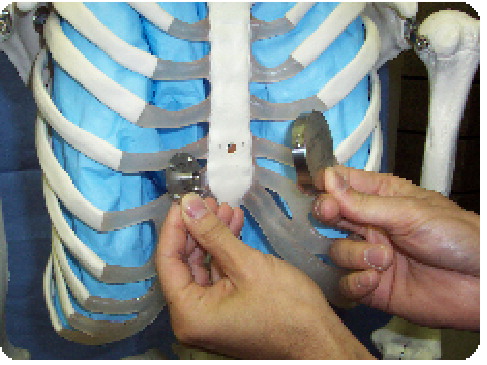
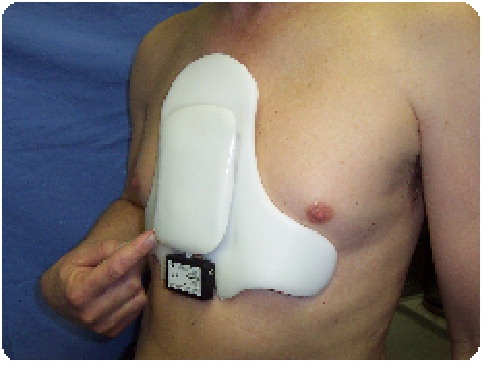
Pectus excavatum, or ‘sunken chest’, is the most common congenital chest wall deformity with an incidence of approximately 1 in 1000 births. It is characterized by posterior depression of the sternum and lower anterior costal cartilages. Despite over 60 years of research, opinions vary on whether this anatomic anomaly causes significant cardiopulmonary deficits. A variety of studies have evaluated an array of pulmonary function indices before and after correction of pectus excavatum but the results are inconsistent. Most studies do, however, generally concur that there is some measurable improvement in lung volumes after correction.
Cardiac effects have also garnered much attention as compression of the mediastinum between the sternum and vertebral column generally indents the anterior wall of the right ventricle. This is thought to contribute to the development of mitral valve prolapse, which is present in a fifth to two thirds of patients. Approximately half of these cases of prolapse resolve after operation. Again, results have varied when trying to demonstrate improvements in quantities, such as ejection fraction and stroke volume, but exercise tolerance generally appears to improve with operation.
While some evidence exists that the altered thoracic geometry can affect cardiopulmonary function, many consider the milder forms of pectus excavatum to simply be a cosmetic issue. This latter view is widely espoused by American insurers, who – swayed by their financial interests – are quick to define this as a cosmetic variant and decline reimbursement for surgical correction. However, discussion with actual patients and their families reveals the significant impact of the condition on patients’ daily lives. Younger children may be undisturbed by their anatomic differences but as they reach adolescence their social development is frequently stunted by the embarrassment of their deformity. Even in those children whose cardiac function or exercise tolerance is not limited, there is a detriment to their development. Parents who themselves have lived with the deformity are crestfallen when they see the same in their children, aware of the social difficulties and self-esteem issues they will face. The bottom line is that pectus excavatum is a genuine and disturbing problem for patients.
Pathogenesis
The development of most pectus abnormalities is poorly understood. Some cases are iatrogenic, resulting from undue posterior tension on the sternum after surgical repairs, such as occurs with congenital diaphragmatic hernia. Theories of altered intrauterine pressure and aberrant diaphragm muscle fiber insertion have been postulated. Higher than expected (i.e., nonrandom) coincidence with scoliosis and Marfan’s syndrome also suggests a possible contribution from connective tissue disease. A third of patients have a family member who is similarly affected, which suggests a genetic component with incomplete penetrance; however, no direct evidence exists. Whatever the mechanism, the central abnormality involves aberrant costochondral tissue, which is the cartilage that links each rib with the breastbone; its overgrowth forces subduction of the sternum from its normal position.
Traditional management
Treatment of pectus excavatum centers on surgically reshaping the chest cage. Since it is a fundamentally anatomic disorder, pharmaceutical treatment has not been proposed. Operative solutions focus on reconstruction of the chest wall, all variations on a similar theme that was initially developed and described by Ravitch Citation[1–3]. The Ravitch repair requires exposure of the costal cartilage and sternum with removal of the abnormal cartilage and fixation of the sternum with a metal bar for approximately 1 year. A major revision to the operation came around with Nuss’ technique, which allows for smaller incisions and videoscopic assistance Citation[4,5]. However, while the Nuss method avoids resection of cartilage, the bar has to be left in place almost twice as long in order to force remodeling. This requires a second operation for bar removal and is extremely uncomfortable for patients. Both operations require general anesthetic and several days of hospitalization for pain control (often managed initially via an epidural catheter). Surgeons who perform the operations generally feel that the procedures lack finesse and describe them as ‘brutal’ Citation[6]. Clearly, the existing solutions to the problem are inadequate and a new treatment is required: one that is less painful and less radical.
Paradigm shift
The unifying principle of traditional treatments for pectus excavatum is that they attempt to apply a corrective force on the sternum in a single step Citation[7–9]. In our lab, we challenged this philosophy by thinking about means of applying a small force over long periods to gradually force remodeling of the chest wall. This is analogous to the concept underlying orthodontics, in which a relatively gentle perturbative force is applied continually in order to gradually force rearrangement. Some groups in Europe have independently pursued this line of thinking with respect to chest deformities, developing tensioned wire devices and even a giant suction cup Citation[10]. While both of these devices show creative thought, they have practical limitations. By contrast, we began to explore the feasibility of employing magnetic force.
Medical magnets
Traditionally, magnets have been used in two realms of medicine: theories of holistic healing and diagnostic imaging (magnetic resonance imaging). To our knowledge, no medical device has been developed that relies on the attractive or repulsive forces that can be generated by a pair of magnets. We set out to design a means of applying magnet-generated force to shape the sternum. The resulting magnetic mini-mover procedure (3MP) involves implantation of a magnet attached to the sternum Citation[6]. This internal device is encased in titanium and completely implanted so that infectious risks are reduced . By itself, this lone magnet has no effect. However, when a magnet-bearing external orthotic is brought nearby, the two attract . The external component braces against the lateral ribs and upper sternum, thus the magnetic attraction force applies outward traction on the sternum. The initially constructed device has morphed over the course of multiple revisions to take the form represented in the figures. The outer device is low-profile and allows for adjustment of magnet position and strength to adapt to the patients’ anatomy over the course of the treatment. It also has an incorporated force sensor and data logger to permit recording of activity; this feature will not only be informative, but will also allow monitoring of patient compliance.
Development
Some initial experiments have been conducted to prove the principle of action, as well as to demonstrate biologic safety of low-field magnets implanted in close proximity to mediastinal organs.
The first of such studies involved characterizing the magnitude of the magnetic field to which the heart is subjected. Ex vivo measurements of the system revealed that our rare earth magnet generated fields in the order of 10-2 T. At a distance of 1 cm (the minimum distance from sternum to heart), the field strength was 4 × 10-2 T (400 Gauss), which was well below the accepted safety limit of 4 T Citation[11–13].
The forces generated by the magnet pair were measured as high as 45 N at a magnet separation distance of 1 cm. As proof of principle, a device was constructed to apply similar force within the chest cavity of rabbits. Over months, the application of these forces did indeed deform the chest wall, confirming remodeling of the costal cartilage. This stands in contradistinction to prior studies that suggest forces of 150–200 N are required to displace the sternum but it must be highlighted that these experiments focused on immediate, static displacement whereas our forces are not meant to generate immediate movement but rather to force gradual movement and remodeling.
Rabbits were also used to verify the safety of a modest-strength implantable magnet with respect to cardiac function. A series of rabbits with magnets implanted in their chests demonstrated no ill effects as measured by survival, growth, cardiac electrical function and cardiac histology – even when followed for up to a year.
Another concern regarding the static magnetic field was the external field; we wanted to be sure there was no risk of attraction to nearby metal or interference with other implantable medical devices, such as pacemakers. The maximum field at the outer surface of our external device was a modest 150 Gauss. Nonetheless, the device was modified by the application of a thin strip of metal with altered permittivity that attenuated the external field to 10 Gauss, which we believe to be inconsequential.
Clinical evaluation
This magnet-based device for the treatment of pectus excavatum has been granted investigational device approval by the US FDA for clinical trial evaluation of safety and efficacy (IDE G050196-A002). We are currently enrolling patients and should have our first procedures completed by the time this article goes to press. The initial study involves children aged 8–14 years, in whom we will implant the magnet and follow the time course of correction closely. While we have plenty of preliminary data to responsibly begin treating patients, we cannot reliably predict the rapidity of correction or frequency of adjustments that will be required.
Our design team consists of pediatric surgeons, a physicist, a clinical engineer, a machinist and an orthotist. We believe that a multidisciplinary group, such as ours, benefits from a rich blend of backgrounds, skills and viewpoints. Our guiding principle is not to work on revisions of existing treatments, but to imagine and explore new treatment paradigms. While the magnetic mini-mover procedure is the most mature of the developments, several projects are in developmental and experimental phases and we look forward to a description of other treatments involving the application of magnetic forces in the treatment of human disease.
References
- Ravitch MM. The operative treatment of pectus excavatum. Ann. Surg.129, 429–444 (1949).
- Fonkalsrud EW. Current management of pectus excavatum. World J. Surg.27(5), 502–508 (2003).
- Sbokos CG, McMillan IK, Akins CW. Surgical correction of pectus excavatum using a retrosternal bar. Thorax30(1), 40–45 (1975).
- Nuss D, Kelly RE Jr, Croitoru DP, Katz ME. 10-year review of a minimally invasive technique for the correction of pectus excavatum. J. Pediatr. Surg.33(4), 545–552 (1998).
- Nuss D. Recent experiences with minimally invasive pectus excavatum repair “Nuss procedure”. Jpn. J. Thorac. Cardiovasc. Surg.53(7), 338–344 (2005).
- Harrison MR, Estefan-Ventura D, Fechter R, Moran AM Jr, Christensen D. Magnetic mini-mover procedure for pectus excavatum: I. Development, design, and simulations for feasibility and safety. J. Pediatr. Surg.42(1), 81–85 (2007).
- Weber PG, Huemmer HP, Reingruber B. Forces to be overcome in correction of pectus excavatum. J. Thorac. Cardiovasc. Surg.132(6), 1369–1373 (2006).
- Boia ES, Susan-Resiga R, Raicov PC, Popoiu CM, Iacob RE. Determination of the mechanical requirements for progressive correction system of pectus excavatum in children. J. Laparoendosc. Adv. Surg. Tech.15(5), 478–481 (2005).
- Fonkalsrud EW, Reemtsen B. Force required to elevate the sternum of pectus excavatum patients. J. Am. Coll. Surg.195(4), 575–577 (2002).
- Schier F, Bahr M, Klobe E. The vacuum chest wall lifter: an innovative, nonsurgical addition to management of pectus excavatum. J. Pediatr. Surg.40(3), 496–500 (2005).
- Chakeres DW, Kangarlu A, Boudoulas H, Young DC. Effect of static magnetic field exposure of up to 8 Tesla on sequential human vital sign measurements. J. Magn. Reson. Imaging18(3), 346–352 (2003).
- Sorahan T, Nichols L. Mortality from cardiovascular disease in relation to magnetic field exposure: findings from a study of UK electricity generation and transmission workers, 1973–1997. Am. J. Indust. Med.45(1), 93–102 (2004).
- Tenforde TS. Magnetically induced fields and currents in the circulatory system. Prog. Biophys. Mol. Biol.87, 279–288 (2005).