Diamond–Blackfan anemia (DBA) is an inherited bone marrow failure syndrome that presents in childhood with macrocytic normochromic anemia and sometimes with variable accompanying developmental anomalies, chiefly short stature, thumb abnormalities and cleft palate Citation[1,2]. In the blood the erythrocyte lineage is most affected though pancytopenia and multilineage bone marrow hypoplasia are sometimes seen. In the majority of patients, the disease results from heterozygous mutations or deletions in genes encoding ribosomal proteins (RP), suggesting haploinsufficiency for RPs triggers the disease Citation[3]. Eleven RP genes have so far been implicated affecting both large and small ribosomal subunits and together accounting for 70% of cases. The mechanism whereby haploinsufficiency for RPs leads to failure of red cell development and the other DBA manifestations is unknown. Research into DBA pathogenesis has been hampered by the rarity of the disease and the scarcity of the cells, in particular hematopoietic stem cells and precursors that are defective in the disease. Currently, there are two main hypotheses in the field to explain the disease mechanism: one is that haploinsufficiency for RPs leads to stabilization of p53 and apoptosis of erythroid precursors, which require massive ribosome synthesis for globin production Citation[4,5], though the details of this model have not been rigorously established. Alternatively, it is thought that haploinsufficiency of ribosomal proteins may affect translation of specific transcripts required for erythropoiesis Citation[6].
One of the intriguing characteristics of DBA is that disease severity varies to the extent that members of the same family carrying the same pathogenic mutation can have very different clinical presentations; they can be transfusion dependent, dependent on steroid treatment or completely asymptomatic Citation[7,8]. Furthermore, patients with therapy-dependent disease can spontaneously enter hematological remission and become therapy independent. The likelihood of patients going into remission is about 20% by age 25 (8). The mechanism responsible for remission is not known but in some instances gene conversion has been found suggesting that genetic differences might be responsible for the variability of disease expression and that these may be acquired or inherited Citation[9]. Understanding the pathogenic mechanism per se, and the mechanisms underlying variable penetrance and spontaneous remission is essential if we are to design rational and effective treatments.
New possibilities for studying rare and intractable diseases have opened up with the development of induced pluripotent stem (iPS) cell protocols Citation[10]. Using this approach differentiated cells such as fibroblasts or peripheral blood mononuclear cells can be reprogrammed by expression of specific transcription factors, to produce pluripotent stem cells that resemble embryonic stem cells. These cells can then be coaxed into differentiating into different tissues such as hematopoietic, cardiac, pancreatic or neural tissue by culturing in the presence of appropriate growth factors and cytokines. If the iPS cells are derived from a patient with an inherited mutation, the effect of this mutation on in vitro differentiation can be investigated and will be informative concerning the in vivo pathogenic mechanism Citation[11]. The usefulness of the iPS cell model is illustrated by the recent production of iPS cells reprogrammed from skin fibroblasts from DBA patients Citation[12]. These cells recapitulate the aberrant ribosome biogenesis that is seen in cells from DBA patients. They also show defective red cell production, the major hallmark of DBA. Thus, researchers can produce essentially infinite numbers of cells that show the DBA defect, where previously these precious cells made up a small part of the bone marrow of DBA patients. There are several technical hurdles that the iPS cell field needs to overcome. One unsolved problem related to the differentiation of iPS cells into hematopoietic lineages is how to obtain terminal and functional subpopulations of cells. A second caveat that applies to iPS cells representing hematological diseases is that they can be readily made to undergo primitive (fetal), but not definitive (adult) hematopoiesis, though progress is being made to overcome this problem Citation[13]. Nevertheless, erythropoiesis from DBA iPS cells was defective encouraging the idea that they will accurately reflect the molecular pathogenesis of the disease.
Figure 1. Possible applications of induced pluripotent stem cells.iPSC: Induced human pluripotent stem cells.
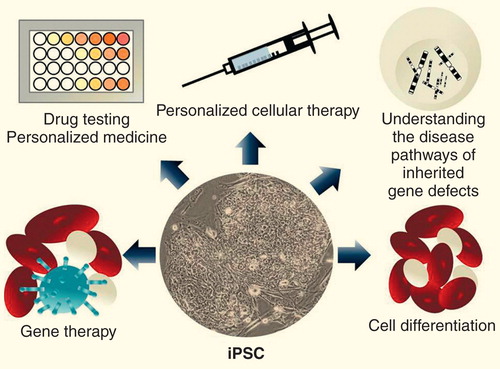
In the short term, iPS cells will be most useful in working out pathogenetic mechanisms. Using high throughput approaches, where all expressed RNAs or proteins are quantified, differences between normal and disease iPS cells, before and during differentiation, can be discovered and quantified. These differences could be in protein, mRNA, non-coding RNAs or in DNA methylation or histone modification. These kinds of data can be used to generate hypotheses about the pathogenetic pathway which can then be tested using the iPS cells. This step is facilitated by parallel technological improvements in methods for manipulating the genome Citation[14]. It is hoped that these techniques will enable researchers to readily produce iPS cells containing simple point mutations or more complex deletions and rearrangements to probe the contribution of individual genes to the disease phenotype. Already, it is a simple matter to express a gene, or an inhibitory RNA, by recombining it into a site in the genome, called a safe harbor site, from which it will be expressed in a regulated manner Citation[15]. This approach was used in the DBA iPS cells to demonstrate that a wild-type copy of the ribosomal protein gene that was haploinsufficient in the patient, was sufficient to rescue the iPS cell from the disease phenotype Citation[12]. It is easy to imagine that gene products downstream of the pathogenic mutation may also correct the phenotype, enabling the reconstruction of the disease pathway.
Another use for iPS cells will be in testing or even discovering drugs that might be useful in treatment Citation[16]. If an assay is available, for DBA this could be erythrocyte production in a differentiation assay, then different compounds could be tested for their effectiveness. Similarly, drugs suspected to be beneficial could be tested in an iPS cell assay in preclinical studies. In the case of DBA, it would be interesting to investigate the effect on the iPS cell phenotype of drugs previously used for treatment; these include valproic acid, calcineurin inhibitors and metoclopramide as well as new drugs such as leucine Citation[15], lenalidomine Citation[17] or sotatercept, a soluble activin receptor Type 2A antagonist Citation[101]. A great advantage of the system is that patient-specific cells, representing a wide range of mutations and background genotypes could be tested in a short time and ex vivo. The work would not be restricted to cells reprogrammed from patients since pathogenic mutations can be induced in non-DBA cells by genetic manipulation.
The clinical use of iPS cells directly is one of the hopes for the future in bone marrow failure syndromes like DBA or indeed in degenerative diseases in general. The idea of taking cells from a patient's skin or cheek lining, reprogramming the cells to pluripotency, repairing the pathogenic mutation and then using the cells in vitro or in vivo to regenerate the defective tissue, with no fear of immunological rejection, is beautiful in its simplicity, and technically it seems that it might work. The major problem that stands in the way of this protocol being put into practice is the fact that during the process of growing the patient's cells, selecting the iPS cell clone and expanding the cells to form tissue there are many opportunities for the selection of faster growing clones of cells. By definition cells carrying oncogenic mutations, whose growth will be less constrained by cellular regulatory mechanisms, will be selected for Citation[18]. The selection would be particularly strong in cells derived from diseases like DBA, which are due to stem cell defects and the baseline cellular proliferation is poor. In the meantime if only cells without nuclei, such as red cells Citation[19] or platelets Citation[20], can be produced from iPS cells the danger of malignancy would not be there, leaving only the challenge of clinical grade mass production of these cells before red cell and platelet disorders become candidates for these futuristic therapies.
Acknowledgements
The authors would like to thank all of the patients and their families for their continuing participation in our bone marrow failure studies.
Financial & competing interests disclosure
The work has been supported by the Buck Family Endowed Chair in Hematology, and by NCI NIH grants 2R01CA106995 to PJ Mason, and 2R01 CA105312 to M Bessler. The authors have no other relevant affiliations or financial involvement with any organization or entity with a financial interest in or financial conflict with the subject matter or materials discussed in the manuscript apart from those disclosed.
No writing assistance was utilized in the production of this manuscript.
References
- Lipton JM, Ellis SR.. Diamond-Blackfan anemia: diagnosis, treatment, and molecular pathogenesis. Hematol. Oncol. Clin. North Am. 23(2), 261–282 (2009).
- Lipton JM, Ellis SR.. Diamond Blackfan anemia 2008–2009: broadening the scope of ribosome biogenesis disorders. Curr. Opin. Pediatr. 22(1), 12–19 (2010).
- Boria I, Garelli E, Gazda HT et al. The ribosomal basis of Diamond-Blackfan Anemia: mutation and database update. Hum. Mutat. 31(12), 1269–1279 (2010).
- Boultwood J, Pellagatti A, Wainscoat JS.. Haploinsufficiency of ribosomal proteins and p53 activation in anemia: Diamond-Blackfan anemia and the 5q- syndrome. Adv. Biol. Regul. 52(1), 196–203 (2012).
- Narla A, Hurst SN, Ebert BL.. Ribosome defects in disorders of erythropoiesis. Int. J. Hematol. 93(2), 144–149 (2011).
- Horos R, Ijspeert H, Pospisilova D et al. Ribosomal deficiencies in Diamond-Blackfan anemia impair translation of transcripts essential for differentiation of murine and human erythroblasts. Blood 119(1), 262–272 (2012).
- Vlachos A, Ball S, Dahl N et al. Diagnosing and treating Diamond Blackfan anaemia: results of an international clinical consensus conference. Br. J. Haematol. 142(6), 859–876 (2008).
- Vlachos A and Muir E. How I treat Diamond-Blackfan anemia. Blood 116(19), 3715–3723 (2010).
- Farrar JE, Vlachos A, Atsidaftos E et al. Ribosomal protein gene deletions in Diamond-Blackfan anemia. Blood 118(26), 6943–6951 (2011).
- Takahashi K and Yamanaka S. Induction of pluripotent stem cells from mouse embryonic and adult fibroblast cultures by defined factors. Cell 126(4), 663–676 (2006).
- Yoshida Y and Yamanaka S. Recent stem cell advances: induced pluripotent stem cells for disease modeling and stem cell-based regeneration. Circulation 122(1), 80–87 (2010).
- Garcon L, Ge J, Manjunath SH et al. Ribosomal and hematopoietic defects in induced pluripotent stem cells derived from Diamond Blackfan anemia patients. Blood 122(6), 912–921 (2013).
- Kennedy M, Awong G, Sturgeon CM et al. T lymphocyte potential marks the emergence of definitive hematopoietic progenitors in human pluripotent stem cell differentiation cultures. Cell Rep. 2(6), 1722–1735 (2012).
- Gaj T, Gersbach CA Barbas CF 3rd. ZFN, TALEN, and CRISPR/Cas-based methods for genome engineering. Trends Biotechnol. 31(7), 397–405 (2013).
- DeKelver RC, Choi VM, Moehle EA et al. Functional genomics, proteomics, and regulatory DNA analysis in isogenic settings using zinc finger nuclease-driven transgenesis into a safe harbor locus in the human genome. Genome Res. 20(8), 1133–1142 (2010).
- Bellin M, Marchetto MC, Gage FH Mummery CL. Induced pluripotent stem cells: the new patient? Nat. Rev. Mol. Cell. Biol. 13(11), 713–726 (2012).
- Narla A, Dutt S, McAuley JR et al. Dexamethasone and lenalidomide have distinct functional effects on erythropoiesis. Blood 118(8), 2296–2304 (2011).
- Alvarez CV, Garcia-Lavandeira M, Garcia-Rendueles ME et al. Defining stem cell types: understanding the therapeutic potential of ESCs, ASCs, and iPS cells. J. Mol. Endocrinol. 49(2), R89–R111 (2012).
- Ebihara Y, Ma F Tsuji K. Generation of red blood cells from human embryonic/induced pluripotent stem cells for blood transfusion. Int. J. Hematol. 95(6), 610–616 (2012).
- Gekas C Graf T. Induced pluripotent stem cell-derived human platelets: one step closer to the clinic. J. Exp. Med. 207(13), 2781–2784 (2010).
Website
- ClinicalTrials.gov identifier: NCT01464164. http://clinicaltrials.gov/show/NCT01464164