells. J Infect Dis 1983;148(5):785-94
- Milligan C, Richardson BA, John-Stewart G, et al. Passively acquired antibody-dependent cellular cytotoxicity (ADCC) activity in HIV-infected infants is associated with reduced mortality. Cell Host Microbe 2015;17(4):500-6
- Hessell AJ, Rakasz EG, Poignard P, et al. Broadly neutralizing human anti-HIV antibody 2G12 is effective in protection against mucosal SHIV challenge even at low serum neutralizing titers. PLoS Pathog 2009;5(5):e1000433
- Hessell AJ, Hangartner L, Hunter M, et al. Fc receptor but not complement binding is important in antibody protection against HIV. Nature 2007;449(7158):101-4
- Lambotte O, Ferrari G, Moog C, et al. Heterogeneous neutralizing antibody and antibody-dependent cell cytotoxicity responses in HIV-1 elite controllers. AIDS 2009;23(8):897-906
- Gelderman KA, Tomlinson S, Ross GD, Gorter A. Complement function in mAb-mediated cancer immunotherapy. Trends Immunol 2004;25(3):158-64
- Trikha M, Yan L, Nakada MT. Monoclonal antibodies as therapeutics in oncology. Curr Opin Biotechnol 2002;13(6):609-14
- Velders MP, Litvinov SV, Warnaar SO, et al. New chimeric anti-pancarcinoma monoclonal antibody with superior cytotoxicity-mediating potency. Cancer Res 1994;54(7):1753-9
- Shields RL, Namenuk AK, Hong K, et al. High resolution mapping of the binding site on human IgG1 for Fc gamma RI, Fc gamma RII, Fc gamma RIII, and FcRn and design of IgG1 variants with improved binding to the Fc gamma R. J Biol Chem 2001;276(9):6591-604
- Lazar GA, Dang W, Karki S, et al. Engineered antibody Fc variants with enhanced effector function. Proc Nat Acad Sci USA 2006;103(11):4005-10
- Nordstrom JL, Gorlatov S, Zhang W, et al. Anti-tumor activity and toxicokinetics analysis of MGAH22, an anti-HER2 monoclonal antibody with enhanced Fcgamma receptor binding properties. Breast Cancer Res 2011;13(6):R123
- Shields RL, Lai J, Keck R, et al. Lack of fucose on human IgG1 N-linked oligosaccharide improves binding to human Fcgamma RIII and antibody-dependent cellular toxicity. J Biol Chem 2002;277(30):26733-40