Emerging studies indicate that, in addition to drug accumulation, subtherapeutic antibiotic concentrations may affect the outcome of therapy during renal replacement therapy (RRT) in the critically ill [Citation1,Citation2]. While dosing appropriateness for some drugs could be gauged by objective outcome measures, the lack of a clear ‘end-of-needle’ effect for antibiotics has made it difficult to ascertain dosing adequacy at the bedside. The preferred means of measuring adequacy of antibiotic dosing regimens is through characterization of the relationship between the plasma drug concentration profile, in vitro antibacterial activity (minimum inhibitory concentration [MIC]), and clinical outcomes. Specifically, the time that the free-drug concentration remains above the MIC, and the ratio of area under the concentration–time curve or the maximum plasma concentration (Cmax) to MIC are the pharmacokinetic/pharmacodynamic (PK/PD) ratios that are best used to describe dosing adequacy for different antibiotics. Current dosing practices may fail to attain target ratios, due to a complex set of factors that alter the PK/PD of antibiotics [Citation2].
Initial dosing adequacy may be altered by disease-related changes in the volume of distribution, whereas the maintenance dose may be significantly affected by extracorporeal RRT clearance. For those antibiotics significantly eliminated via renal excretion (i.e. >25–30%), extracorporeal clearance may constitute a substantial proportion of the overall drug clearance, particularly during continuous renal replacement therapy (CRRT) [Citation1,Citation2]. However, it can be extremely challenging to predict drug clearance during RRT because of a complex matrix of factors, including RRT-related factors, pathophysiology, residual renal clearance, and the presence/alteration of nonrenal clearance pathways.
Different modalities of RRT such as prolonged intermittent RRT either in the form of sustained low-efficiency dialysis (SLED) or diafiltration (SLED-f) and also CRRT in the form of hemofiltration, hemodialysis or hemodiafiltration are used in intensive care units (ICUs). The difference in the intensity of therapy, mechanisms of filtration, surface area, and type of filter in these different modalities gives rise to different RRT drug clearances [Citation3]. For some antibiotics (e.g. amikacin), adsorption to certain filter types could be a significant means of elimination [Citation4]. Importantly, variable operational settings are used across different ICUs even for the same RRT modality and filter conditions. Particularly, the blood and effluent flow rates can significantly affect extracorporeal drug removal [Citation5,Citation6]. Another source of variability is the location of replacement fluid administration, where pre-filter dilution may lead to reduced clearance while post-filter administration accelerates clearance. Even if RRT modalities and operational settings are standardized, accurate prediction of clearance in critically ill patients is difficult, not only due to patient-specific PK variability, but also due to the inconsistent relationship between RRT-related factors and the extent of extracorporeal clearance. For instance, Roberts et al. [Citation1] showed that the effect of prescribed CRRT effluent flow rate on vancomycin clearance was not consistently dose-dependent, and a clear relationship of RRT clearance with effluent flow rate is not observed for all antibiotics. Further to this, a correlation between intensity of CRRT and dosing could be confounded by unintended interruption of CRRT due to blood clotting or when patients have to attend to surgery or other procedures. In such circumstances, the rebound antibiotic concentrations (drug redistributing from tissues to blood) and subsequent maintenance doses are hard to predict.
In addition to the extracorporeal clearance, the residual renal clearance is a variable that contributes toward the total clearance and may be hard to estimate. Furthermore, upregulation of none renal clearance pathways, such as biliary route of elimination, may add another layer of dosing complexity for some antibiotics.
The traditional empirical dosing approach is unable to account for the factors described above. It is not uncommon to see similar dosing regimens being used even in a particular patient when the RRT settings are modified during the course of treatment, without any consideration for the consequence of these changes on the effectiveness of the antibiotic therapy [Citation7]. Although dosing may be based, at times, on RRT-specific recommendations in the product information of some drugs, these are often based on PK studies in patients with chronic kidney disease receiving intermittent hemodialysis. Pharmacokinetically, such stable patients may behave differently as compared to those in the ICU; for example, such patients are unlikely to exhibit an elevated volume of distribution of the antibiotic and associated initial subtherapeutic exposure after initial dosing. Unfortunately, there is limited PK data in ICU patients, particularly for modalities that have emerged over the last one to two decades, such as SLED techniques. Thus, the current dosing approach in critically ill patients receiving RRT is largely unsupported by evidence.
Consequently, contemporary antibiotic dosing regimens used in RRT frequently give rise to inappropriate antibiotic exposure [Citation8]. Because an empirical dosing regimen primarily aims to avoid drug accumulation, a common mistake could be unnecessary dose reductions which then lead to subtherapeutic concentrations. This is particularly true for drugs that are predominantly eliminated via kidney, because these are more likely to have substantial RRT clearance. Indeed, a number of clinical studies have demonstrated highly variable antibiotic PK and the possibility of underexposure with conventional dosing regimens [Citation1]. This may result not only in treatment failure but also in the emergence of resistant pathogens; there are reports of selection of drug-resistant strains during empiric therapy in patients receiving CRRT [Citation9].
Suboptimal antibiotic therapy can heighten an already high risk of mortality for patient with acute kidney injury receiving RRT. Thus, optimization of antibiotic therapy should be a key strategy to reduce the risk of mortality. However, although the ongoing efforts to characterize the PK of antibiotics and dosing needs of patients receiving RRT are encouraging, yet a well-established, evidence-based dosing strategy is lacking. For those antibiotics for which PK data during RRT is accumulating, knowledge of relationships of different RRT settings and disease factors that alter PK parameters may be synthesized into a dosing algorithm to aid clinical decision-making. The utility of such an approach for dosing individualization, using a simplified noncompartmental PK analysis, has been described by Choi et al. [Citation10]. However, this approach fails to incorporate PK variability and thus may not ensure appropriate dose prediction. Accurate dose prediction requires the prescriber to account for between-patient PK variability. In addition, the patients’ condition in ICU may spontaneously change during the course of therapy leading to altered PK as well. This heightens the challenge of optimizing antibiotic dosing, necessitating a robust dose individualization tool.
An attractive approach to account for the significant PK variability is the application of population PK analysis. This enables development of mathematical models that describe PK variability in terms of most relevant RRT-related and clinical covariates. The more precise estimation of PK parameters enables a more precise prediction of dosing requirements [Citation11,Citation12]. However, even the few available population PK models do not account for the full range of covariates and would require standardization in terms of RRT modalities and operational settings to ensure wide application. Unfortunately, this is challenging to do so, given the heterogeneity of patients and hence their respective RRT needs; for instance, some patents may need high CRRT dose to manage electrolyte/acid–base disorders [Citation13]. An additional limitation of dose optimization only based on population PK models/covariates is that clinicians still cannot confirm if the newly adopted doses give rise to appropriate exposure. At the moment, appropriateness of dosing can only be ascertained by measuring plasma drug concentration coupled with an analysis PK/PD target attainment [Citation14]. This would also be important given the frequency of less susceptible bacteria in ICU settings, which requires dosing to be titrated not only based on PK but also based on the altered PD.
Thus, a more rational approach to dosing antibiotics during RRT in ICU patients could be giving an aggressive initial dose (e.g. a loading dose of 150% of usual dose) to avoid low concentrations associated with expansion of the volume of distribution, and subsequently performing therapeutic drug monitoring (TDM)–guided adjustments of the maintenance dose. These loading doses are particularly important for hydrophilic drugs. Unfortunately, there are practical challenges for the wide clinical application of TDM. Primarily, rapid antibiotic concentration measurement is not routinely available for many drugs. Also, dosing adjustment strategies that reference TDM concentrations have not been validated, although traditionally this involves empirical adjustments. A more robust dose individualization based on TDM concentrations and clinical covariates is possible through Bayesian estimation using population PK models. However, clinical studies are limited in this regard, with only a few antibiotics have population PK models available for different RRT modalities.
All in all, despite the substantial clinical evidence that the current antibiotic dosing practice during RRT frequently results in inappropriate antibiotic exposure, validated and robust dosing methodologies are not available for use by clinicians. Given the very high mortality rate of infected patients on RRT, the ongoing effort to devise rational dosing strategies for these patients should be intensified. A successful design and validation of dosing methodology would require a systematic, stepwise study that integrates different approaches. Until that time, use of dosing recommendations based on published RRT PK studies supported by TDM is suggested to maximize the likelihood of achieving therapeutic concentrations.
Financial and competing interests disclosure
JA Roberts is a recipient of an Australian National Health and Medical Research Council Fellowship (APP1048652). We wish to acknowledge funding from the Australian National Health and Medical Research Council for Project Grants (APP1044941, APP1062040) and Centre of Research Excellence (APP1099452). The authors have no other relevant affiliations or financial involvement with any organization or entity with a financial interest in or financial conflict with the subject matter or materials discussed in the manuscript apart from those disclosed.
Additional information
Notes on contributors

Fekade Bruck Sime
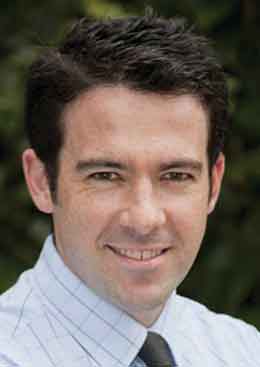
Jason A. Roberts
References
- Roberts DM, Liu X, Roberts JA, et al. A multicenter study on the effect of continuous hemodiafiltration intensity on antibiotic pharmacokinetics. Crit Care. 2015;19:84.
- Jamal JA, Mueller BA, Choi GY, et al. How can we ensure effective antibiotic dosing in critically ill patients receiving different types of renal replacement therapy? Diagn Microbiol Infect Dis. 2015;82(1):92–103.
- Roger C, Muller L, Wallis SC, et al. Population pharmacokinetics of linezolid in critically ill patients on renal replacement therapy: comparison of equal doses in continuous venovenous haemofiltration and continuous venovenous haemodiafiltration. J Antimicrob Chemother. 2015. pii: dkv349. [Epub ahead of print].
- Tian Q, Gomersall CD, Ip M, et al. Adsorption of amikacin, a significant mechanism of elimination by hemofiltration. Antimicrob Agents Ch. 2008;52(3):1009–1013.
- Jamal JA, Udy AA, Lipman J, et al. The impact of variation in renal replacement therapy settings on piperacillin, meropenem, and vancomycin drug clearance in the critically ill: an analysis of published literature and dosing regimens. Crit Care Med. 2014;42(7):1640–1650.
- Roberts DM, Roberts JA, Roberts MS, et al. Variability of antibiotic concentrations in critically ill patients receiving continuous renal replacement therapy: a multicentre pharmacokinetic study. Crit Care Med. 2012;40(5):1523–1528.
- Jiang SP, Zhu ZY, Wu XL, et al. Effectiveness of pharmacist dosing adjustment for critically ill patients receiving continuous renal replacement therapy: a comparative study. Ther Clin Risk Manag. 2014;10:405–412.
- Harris LE, Reaves AB, Krauss AG, et al. Evaluation of antibiotic prescribing patterns in patients receiving sustained low-efficiency dialysis: opportunities for pharmacists. Int J Pharm Pract. 2013;21(1):55–61.
- Akers KS, Rowan MP, Niece KL, et al. Colistin pharmacokinetics in burn patients during continuous venovenous hemofiltration. Antimicrob Agents Chemother. 2015;59(1):46–52.
- Choi G, Gomersall CD, Tian Q, et al. Principles of antibacterial dosing in continuous renal replacement therapy. Crit Care Med. 2009;37(7):2268–2282.
- Roberts JA, Udy AA, Bulitta JB, et al. Doripenem population pharmacokinetics and dosing requirements for critically ill patients receiving continuous venovenous haemodiafiltration. J Antimicrob Chemother. 2014;69(9):2508–2516.
- Loo AS, Neely M, Anderson EJ, et al. Pharmacodynamic target attainment for various ceftazidime dosing schemes in high-flux hemodialysis. Antimicrob Agents Chemother. 2013;57(12):5854–5859.
- Freda BJ. Dosing of continuous renal replacement therapy in critically ill patients with acute kidney injury: how low should we go?. Crit Care Med. 2013;41(11):2655–2657.
- Chow KM, Li PK. Acute kidney injury: antibiotic therapy during CRRT–getting the dose just right. Nat Rev Nephrol. 2014;10(9):486–488.