Pathogen sensors of the innate immune system recognize the molecular signatures of pathogens and activate antimicrobial defenses and initiate inflammatory response by MyD88-dependent and independent pathways. Viral and bacterial proteins that are known to inhibit signal transduction pathways are shown in red.
AMP: Antimicrobial peptide; ds: Double stranded; HCV: Hepatitis C virus; IFN: Interferon; IκB: Inhibitor κB; IKK: IkB kinase; IPS: IFN-β promoter stimulator 1; IRAK: Interleukin-1 receptor-associated kinase; IRF: Interferon regulation factor; MyD: Myeloid differentiation primary-response gene; NF: Nuclear factor; NOD: Nucleotide-binding oligomerization domain; NS: Nonstructural; RIG: Retinoic acid inducible gene; ss: Single stranded; TBK: TANK-binding kinase; TLR: Toll-like receptor; TRAF: Tumor necrosis factor receptor-associated factor.
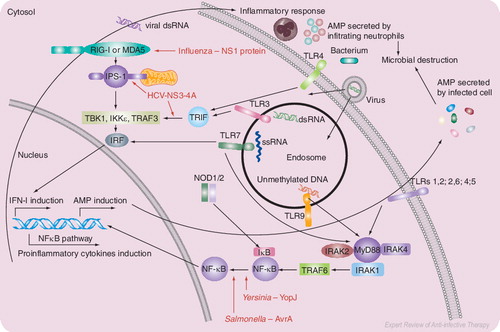
For over 60 years, antibiotics have enabled millions of people to survive infections that would have been lethal in earlier eras. However, the emergence of pathogens that are resistant to current antibacterial or antiviral agents is increasingly common, and relatively few novel anti-infective agents are being developed. Those seeking new antimicrobial agents and strategies might find it rewarding to explore the innate immune system.
Huge numbers of microbes, including many potential pathogens, inhabit the skin and portions of the gastrointestinal, genitourinary and respiratory tracts. The fact that people are not constantly sick is largely attributable to innate immune defenses. Cutaneous and mucosal epithelial cells produce peptides and proteins that can modulate the composition of this microbial flora and select innocuous or even beneficial microflora. In addition, multiple innate cellular receptors recognize molecular signals elaborated when microbial or viral pathogens invade portions of the body that normally remain sterile. Engagement of these tell-tale ligands activates these cellular receptors and initiates the synthesis and secretion of various gene-encoded defense polypeptides, including the defensins discussed below. Collectively, these peptides not only kill a broad spectrum of bacteria and fungi, they also exert antiviral and/or antitoxic properties.
Pathogens that reach the inner sancta of the body may reside in various locales, depending on their metabolic needs, preferences (tropisms), portals of entry and their ability to exploit ‘soft spots’ in the host’s immunological surveillance (pathogen sensors) and control (antimicrobial polypeptides and many other mechanisms).
Pathogen sensors of the innate immune system
The discovery of Toll-like receptors (TLRs) and, subsequently, of other pattern-recognition receptors (PRRs) has greatly invigorated interest in innate immunity. These other PRRs include: nucleotide-binding oligomerization domain (NOD)-like receptors (NLRs), retinoic acid inducible gene (RIG)-I-like receptors (RLRs) and lectin-like receptors (LLRs) Citation[1]. PRRs recognize pathogen-associated molecular patterns, which include structural components, such as lipopolysaccharide (LPS), lipopeptides and β-glucans; microbial proteins, such as flagellin and porins; F-protein of respiratory syncytial virus (RSV); or genetic material, such as unmethylated bacterial DNA and single- or double-stranded RNA. Interaction of the innate immune receptors with these pathogen-derived signature molecules activates signal transduction cascades that ultimately influence gene transcription, with outcomes that enhance antibacterial and antiviral defences Citation[2]. These include the secretion of pro-inflammatory cytokines and chemokines, repair of damaged tissues and activation of adaptive immune responses directed at reversing the pathogen’s replication and survival . However, these signal transduction processes are tightly regulated to prevent chronic inflammatory conditions.
Pathogens that overcome the structural and biochemical defences of epithelia and enter the normally sterile interior will encounter a second array of innate defence molecules, some produced by locally resident cells and others delivered by immigrant blood leukocytes, especially polymorphonucleated neutrophils (PMNs). These motile phagocytes are attracted to infected sites by chemical signals that may emanate from the pathogen (e.g., the N-formylated polypeptides elaborated by bacteria), from the pathogen’s interaction with complement (e.g., C5a) or from cytokines (e.g., interleukin [IL]-8) produced by tissue cells equipped with the PRRs described below. The impressive host defense armamentarium of PMNs is composed of de novo-generated oxidants and various presynthesized polypeptides. The oxidants produced by human PMNs consist primarily of ‘reactive oxygen intermediates’ (ROI). These are produced by NADPH oxidase and include superoxide anions (O2-), hydrogen peroxide and (perhaps) singlet oxygen and hydroxyl radicals. Human PMNs contain myeloperoxidase, an enzyme that converts H2O2 to more formidable oxidants, including hypochlorous acid and chloramines. The oxidants produced by human neutrophils could be called ‘drugs of the past’, given their use for topical disinfection. In addition to elaborating ROI, murine PMNs (but not human PMNs) produce antimicrobial amounts of nitric oxide via an inducible nitric oxide synthase (iNOS).
Human PMNs complement their generation of antimicrobial ROI by storing five antimicrobial peptides in their cytoplasmic granules, including four α-defensins (HNPs 1–4) and one cathelicidin (a peptide whose synthetic precursors contain a conserved ~100-amino acid domain, known as cathelin; hCAP-18/LL-37). HNPs 1–3 comprise 30–50% of the protein in a neutrophil’s primary (azurophil) granules. Although HNPs 1–3 are also produced by human natural killer cells, human macrophages ‘outsource’ their α-defensins, obtaining them by ingesting apoptotic PMNs or by imbibing fluid-phase defensins after their secretion from PMNs (unpublished data). Importantly, human macrophages route such imported defensins to their phagolysosomes, an optimal arena in which to display their antimicrobial properties Citation[3]. The secondary (specific) granules of human PMNs also contain hCAP18/LL-37 – a cathelin-containing propeptide whose C-terminal domain comprises LL-37, an α-helical ‘cathelicidin’ with antimicrobial properties Citation[4]. The granules of human PMNs also contain antimicrobial proteins, including cathepsin G, azurocidin/CAP37, bactericidal/permeability-inducing factor (BPI)/CAP57, lysozyme and lactoferrin.
Like those of humans, murine PMNs contain one cathelicidin (MCRAMP), lysozyme and lactoferrin. However, their PMNs lack defensins, BPI, azurocidin and cathepsin G. Curiously, Paneth cells in the murine small intestine express over 20 different α-defensins (cryptdins), whereas human Paneth cells express only two (HD-5 and -6) and cathepsin G is expressed in murine NK cells, whereas human NK cells express the homologous serine protease, granzyme B. Perhaps the presence of two systems in murine PMNs that actively generate reactive oxidants (NADPH oxidase and iNOS) allows these cells to skimp on antimicrobial peptides and proteins. Given the wide use of murine models, differences between murine and human PMNs may have important implications for infections in which PMNs contribute to host defense (i.e., most infections).
The presence of multiple antimicrobial peptides is not unique to human PMNs. Bovine PMNs contain more than a dozen different β-defensins (BNBDs), as well as a variety of different cathelicidins. The bovine cathelicidins include a 12-residue cyclic peptide called bactenecin dodecapeptide, a 13-residue, tryptophan-rich peptide called indolicidin, three α-helical peptides (BMAPs) containing 27-, 28- and 34-amino acid residues, respectively, and two proline and arginine-rich peptides called bactenecins 5 and 7. Porcine PMNs lack α- and β-defensins, but contain multiple cathelicidin peptides. These include at least three protegrins, a proline- and arginine-rich rich peptide (PR-39), two proline- and phenylalanine-rich peptides (prophenins 1 and 2), two α-helical peptides, PMAP-23 and PMAP-36 and tritrpticin (VRRFPWWWPFLRR), a tryptophan-rich and almost palindromic tridecapeptide. Given their structural variety, cathelicidin peptides provide many leads for designing new antimicrobial peptides and a substantial preclinical literature exists describing such efforts.
Only two antimicrobial peptides based on molecules of the innate immune system have been tested in controlled Phase III clinical trials, all of which involved their topical administration. Pexiganan (MSI-78) is a 22-residue α-helical peptide similar to magainin-2, a peptide found in the skin of Xenopus, the African clawed frog. Iseganan (IB-367) is a 17-residue peptide that resembles protegrin-1, a potent antimicrobial peptide found in porcine PMNs. Although topically administered, Pexiganan cream was approximately as effective as oral ofloxacin in treating patients with diabetic foot ulcers. However, this equivalence did not result in US FDA approval. Treatment with iseganan-containing mouth rinses did not prevent oral mucositis in outpatients receiving chemotherapy or radiation for cancer, nor did it prevent ‘ventilator-associated pneumonia’ in hospitalized patients receiving prolonged mechanical ventilation Citation[5–7].
Given the need to discuss a large topic in a few words, we will limit our remaining comments to two areas: the pathogen sensors whose activation might awaken or enhance existing innate resistance mechanisms and the possibilities of using defensin-like molecules as systemic therapeutics.
Defensins
α-, β- and θ-defensins are antimicrobial peptides whose common ancestry can be traced back as far as bony fishes. They are cationic (positively charged) peptides whose largely β-sheet structures are stabilized by three intramolecular cystine disulfide bonds. α and β-defensins have similar backbone folds but differ in the placement, spacing and connectivity of their six conserved cysteines. Humans express six different α-defensins and 30 or more different defensins in total. α-defensins HNP 1–4 are present in neutrophils, and HNP 1–3 have been demonstrated in NK cells and γδ-T cells. α-defensins-5 and -6 are stored in and secreted by small intestinal Paneth cells. Deficient production of HD-5 and -6, secondary to mutation of the NOD2 cytoplasmic peptidoglycan sensor, has been associated with ileal Crohn’s disease. There is marked copy number polymorphism of many human β-defensin genes, and a relative deficiency of HBD2 secondary to a low gene-copy number may predispose individuals to colonic Crohn’s disease Citation[8]. Since Crohn’s disease affects over a million individuals worldwide and appears to be increasing in incidence, replacement therapy with appropriate exogenous defensins might prove beneficial.
One major factor – their cost-effective production – has long impeded the development of therapeutic defensins. Unlike peptide hormones or cytokines, which are typically effective at or below nanomolar concentrations, antimicrobial peptides work at low micromolar concentrations. Since the daily production rate of endogenous HNPs 1–3 is in the order of 10–15 mg/kg body weight/day, the required daily dose of an exogenous defensin could be of similar magnitude. A recent report describing the fungal defensin, plectasin, documented not only the peptide’s efficacy in treating experimental pneumococcal infection, but also its cost-effective recombinant production, low toxicity and favorable pharmacokinetics Citation[9]. Although the plectasin report suggests that cost-effective synthesis of human defensin will also be feasible, it may be that the first defensins to enter therapy will derive from the phylogenetically older constellation of defensins that fungi and bacteria have expressed for the past billion years.
Antiviral response of the host is characterized by the secretion of type 1 interferon (IFN-1), which results from the interaction of extracellular double-stranded RNA and single-stranded RNA with TLR3 and TLR7 Citation[10]. While TLR7 uses myeloid differentiation primary response protein 88 (Myd88) as an adaptor protein for IFN-I signaling, TLR3 signals through a Myd88-independent pathway, which involves Toll/interleukin-1 receptor (TIR) domain-containing, adaptor-inducing IFN-β (TRIF). In addition to TLR-dependent and -independent pathways, IFN-I is also induced by RIG-I or melanoma differentiation-associated gene (MDA) 5 present in cytosol through recognition of intracellular double-stranded RNA, a component or replication product of many RNA viruses. RIG-I and MDA5 contain caspase-recruitment domains at their N-termini and RNA helicase domains at their C-termini. Both RIG-I and MDA-5 interact with dsRNA through their helicase domains and initiate IFN-I production by interacting with IFN-β promoter stimulator (IPS)-1, also known as Cardif, MAVS and VISA. Several human viruses, namely hepatitis C virus (HCV), vaccinia virus, Ebola virus and influenza virus, have evolved strategies to overcome antiviral responses of the host by targeting TLR-dependent and -independent pathways. Since a detailed discussion of microbial evasive mechanisms is beyond the scope of this editorial, we only mention a couple of examples. The viral protease NS3/4A encoded by HCV inactivates TRIF and IPS-1 adaptor proteins in the TLR3- and RIG-I-dependent pathways, respectively, to prevent IFN-I production. The authors and others have demonstrated recently that nonstructural protein (NS)1 of influenza A virus targets RIG-I Citation[11–14]. Similar to HCV, influenza and other viruses, several bacterial pathogens developed strategies to overcome innate immune responses by interfering with signal transduction cascades leading to the activation of antimicrobial defenses, such as inhibiting nuclear factor (NF)κB activation. The YopJ and AvrA proteins of Yersinia and Salmonella have been shown to inhibit NFκB activation and prevent the activation of antimicrobial defences and initiation of the inflammatory response.
Strategies to enhance antimicrobial defences
Mother Nature has endowed both unicellular and multicellular organisms with complex systems, the ability to resist the microbial onslaught with innate immune responses, without which we would be unable to survive as species. Today we are faced with the serious problem of emerging bacteria and viruses that are resistant to antibiotics and antivirals, especially at a time when there are few drugs in development. Our understanding of the innate immune system, the frontline of host defense, as well as evasive microbial strategies at the molecular level, provides clues for the development of strategies either alone or in combination to tackle the drug resistant microbes. RIG-I, MDA5, IPS1, TRAF3, as well the ligands for TLR3, TLR7, RIG-I and MDA5 are all logical targets to overcome antiviral resistance, combat pathogen strategies to overcome innate immune defenses and to develop new drugs. Developing synthetic small molecules that modulate pathogen-sensing mechanisms is crucial not only to treat chronic infections, but also to deal with the antimicrobial resistance. In support of this approach, a synthetic TLR7 ligand, imiquimod, has been developed and is licensed for treating genital herpes, although the mechanism of its action at the molecular level did not become evident until later. Identification of PRRs and their transduction paths and intersections is a vibrant area of current research, which will lead to the development of newer agents with the potential to enhance antimicrobial defenses against antibiotic- and antiviral-resistant strains, ameliorate chronic inflammatory conditions and enhance the efficacy of preventive and therapeutic vaccines.
Disclaimer
The findings and conclusions in this report are those of the authors and do not necessarily represent the views of the US CDC, The Agency for Toxic Substances and Disease Registry or the funding agency.
Conflict of Interest
Sambhara has no conflict of interest. Lehrer has received patents on antimicrobial peptides, all of which are assigned to the University of California (CA, USA). He currently serves on the Scientific Advisory Board of Novozymes, which is developing a fungal defensin (plectasin) as a potential therapeutic agent.
References
- Creagh EM, O’Neill LA. TLRs, NLRs and RLRs: a trinity of pathogen sensors that co-operate in innate immunity. Trends Immunol.27, 352–357 (2006).
- Oda K, Kitano H. A comprehensive map of the toll-like receptor signaling network. Mol. Syst. Biol.2 (2006).
- Tan BH, Meinken C, Bastian M et al. Macrophages acquire neutrophil granules for antimicrobial activity against intracellular pathogens. J. Immunol.177, 1864–1871 (2006).
- Zanetti M. The role of cathelicidins in the innate host defenses of mammals. Curr. Issues Mol. Biol.7, 179–196 (2005).
- Giles HG, Miller R, Macleod SM, Sellers EM. Diazepam and N-desmethyldiazepam in saliva of hospital inpatients. J. Clin. Pharmacol.20, 71–76 (1980).
- Kollef M, Pittet D, Sanchez GM et al. A randomized double-blind trial of iseganan in prevention of ventilator-associated pneumonia. Am. J. Respir. Crit. Care Med.173, 91–97 (2006).
- Trotti A, Garden A, Warde P et al. A multinational, randomized Phase III trial of iseganan HCl oral solution for reducing the severity of oral mucositis in patients receiving radiotherapy for head-and-neck malignancy. Int. J. Radiat. Oncol. Biol. Phys.58, 674–681 (2004).
- Wehkamp J, Stange EF. A new look at Crohn’s disease: breakdown of the mucosal antibacterial defense. Ann. NY Acad. Sci.1072, 321–331 (2006).
- Mygind PH, Fischer RL, Schnorr KM et al. Plectasin is a peptide antibiotic with therapeutic potential from a saprophytic fungus. Nature437, 975–980 (2005).
- Meylan E, Tschopp J. Toll-like receptors and RNA helicases: two parallel ways to trigger antiviral responses. Mol. Cell 22, 561–569 (2006).
- Guo Z, Chen LM, Zeng H et al. NS1 protein of influenza A virus inhibits the function of intracytoplasmic pathogen sensor, RIG-I. Am. J. Respir. Cell. Mol. Biol. (2006) (Epub ahead of print).
- Mibayashi M, Martinez-Sobrido L, Loo YM, Cardenas WB, Gale M Jr, Garcia-Sastre A. Inhibition of retinoic acid-inducible gene-I-mediated induction of interferon-β by the NS1 protein of influenza A virus. J. Virol.81, 514–524 (2007).
- Opitz B, Rejaibi A, Dauber B et al. IFNβ induction by influenza A virus is mediated by RIG-I which is regulated by the viral NS1 protein. Cell. Microbiol. (2006) (Epub ahead of print).
- Pichlmair A, Schulz O, Tan CP et al. RIG-I-mediated antiviral responses to single-stranded RNA bearing 5´-phosphates. Science314, 997–1001 (2006).