Abstract
Preoperative chemoradiotherapy and radiotherapy with an integrated boost offer excellent local control rates in patients with rectal cancer. The introduction of intensity-modulated radiotherapy and image-guided radiotherapy has drastically improved the tolerance of these treatments. The new challenge is developing organ-preserving strategies and curative treatments for medically inoperable patients. Contact radiotherapy seems efficient for small tumors. Tumor hypoxia limits the success of radiotherapy for locally advanced cancers. Modulation of the L-arginine/iNOS pathway and implementation of hypoxia imaging in radiotherapy planning may overcome this hurdle.
The addition of concomitant 5-fluorouracil (5-FU) or its prodrug capecitabine to preoperative radiotherapy (RT) is the standard of care in patients with locally advanced rectal cancer. According to randomized trials, the combined treatment modality increases the pathologic complete remission rate and local control (LC) over RT alone, but has no impact on survival or the incidence of distant metastases Citation[1,2]. However, this treatment is associated with significant acute and late digestive toxicity, when using 3D-conformal RT. Current strategies mainly aim at improving the outcome by addition of oxaliplatin and biologic agents such as cetuximab. The role of those agents in addition to 5-FU chemoradiotherapy is questionable as so far the results from Phase III trials do not show improvement in pathologic complete remission rate, negative circumferential resection margins, LC or survival, nevertheless an increased toxicity Citation[3–6]. Considering the excellent LC rates in operable rectal cancer in patients, decreasing radiation enteritis should be an absolute priority in our vision.
Intensity-modulated & image-guided radiotherapy
In an attempt to decrease treatment-related toxicity, we introduced the concept of intensity-modulated and image-guided RT (IMRT–IGRT) in the preoperative treatment of rectal cancer. The TomoTherapy Hi-Art II System is a linac that fully integrates IGRT by means of megavolt computed tomography and IMRT by means of helical tomotherapy. A pilot study explored the potential of the integrated megavolt computed tomography in decreasing the clinical target volume–planning target volume (CTV–PTV) margin compared with classic laser skin marks, by measuring the setup error and internal organ motion . The CTV–PTV margin can be reduced from 15 mm isotropically to 8 mm in both lateral, 11 mm in the anterior and 7 mm in the posterior direction Citation[7]. As a next step, we investigated to what extent the integration of IMRT and IGRT can reduce the irradiated volume of small bowel, which is the major predictor of radiation enteritis. To do so, 3D-conformal RT, IMRT (helical tomotherapy) and IMRT–IGRT (helical tomotherapy with reduced CTV–PTV margins) were compared in a dosimetric evaluation. This study demonstrated a synergism between IMRT and IGRT in decreasing the normal tissue complication probability for developing symptomatic diarrhea to 18%, as opposed to a calculated risk of 27 and 40% for IMRT and 3D-conformal RT, respectively Citation[8]. The clinical implementation of preoperative IMRT–IGRT in a Phase II trial in 108 patients with locally advanced rectal cancer confirmed this favorable toxicity profile, with 16 patients developing symptomatic diarrhea and one patient a grade 3 acute toxic effect only Citation[9,10]. This is considerably lower than the 15% acute grade 3+ toxicity reported using 3D-conformal RT with concomitant 5-FU (1,2).
Figure 1. Intensity-modulated and image-guided radiotherapy (IMRT-IGRT) for rectal cancer. (A) Positioning by image-guided radiotherapy (right) improves the positioning accuracy compared with laser skin marks (left). (B) Intensity-modulated radiotherapy allows dose sculpting around the target volume while sparing the small bowel (left), and delivering a simultaneous integrated boost (right).
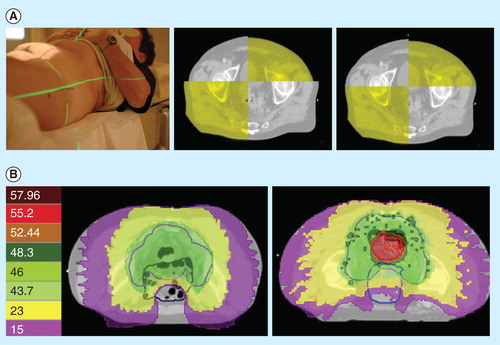
Simultaneous integrated boost
As alternative strategy to the administration of concomitant 5-FU, we decided exploring a simultaneous integrated boost in patients with a circumferential resection margins <2 mm on MRI, until 55.2 Gy or 120% of the prescription dose (46 Gy in daily fractions of 2 Gy, ). With a median follow-up of 54 months, an actuarial 5-year LC of 97% was documented in this population at high risk for local failure Citation[11]. The use of preoperative IMRT–IGRT with a simultaneous integrated boost is currently being compared with standard preoperative chemoRT in a multicenter Phase III trial (NCT 01224392) Citation[12]. The aim of this study is to demonstrate the non-inferiority of a higher radiation dose compared with concomitant chemotherapy, with metabolic response as primary endpoint. A first safety monitoring after inclusion of 114 patients shows a comparable mean decrease in standardized uptake value max (54 vs 50%) and a reduced grade 2 enteritis (25 vs 36%) and grade 3 toxicity (10 vs 4%) in favor of the simultaneous integrated boost arm Citation[13].
Contact radiotherapy
With these excellent results for both LC and toxicity in mind, our research program will focus on organ preserving approaches and treatments for medically inoperable patients. The latter group is continuously increasing with the aging of the population and emerging screening programs. In inoperable patients with cT1 rectal cancer, we propose delivering 90–100 Gy in four fractions by contact radiotherapy. Contact radiotherapy for rectal cancer was developed in the 1950s by Dr. Jean Papillon and is very effective for early rectal cancer with LC rates superior to 85% Citation[14]. However, few centers offer this treatment today, because of a lack of contact x-ray machines and experience. Ariane Medical Company is producing a prototype of a modern contact x-ray machine, the Papillon+ x-ray brachytherapy system (XRB). XRB is delivered by intrarectal applicators with a diameter of 2–3 cm. The limited penetration of 50kV x-rays (50% depth dose at 5–7 mm) allows limiting the ablative dose of radiation to small volumes (1.5–3.5 cc), allowing regeneration of the rectal wall without risk of major complications. For patients with cT2–3 rectal cancer with a diameter of less than 4 cm, we propose a Phase II trial combining 105 Gy XRB in four fractions with CAP50 (capecitabine + 50Gy external beam radiotherapy). Patients with circumferential cT3 and cT4 tumors are not to be considered candidates for XRB and will be treated by 5 × 5 Gy for symptom control or within an observational study that explores the integration of biological tumor signatures in radiotherapy Citation[15].
Biological tumor signatures
The growth and survival of cells in solid tumors is dependent on the adequate supply of oxygen and nutrients, which diffuse from the blood vessels and are consumed by the tumor cells. Besides, oxygen plays an important role in the fixation of radiation-induced DNA damage. When radiation is absorbed in a biological material, free radicals are produced. It is the fate of the free radicals produced in the DNA (DNA•) that determines the biological damage. In the presence of oxygen, DNA-OO• is produced and further processed to DNA-OOH. This results in a changed chemical composition of the DNA and thus fixation of the DNA damage. In the absence of oxygen, DNA• can react with H+, restoring the DNA in its original form. The neovasculature in solid tumors is morphologically and functionally abnormal and is generally unable to meet the increasing demands, resulting in diffusion-limited chronic hypoxia. This picture becomes more complex when considering temporary closures of blood vessels by thrombi and spasms, resulting in perfusion-limited acute hypoxia. Hence, hypoxia is a principal signature of the tumor microenvironment and is considered to be an important cause of clinical radioresistance and local recurrence Citation[16].
Our laboratory demonstrated that endogenous production of nitric oxide (NO) through the inducible isoform of NO synthase (iNOS) by tumor cells, activated macrophages and hepatocytes results in efficient hypoxic tumor cell radiosensitization Citation[17,18]. Another important tumor signature is the immunosuppressive network, which consists of myeloid-derived suppressor cells, Tregs and tumor-associated macrophages. Myeloid-derived suppressor cells are significantly up-regulated in cancer patients, overexpress arginase and induce T-cells anergy, thus resulting in tumor immune escape Citation[19]. As both iNOS and arginase compete for L-arginine, a limiting physiological substrate, we will investigate the role of the L-arginine metabolism in hypoxic radiosensitization and tumor immunity . The L-arginine pathway can be modulated by iNOS indicers such as lipid A and its analog OM-174, myeloid-derived suppressor cells inhibitors and iv administration of L-arginine Citation[17,20]. These preclinical findings have still to be translated to the clinic.
Hypoxia imaging
Tumor hypoxia can be evaluated in patients by hypoxia-selective PET tracers, such as 18FMISO Citation[21]. Alternatively, we have a research program in perfusion-weighted MRI and CT imaging Citation[22]. Because tumor hypoxia is induced by poor tumor perfusion, perfusion maps may help determining hypoxic and hence radio-resistant tumor areas. In conventional radiotherapy, a homogenous or uniform dose is generally delivered to the target volume without violating the tolerance of the nearby tissues. However, biologically, it makes more sense to prescribe non-uniform target dose distributions. Models suggest that a significant increase in tumor control probability might be gained from partial tumor boost doses, if they are delivered to 60% or more of the tumor volume Citation[15]. We will clinically explore this concept by delivering a partial tumor boost on poorly perfused tumor regions. Considering the dynamic nature of tumor hypoxia and reoxygenation during fractionated radiotherapy, it seems advisable to regularly re-determine the partial boost volume during a 5- to 6-week fractionated radiotherapy schedule. In this regard, repetitive computed tomography imaging may offer an advantage over PET scans, with regard to patient comfort.
However, the resolution of tumor hypoxia may be a major obstacle for this approach. Indeed, diffusion-limited chronic hypoxia induces hypoxic rims at 70–100 μm from the tumor vessels. This phenomenon cannot be visualized and treated by current imaging and radiotherapy modalities. We will explore an alternative strategy by implementing hypoxia-specific imaging modalities in tumor control probability–normal tissue complication probability models for patient individualized dose escalation protocols.
Conclusion
Preoperative chemoradiotherapy and radiotherapy with an integrated boost offer excellent LC rates (>95% at 5 years) in patients with rectal cancer. The introduction of IMRT and IGRT has drastically improved the tolerance of these treatments. The new challenge is developing organ preserving strategies and curative treatments for medically inoperable patients. Contact radiotherapy seems efficient for small tumors. Tumor hypoxia limits the success of radiotherapy for locally advanced cancers. Modulation of the L-arginine/iNOS pathway and implementation of hypoxia imaging in radiotherapy planning may overcome this hurdle.
Radiotherapy vocabulary
Gross tumor volume (GTV): The gross palpable or visible extent of malignant growth.
Clinical target volume (CTV): A tissue volume that contains the gross tumor volume and subclinical malignant disease, which is to be eliminated.
Planning target volume (PTV): An additional margin added to the CTV to ensure that the prescribed dose is delivered to the CTV, taking into account setup errors, internal organ motion and movement of the patient during treatment.
Conformal radiotherapy: It describes the aim in radiotherapy of conforming or shaping the high-dose volume to the PTV.
Normal-tissue complication probability: With the introduction of 3D treatment planning systems, it has become possible to calculate and evaluate the dose distribution not only in tumors but also in nearby normal tissues. From these 3D dose distributions, it is possible to model the outcome in biological terms of tumor control probability and normal-tissue complication probability. However, proper clinical validation of these models is still lacking.
Intensity-modulated radiotherapy (IMRT): Radiotherapy technique in which the intensity of irradiation varies within a radiation field. IMRT allows sculpting of high radiation doses round the PTV.
Image-guided radiotherapy (IGRT) is defined as frequent imaging in the treatment room that allows treatment decisions to be made on the basis of these images. IGRT aims at decreasing CTV-to-PTV margins from centimeters to millimeters.
Acknowledgements
The authors thank J-P Gerard, Centre Antoine Lacassagne, Nice, France, for kindly providing figure 2.
Financial & competing interests disclosure
The authors were funded by the Anticancer Fund and by the Vlaamse Liga tegen Kanker, Belgium. The authors have no other relevant affiliations or financial involvement with any organization or entity with a financial interest in or financial conflict with the subject matter or materials discussed in the manuscript apart from those disclosed.
Notes
References
- Bosset JF, Collette L, Calais G, et al. Chemotherapy with preoperative radiotherapy in rectal cancer. N Engl J Med 2006;355:1114-23
- Gérard JP, Conroy T, Bonnetain F, et al. Preoperative radiotherapy with or without concurrent fluorouracil and leucovorin in T3-4 rectal cancers: results of the FFCD 9203. J Clin Oncol 2006;24:4620-5
- Aschele C, Cionini L, Lonardi S, et al. Primary tumor response to preoperative chemoradiation with or without oxaliplatin in locally advanced rectal cancer: pathologic results of the START-01 randomized phase III trial. J Clin Oncol 2011;29:2773-80
- Dewdney A, Cunningham D, Tabernero J, et al. EXPERT-C: A randomized phase II European multicenter trial of neoadjuvant chemotherapy (capecitabine/oxaliplatin) and chemoradiation (CRT) with or without cetuximab followed by total mesorectal excision (TME) in patients with MRI-defined high-risk rectal cancer. J Clin Oncol 2011;29(Suppl 4); abstr 360
- Gérard JP, Azria D, Gourgou-Bourgade S, et al. Comparison of two neoadjuvant chemoradiotherapy regimens for locally advanced rectal cancer: results of the phase II trial ACCORD12/0405)-Prodige 2. J Clin Oncol 2010;28:1638-44
- Roh M.S, et al. The impact of capecitabine and oxaliplatin in the preoperative multimodality treatment in patients with carcinoma of the rectum: NSABP R-04. J Clin Oncol 2011;29(Suppl 4; abstr 3503
- Tournel K, De Ridder M, Engels B, et al. Assessment of intrafractional movement and internal motion in radiotherapy of rectal cancer using megavoltage computed tomography. Int J Radiat Oncol Biol Phys 2008;71:934-9
- Engels B, De Ridder M, Tournel K, et al. Preoperative helical tomotherapy and megavoltage computed tomography for rectal cancer: impact on the irradiated volume of small bowel. Int J Radiat Oncol Biol Phys 2009;74:1476-80
- De Ridder M, Tournel K, Van Nieuwenhove Y, et al. Phase II study of preoperative helical tomotherapy for rectal cancer. Int J Radiat Oncol Biol Phys 2008;70:728-34
- Engels B, Tournel K, Everaert H, et al. Phase II study of preoperative helical tomotherapy with a simultaneous integrated boost for rectal cancer. Int J Radiat Oncol Biol Phys 2012;83(1):142-8
- Engels B, Platteaux N, Van den Begin R, et al. Preoperative intensity-modulated and image-guided radiotherapy with a simultaneous integrated boost in locally advanced rectal cancer: report on late toxicity and outcome. Radiother Oncol 2014;110(1):155-9
- Engels B, De Ridder M. Clinical implementation of intensitymodulated and image-guided radiotherapy in colorectal cancer. Belg J Med Oncol 2012;6:70-2
- Engels B, De Paoli A, Cattari G, et al. Preoperative radiotherapy with a simultaneous integrated boost compared to chemoradiotherapy for T3-4 rectal cancer: interim analysis of a multicentric randomized trial. 56th ASTRO Annual Meeting, San Francisco 2014
- Gérard JP, Ortholan C, Benezery K, et al. Contact X-ray therapy for rectal cancer: experience in Centre Antoine-Lacassagne, Nice, 2002-2006. Int J Radiat Oncol Biol Phys 2008;72(3):665-70
- Verellen D, De Ridder M, Linthout N, et al. Innovations in image-guided radiotherapy. Nat Rev Cancer 2007;7(12):949-60
- De Ridder M, Van Esch G, Engels B, et al. Hypoxic tumor cell radiosensitization: role of the iNOS/NO pathway. Nitric Oxide-Biology and Chemistry 2008;19(2):164-9
- De Ridder M, Verovski V, Darville MI, et al. Macrophages enhance the radiosensitizing activity of lipid A: a novel role for immune cells in tumor cell radioresponse. Int J Radiat Oncol Biol Phys 2004;60(2):598-606
- Jiang H, Verovski VN, Leonard W, et al. Hepatocytes determine the hypoxic microenvironment and radiosensitivity of colorectal cancer cells through production of nitric oxide that targets mitochondrial respiration. Int J Radiat Oncol Biol Phys 2013;85(3):820-7
- Raber P, Ochoa A, Rodríguez PC. Metabolism of L-arginine by myeloid-derived suppressor cells in cancer: mechanisms of T cell suppression and therapeutic perspectives. Immunol Invest 2012;41(6-7):614-34
- De Ridder M, Verovski V, Chiavaroli C, et al. The radiosensitizing effect of immunoadjuvant OM-174 requires cooperation between immune and tumor cells through interferon-gamma and inducible nitric oxide synthase. Int J Radiat Oncol Biol Phys 2006;66(5):1473-80
- Bittner MI, Wiedenmann N, Bucher S, et al. Exploratory geographical analysis of hypoxic subvolumes using 18F-MISO-PET imaging in patients with head and neck cancer in the course of primary chemoradiotherapy. Radiother Oncol 2013;108(3):511-16
- Makkat S, Luypaert R, De Mey J. Deconvolution-based dynamic contrast-enhanced MR imaging of breast tumors: correlation of tumor blood flow with human epidermal growth factor receptor 2 status and clinicopathologic findings - preliminary results. Radiology 2008;249(2):471-82