Abstract
In this article, we review some basic aspects of imaging for neuro-ophthalmic and orbital diseases in children and summarize the recent literature on newer imaging modalities. First, we discuss the potential dangers related to the overuse of computed tomography scan due to radiation exposure and cancer risks, as this topic has been of concern to scientists and the general public recently. We then briefly review emerging MRI techniques that are of interest in neuro-ophthalmology.
Medscape: Continuing Medical Education Online
This activity has been planned and implemented in accordance with the Essential Areas and policies of the Accreditation Council for Continuing Medical Education through the joint sponsorship of Medscape, LLC and Expert Reviews Ltd. Medscape, LLC is accredited by the ACCME to provide continuing medical education for physicians. Medscape, LLC designates this educational activity for a maximum of 1.0 AMA PRA Category 1 Credits™. Physicians should only claim credit commensurate with the extent of their participation in the activity. All other clinicians completing this activity will be issued a certificate of participation. To participate in this journal CME activity: (1) review the learning objectives and author disclosures; (2) study the education content; (3) take the post-test and/or complete the evaluation at www.medscapecme.com/journal/expertophth; (4) view/print certificate.
Learning objectives
Upon completion of this activity, participants should be able to:
• Identify strategies to protect children from radiation exposure associated with orbital CT imaging
• Describe advantages and shortcomings of orbital MRI in children, including newer techniques
• Describe characteristic MRI findings associated with specific orbital lesions in children
Financial & competing interests disclosure
Editor
Elisa Manzotti, Editorial Director, Future Science Group, London, UK.
Disclosure:Elisa Manzotti has disclosed no relevant financial relationships.
CME Author
Laurie Barclay, MD
Freelance writer and reviewer, Medscape, LLC
Disclosure: Laurie Barclay has disclosed no relevant financial relationships.
Authors and Credentials
Angel Sanchez-Montanez Garcia-Carpintero, MD
Department of Radiology, University of North Carolina Hospital, Old Clinic room 2010 CB7510, Chapel Hill, 27599-7510 USA and Coorporacio Sanitaria Parc Tauli, UDIAT-CD, Parc Tauli s/n, Sabadell, Barcelona 08208, Spain.
Disclosure:Angel Sanchez-Montanez Garcia-Carpintero has disclosed no relevant financial relationships.
Sasitorn Petcharunpaisan, MD
Department of Radiology, University of North Carolina Hospital, Old Clinic room 2010 CB7510, Chapel Hill, 27599-7510 USA and King Chulalongkorn Memorial Hospital, 1873 Rama IV Road, Pathumwan, Bangkok, 10330, Thailand.
Disclosure:Sasitorn Petcharunpaisan is supported by the King Chulalongkorn Memorial Hospital, Thai Red Cross Society.
Joana Pinto Revez Silva Neves Pedrosa Ramalho, MD
Department of Radiology, University of North Carolina Hospital, Old Clinic room 2010 CB7510, Chapel Hill, 27599-7510 USA and Centro Hospitalar de Lisboa Central, EPE, Rua jose Antonio Serrano, Lisboa, 1150-199, Portugal.
Disclosure:Joana Pinto Revez Silva Neves Pedrosa Ramalho is supported by the Centro Hospitalar de Lisboa central EPE, Calouste Gulbenkian Foundation and Luso-American Foundation.
Mauricio Castillo, MD, FACR
Division of Neuroradiology, University of North Carolina School of Medicine, Room 3326 Old Infirmary Building, Manning Dr. Chapel Hill, NC 27599, USA.
Disclosure:Mauricio Castillo is Editor in Chief of the American Journal of Neuroradiology.
(A) Shows a child just before CT scanning with eyeglasses covered with a bismuth shield (white arrow) that protects from irradiation. (B) Axial CT soft tissue window at the level of the orbits demonstrate the bismuth shield with no underlying artifact in the image obtained.
Reprinted with permission from Elida Vazquez, MD, Hospital Materno-Infantil Vall d’Hebron, Barcelona, Spain.
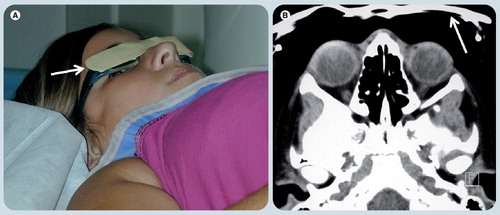
(A) Coronal T1-weighted image and (B) T2-weighted image with fat suppression show a right orbital floor fracture (arrow) with prolapsed orbital fat into adjacent right maxillary sinus.
Reprinted with permission from Citation[13].
![Figure 2. A 9-year-old boy with right orbital floor fracture.(A) Coronal T1-weighted image and (B) T2-weighted image with fat suppression show a right orbital floor fracture (arrow) with prolapsed orbital fat into adjacent right maxillary sinus.Reprinted with permission from Citation[13].](/cms/asset/d2b59b21-0594-4207-bd7a-00c4c1da0ed5/ierl_a_11211385_f0002_b.jpg)
(A & C) Axial gradient echo T2* weighted images show dark signal intensity of ocular calcification corresponding with (B & D) hyperattenuated calcified spots on CT scans in the same patient.
Reprinted with permission from Citation[7].
![Figure 3. A case of right orbital retinoblastoma.(A & C) Axial gradient echo T2* weighted images show dark signal intensity of ocular calcification corresponding with (B & D) hyperattenuated calcified spots on CT scans in the same patient.Reprinted with permission from Citation[7].](/cms/asset/64db6243-2d19-4ff9-affb-c2094d02ef3a/ierl_a_11211385_f0003_b.jpg)
(A) Axial T1 weighted image shows a well-defined area of isointense signal (thick arrow) with focal mass effect and globe distortion medially (thin arrow), and a subtle area of internal high signal intensity (*) that suggests the lesion is complex. (B) Axial diffusion-weighted image shows markedly hyperintense signal (arrow), confirming area of focal abscess. (C) Axial T1-weighted contrast-enhanced image shows an ovoid area of nonenhancement (arrow) corresponding with area of restriction diffusion and representing pus.
Reprinted with permission from Citation[16].
![Figure 4. An 11-year-old girl with orbital cellulitis and extraconal abscess.(A) Axial T1 weighted image shows a well-defined area of isointense signal (thick arrow) with focal mass effect and globe distortion medially (thin arrow), and a subtle area of internal high signal intensity (*) that suggests the lesion is complex. (B) Axial diffusion-weighted image shows markedly hyperintense signal (arrow), confirming area of focal abscess. (C) Axial T1-weighted contrast-enhanced image shows an ovoid area of nonenhancement (arrow) corresponding with area of restriction diffusion and representing pus.Reprinted with permission from Citation[16].](/cms/asset/706cf2de-4bf1-4c81-8180-23dfc3d0a879/ierl_a_11211385_f0004_b.jpg)
(A) Diffusion-weighted imaging and (B) apparent diffusion coefficient map shows marked restricted diffusion (arrow) in the posterior portion of the right optic nerve when compared with the normal anterior aspect (arrowhead) of the nerve.
Reprinted with permission from Citation[18].
![Figure 5. A case of rhinocerebral mucormycosis with acute right optic nerve infarction.(A) Diffusion-weighted imaging and (B) apparent diffusion coefficient map shows marked restricted diffusion (arrow) in the posterior portion of the right optic nerve when compared with the normal anterior aspect (arrowhead) of the nerve.Reprinted with permission from Citation[18].](/cms/asset/f060b199-23c8-4dc5-8821-f9834689d849/ierl_a_11211385_f0005_b.jpg)
(A) Coronal T2-weighted image and (B) postcontrast T1-weighted image shows a well defined nonenhancing mass in the chiasm (arrows). (C) Single voxel MR spectroscopy placed on this lesion shows increased Cho peak and decreased NAA peak, implying high cellularity nature of the glioma. A small Lac is typical of pilocytic gliomas, which use glucose exclusively as their source of energy.
Cho: Choline; Lac: Lactate; NAA: N-acetyl aspartate; Or: .
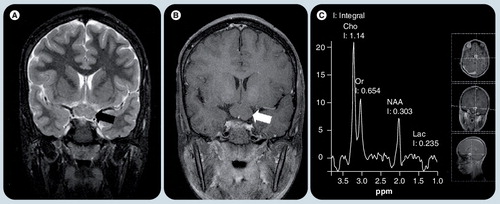
(A) Axial postcontrast soft tissue window CT image at the level of the orbits demonstrates diffuse thickening of soft tissues in the left preseptal region (white arrowheads). The postseptal region is normal. These signs together with the classical clinical history are diagnostic for preseptal cellulitis. (B) Axial postcontrast soft tissue window CT image in a different patient shows the diffuse thickening of soft tissues in left preseptal region (white arrowheads) extending to sclera, which enhances and is also thickened. A fusiform fluid collection (white asterisk) is depicted in the extraconal space, between the medial rectus muscle and the lamina papiracea with an air bubble (white arrow). The findings are compatible with an abscess. There is fluid in the ipsilateral ethmoid cells compatible with sinusitis (black arrow), which is the likely origin of the abscess.
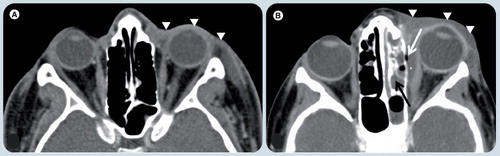
(A) Coronal noncontrast soft tissue window CT image, (B) T2-weighted image (WI), (C) T1-WI and (D) T1-weighted fat-suppressed postgadolinium MR images. There is enlargement of the superior, medial and inferior rectus muscles (white arrowheads) more prominent in the superior one which shows an area of (B) T2 hyperintensity and (C) T1-WI isointensity relative to signal intensity of muscle and a poorly-defined area (white arrows) in its center. The ‘dirty fat’ sign is secondary to inflammatory changes in the intraconal fat (white asterisk). The optic nerve (black arrowheads) is shifted, but otherwise normal. The left orbit is unremarkable.
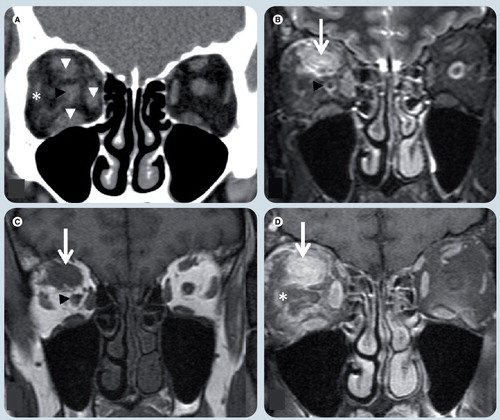
(A) Axial T2-weighted, (B) axial T1-weighted fat-suppressed postgadolinium, (C) coronal T1-weighted, and coronal T1-weighted fat-supressed postgadolinium (D) MRI at level of optic nerves. Symmetrical enlargement of both optic nerves with homogeneous contrast enhancement is seen (white arrows). The optic chiasm (black arrowhead) and the rest of the optic pathways are spared.
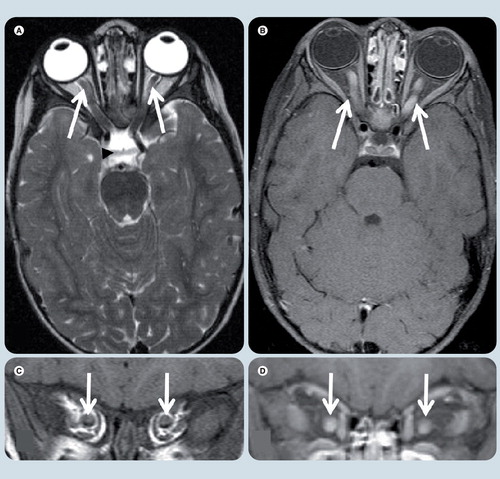
(A) Coronal T2-weighted image (WI), (B) T1-WI, (C) T1-WI fat-suppressed postgadolinium, and (D) axial T1-WI fat-suppressed postgadolinium MRI at level of the optic nerves. An intraconal, left orbital well-defined oval tumor (white arrows), which is (A) T2 hyperintense and (B) isointense on T1-WI relative to the optic nerve is present and (C & D) has avid contrast enhancement. (D) The optic nerve (black arrow) is seen within the mass and does not enhance, giving the typical ‘tramtrack’ sign on axial image.
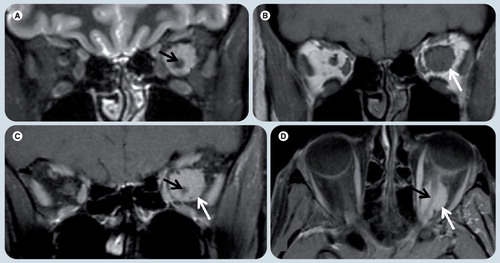
(A) Coronal T2-weighted, (B) axial T1-weighted fat-supressed postgadolinium, (C) axial FLAIR, and (D) axial T2-weighted MRI demonstrate a heterogeneous mass in the posterior and inferior right globe (white arrows). (A & D) The lesion is T2 hypointense with respect to the vitreous. (B) The tumor has marked enhancement after contrast administration. (C) On a FLAIR image the mass has heterogeneous signal intensity and the vitreous shows abnormal signal intensity (white asterisk), likely due to protein exudate. There are small foci of hyposignal in the mass (white arrowhead in (C)) that represent speckle calcifications.
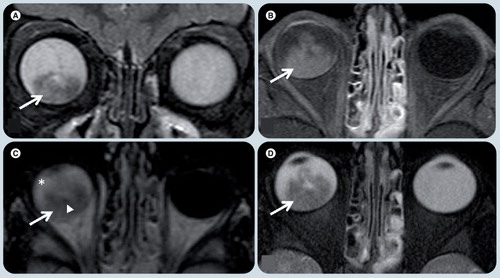
Axial T2-weighted image (WI) (A), axial T1-WI fat-supressed post-gadolinium (B), diffusion weighted (C), and apparent diffusion coefficient map (D) MR images. A small single lesion (black arrow) is seen in the posterior and medial-center aspect of the left globe. It has low signal on T2-WI (A) relative to the vitreous and avid enhancement in postcontrast image (B). On diffusion WIs there is restricted diffusion in the mass (arrows) (C & D).
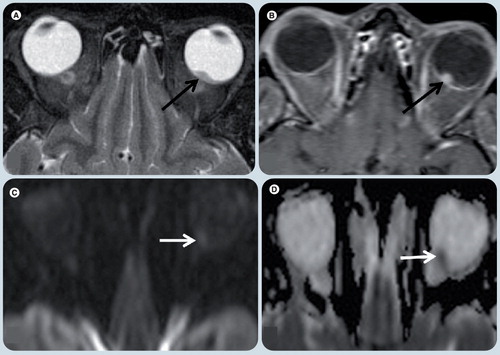
(A) Axial T2-weighted image (WI), (B) T1-weighted and (C) T1-weighted fat-supressed postgadolinium MRI show a small lesion in the posterior and lateral aspect of the left globe (black arrow). (A) The lesion is hypointense on T2-WI and (B) hyperintense on T1-WI relative to the vitreous and (C) shows avid enhancement after contrast administration. There were no other lesions. Patient’s history of prior primary lung carcinoma suggested the correct diagnosis.
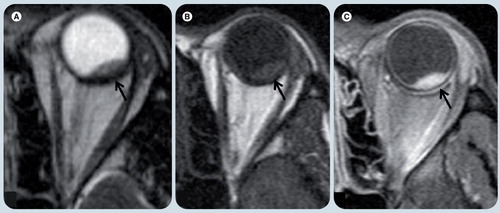
(A) Axial postcontrast soft-tissue window CT image, (B) axial T2-weighted, (C) coronal T1-weighted image (WI), and (D) coronal T1-weighted fat-suppressed postgadolinium MR images demonstrate a poorly circumscribed and heterogeneous lesion (white arrows) in the right orbit involving several compartments. (B) The tumor is hyperintense on T2-WI and (C) isointense on T1-WI relative to the muscles. Intense enhancement is depicted in both (A) CT and (D) MRI. There are flow-voids (white arrowheads) corresponding to blood vessels. The lesion is causing expansion of the bony orbit suggesting slow growth, chronicity and proptosis.
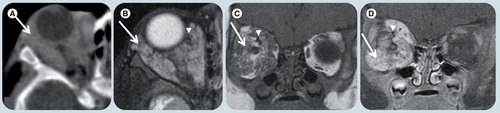
Is computed tomography useful in pediatric orbital imaging?
Computed tomography (CT) is one of the most valuable orbital imaging modalities and its use in adults and children has increased approximately tenfold in the last 20 years Citation[1]. It is estimated that more than 62 million CT studies/year are currently carried out in the USA, including at least 4 million in children Citation[2]. Approximately 33% of pediatric CTs are performed in children in the first decade of life, with 17% of them at or under the age of 5 years Citation[3].
A major concern with CT is the risk of developing cancer from radiation exposure, especially in children. Considerations unique to children include: increased radiosensitivity of certain tissues, a longer lifetime for cancers to occur, and a lack of size-based adjustments in the technique Citation[3]. As a result, the risk for developing radiation-related cancers is several times higher for children compared with adults exposed to identical CT doses. Patients undergoing a brain CT scan typically receive organ doses between 5 and 10 mGy. Therefore, organ dose in CT is below the threshold for induction of skin lesions or cataracts. The principal concern at those low doses is induction of fatal and nonfatal cancers. This concern stems in part from data published as part of a study of atomic bomb survivors Citation[4]. The radiation dose that some Japanese survivors were exposed to is similar to that from CT. The cancer risk appears to be linear in relation to the dose and is highest in those exposed as children Citation[1,4]. Although the form of radiation delivered by the atomic bomb (γ-radiation) is different from x-rays, there is no difference in their carcinogenic potential Citation[5].
Recent studies show that 600,000 abdominal and head CT scans performed annually in children under the age of 15 years could result in 500 deaths from cancer attributable to radiation Citation[5]. The most common malignancies associated with radiation are breast, thyroid, lung and stomach cancers and leukemia. The latency period for solid cancers is generally 10–20 years and persists throughout life. Of all malignancies, leukemia has the shortest latency period, with increased risk noted as early as 2–5 years following radiation exposure Citation[5].
The first step to minimize radiation exposure in children is to reduce the number of unnecessary procedures. It is estimated that one-third of CTs performed in children may not be pertinent to diagnosis or management Citation[1]. Neuroimaging modalities without radiation such as MRI or sonography/ultrasound (US) may be considered as options. US should be considered an adjunctive test, rather than a primary means of orbital imaging, owing to its limited resolution and depth of penetration. MRI avoids the risk of radiation and provides superior soft tissue contrast. Gadolinium (Gd)-based contrast agents used in MRI may be safer than iodine-based CT contrast Citation[5]. MRI in children frequently requires sedation, and children who are too old for oral chloral hydrate need anesthesia. Pediatric anesthesia carries a risk that is probably lower than the estimated risk of subsequent tumor development associated with CT radiation. MRI is superior to CT for most neuro-ophthalmic indications, but CT is still the first imaging modality for evaluation of bone and trauma. CT is useful if a patient cannot undergo MRI (e.g., severe claustrophobia, marked obesity exceeding the table weight limit, pacemaker, ferromagnetic aneurism clip, cochlear implant or metallic foreign bodies) Citation[6]. Retinoblastoma (Rb) is another lesion for which many still prefer CT. A recent study concluded that the combination of ophthalmologic investigation, US and MRI detects all Rbs Citation[7]. The MRI orbital protocol used in our institution is shown in the appendix.
The diagnostic value of CT when used for specific indications is unquestionable, but it is essential to reduce the radiation dose per procedure following the ‘as low as reasonably achievable’ (ALARA) principle. Adjustments in CT parameters should be conducted based on individual size and/or weight. Another consideration in orbital imaging is the need for at least two orthogonal planes. Because CT scanners obtain very thin axial slices, the quality of reformatted images has improved and it is possible to eliminate direct coronal scans, reducing radiation exposure. Bismuth glasses are a device being tested in Spain when CT is ordered . In addition to the benefit of reduced eye radiation exposure, they do not cause imaging artifacts, which may reduce imaging quality.
New MRI techniques for pediatric imaging
Magnetic resonance imaging is rapidly evolving and continues to develop faster sequences with greater image signal and resolution. Multichannel coils with parallel imaging and improved gradients substantially reduce acquisition time, improve image quality and permit 3D imaging. These techniques are important when imaging children who have small anatomical structures and tend to move Citation[8].
An important improvement in pediatric MRI is the use of 3.0T systems. Benefits of higher field strength include improved resolution, speed and increased signal-to-noise ratio (SNR) compared with 1.5T systems Citation[8]. Parallel imaging is a method that increases imaging speed Citation[8]. It relies on distinct sensitivities of individual elements of receiver coil arrays that allow sampling the k-space more sparsely and quickly Citation[9]. Parallel imaging may be used with all pulse sequences and permits increasing image acquisition speed by a factor of two to three. Development of an increased number of receiver channels provides higher acceleration factors resulting in faster acquisitions Citation[8].
High-resolution MRI with phased-array head coil & surface orbital coil
Orbital surface coils provide high-resolution images with greatest SNR of the anterior optic pathway, but they do not offer adequate evaluation of posterior structures Citation[10]. A phased-array head coil can be used to provide high-quality images of the intracranial structures, not only the posterior optic pathways, but also the cranial nerves and brain stem. Imaging of the entire course of the cranial nerves affected in ocular movement disorders can be easily achieved. The use of phased-array head coils at 3.0T improves SNR, allowing for better images of the orbits with high resolution without the need for surface coils Citation[11]. Microscopic surface coils can be used to produce images of extreme high resolution Citation[11,12]. Kolk et al. used combined head and microscopy surface orbital coils in the diagnosis of pediatric orbital fractures and demonstrated that MRI resulted in excellent visualization of the fractures and adjacent soft tissues Citation[13].
Using 3D gradient echo T2* weighted imaging and 3D turbo spin echo T2-weighted for calcification detection
Computed tomography is superior to MRI to detect calcifications and for detailed study of bone. Gradient echo T2* weighted image (GRE T2* WI) is an MRI sequence sensitive to susceptibility differences among tissues that cause magnetic field inhomogeneity leading to signal loss. This sequence is used to depict blood products and calcifications Citation[14]. GRE T2*W is effective in the detection of calcified structures, typically seen as hypointense regions Citation[7].
Galluzzi et al. assessed MRI in the detection of calcifications in Rb. Their studies were performed with a surface orbital coil using 3D turbo spin echo T2-weighted and 3D T2* gradient-echo images. They demonstrated excellent identification of calcification, and concluded that radiation hazard from CT can be avoided when ophthalmologic findings are used together with MRI in the evaluation of Rb Citation[7].
Diffusion-weighted imaging
Diffusion-weighted imaging (DWI) using echo planar (EPI) sequences provide contrast that is dependent on the molecular motion of water. When measuring molecular motion with DWI, the apparent diffusion coefficient (ADC), a quantification of water diffusion in biologic tissue, can be calculated Citation[15]. DWI has been extensively used for early detection of ischemic stroke, and differentiation of intracranial masses and abscesses Citation[9,15]. Restricted diffusion is seen in cytotoxic edema in acute ischemic stroke Citation[15], hypercellular tumors and hyperviscosity in abscesses and epidermoid cysts Citation[16].
Studies of DWI in head and neck lesions have been published, but there are limited studies employing it in the orbits Citation[16]. Geometric distortions and signal loss in DWI in the orbits are pronounced due to interfaces between bone, soft tissue and air causing an inhomogeneous magnetic field and resulting in susceptibility artifacts Citation[9]. These effects are aggravated at higher field strengths Citation[17]. With the introduction of parallel imaging, blurring and distortion of images are reduced in orbital imaging at high field strengths Citation[9,16,17].
Sepahdari et al. assessed the role of DWI in diagnosing orbital abscess Citation[16]. Orbital abscesses showed restricted diffusion similar to that found in cerebral abscesses. Their study found that DWI improves diagnostic confidence when used in conjunction with contrast-enhanced MRI, and is useful in patients in whom Gd is contraindicated . Kapur et al. investigated the applications of DWI in orbital inflammatory syndrome (OIS) or inflammatory orbital pseudotumor, orbital lymphoid lesions and orbital cellulitis and concluded that DWI helps differentiate OIS from lymphoid lesions and cellulitis Citation[18]. Significant differences in DWI intensities, ADC and ADC ratios between OIS, orbital lymphoid lesions and orbital cellulitis were observed. The study showed that lymphoid lesions were significantly brighter than OIS, and OIS lesions were significantly brighter than cellulitis. Lymphoid lesions showed lower ADC than OIS and cellulitis Citation[19].
There are case reports demonstrating restricted diffusion and low ADC values in optic nerves and retinal infarcts Citation[18,20,21]. DWI aids in the diagnosis of optic nerve infarction when conventional images are normal and may be the sequence that shows earliest abnormality detectable in ischemic optic neuropathy Citation[18].
MRI dacryocystography
Magnetic resonance imaging dacryocystography is a technique used to evaluate patients with epiphora. It is comparable with digital dacryocystography in detection and localization of nasolacrimal system obstruction Citation[22]. MRI dacryocystography has significant advantages over digital and CT dacryocystography in terms of superior soft tissue contrast resolution and lack of ionizing radiation. However, CT dacrocystography remains superior for evaluation of congenital bony stenosis, atresia of the bony segment of the nasolacrimal duct, and detection of intracanicular bony fragments in facial trauma Citation[8]. MRI dacryocystography is performed by topical administration of a diluted mixture of Gd-based contrast agents Citation[22], which provides similar results to catheterization of the lacrimal canaliculi Citation[8]. It may be the best technique for evaluation of the lacrimal system in children.
Diffusion tensor imaging & tactography
By incorporating directionality into DWI, diffusion-tensor imaging (DTI) is obtained. DTI can study tissue microstructure and brain anatomy. Water diffusion in white matter is strongly anisotropic (directional), and occurs maximally in the orientation of white matter tracts Citation[23]. Quantitative information about water diffusion in white matter, including the water’s preferential direction of movement within a voxel (fractional anisotropy [FA]) and its magnitude independent of directionality (mean diffusivity [MD]), can be derived and used to characterize axonal architecture Citation[24].
The optic nerve is surrounded by cerebrospinal fluid and orbital fat, which may cause partial volume effects and chemical-shift artifacts, and thus fluid and fat suppression are necessary. Susceptibility artifacts due to adjacent aerated sinuses and motion artifacts from eye movement further complicate optic nerve DTI Citation[25]. There are several DTI studies of optic nerve pathology in adults related to optic nerve degeneration and neuritis. Decreased FA and increased MD are demonstrated in these diseases Citation[24,26]. DTI may be more sensitive than MRI in detecting abnormalities of the optic nerves Citation[24].
A few DTI studies of optic nerves in pediatric patients have been published. Salmela et al. performed DTI of optic nerves and optic radiations in two pediatric patients with septo-optic dysplasia, and in seven normal children. They showed increased FA with increasing age in both optic nerves and optic radiations. Septo-optic dysplasia patients showed lower FA and higher MD compared with controls in both optic nerves and optic radiations. The authors concluded that DTI may be an effective technique to monitor myelination in septo-optic dysplasia patients, some of whom demonstrated improvement in their vision over time Citation[27].
Diffusion tensor tractography (DTT) is a method that reconstructs and visualizes the 3D structure of white matter tracts Citation[28]. DTT is important because it can delineate white matter tracts for surgical planning. The goal of presurgical DTT is to identify the position of eloquent white matter pathways Citation[29]. DTT may be helpful for surgical planning of lesions near the optic radiations Citation[30]. Salmela et al. demonstrated that DTI and DTT of the optic nerves could differentiate between the suprasellar/chiasmatic tumor and the optic nerves in two pediatric patients Citation[31].
Magnetic resonance spectroscopy
Tumors in pediatric patients that are located at the hypothalamic/chiasmatic/suprasellar region include astrocytoma, craniopharyngioma and germ cell tumor, and visual disturbances are common clinical presentations of these tumors Citation[32,33]. In addition to MRI, magnetic resonance spectroscopy (MRS) may be performed for these lesions. Proton MRS, a US FDA-approved technique, offers a unique, noninvasive approach to assess abnormalities at microscopic levels by quantifying cellular metabolites.
Magnetic resonance spectroscopy of hypothalamic/chiasmatic astrocytoma shows increased choline and diminished N-acetyl aspartate levels, implying high cellularity and neuronal/axonal damage, respectively. The presence of lactate and lipids is seen in aggressive tumors, reflecting anaerobic metabolism and cellular necrosis, respectively. Craniopharyngiomas show dominant lipid peaks. Prominent lipid peaks in hypothalamic germinoma suggest aggressive behavior Citation[34].
Dynamic contrast-enhanced MRI
Dynamic contrast-enhanced MRI (DCE-MRI) is a noninvasive technique used to derive semiquantitative parameters that reflect the microcirculatory structure and function of tissues, including blood volume and vascular permeability, which are inherent to tumor neovasculaturity Citation[35]. Jost et al. found a correlation between DCE-MRI and clinical aggressiveness in optic pathway gliomas (OPGs) in pediatric patients Citation[36]. This study demonstrated that aggressive OPGs had higher mean permeability values than stable tumors. The authors suggested that DCE-MRI might be a useful biomarker for clinically aggressive OPG in pediatric patients Citation[36].
Common developmental anomalies
Dermoid & epidermoid cysts
Dermoid and epidermoid cysts are choristomas Citation[37,38]. Both are common congenital disorders often localized in the anterior superolateral orbit, in the extraconal space, adjacent to the orbit rim at the zygomaticofrontal and frontoethmoidal sutures superomedially. Bone erosion may occur due to slow growth Citation[39–41]. Epidermoid and dermoids differ in that dermoids contain adnexal structures Citation[37]. By CT both are well circumscribed, smoothly marginated, low density, unenhanced masses. If a dermoid contains fat, negative attenuation values are seen. Dermoids may have an enhancing rim that may be partially calcified and contain fluid fat levels. Epidermoids show water density (∼0 Hounsfield units) Citation[37,38–40], and on MRI epidermoids show a similar intensity to water (low T1-weighted image [WI] signal/high T2-WI signal), whereas dermoids signal characteristics are variable depending on their content. When dermoids have a significant fat content, they show high signal on T1- and low signal on T2-WIs. If secretory elements predominate in a dermoid, the lesion may have signal characteristics similar to epidermoids. On DWI, epidermoids are markedly hyperintense and show restricted ADC. Both may demonstrate slight enhancement of their wall on postcontrast examinations Citation[37,38,41].
Optic nerve hypoplasia
Optic nerve hypoplasia is recognized as part of a spectrum of optic nerve, CNS and hypothalamic–pituitary axis congenital anomalies. It can be unilateral or bilateral. MRI is recommended in all patients to rule out associated pathologies such as absence of the septum pellucidum, pituitary stalk, neuronal migration anomalies, and craniofacial abnormalities Citation[38,42,43].
Common infectious & inflammatory diseases
Orbital cellulitis
Orbital and periocular infections are common in children. Periorbital or preseptal infections are more common than orbital cellulitis. The orbital septum provides a barrier to the spread of infection between the periorbital soft tissues (preseptal region) and the orbital space (septal). Preseptal disease is usually secondary to skin pathogens, whereas postseptal cellulitis develops from paranasal sinus illness and is usually caused by Staphylococcus or Streptococcus. Intracranial spread of infection may progress to meningitis, or subdural or parenchymal abscesses Citation[37,38,44,45]. To distinguish these entities, imaging should be ordered and CT is commonly used Citation[45]. In postseptal cellulitis, inflammatory tissues and edema collect beneath the medial periosteum to form a subperiosteal phlegmon, which subsequently develops into an abscess . Abscess formation, a medical and surgical emergency, produces increased intraocular pressure owing to infective subperiosteal material that forms a ring-enhancing lesion that may contain air, displacing the medial rectus muscle. If progression persists, intraconal or extraconal abscess formation may occur and this complication may lead ophthalmic vein and cavernous sinus thrombosis. There may be adjacent osteomyelitis. On MRI, the process shows isosignal intensity on T1- and high signal intensity on T2-WIs relative to muscle. DWI shows restricted diffusion in mature abscesses Citation[37,38,41].
Inflammatory pseudotumor
Orbital pseudotumor or orbital inflammatory syndrome (OIS) is one of the most common causes of intraorbital masses in individuals between 10 and 40 years of age. It is the second most common inflammatory process of the orbits in childhood Citation[37]. OIS is a process with no known local or systemic causes. It is a diagnosis of exclusion based on history, clinical course and response to steroid therapy Citation[38,41,46].
Orbital inflammatory syndrome is usually a unilateral and sometimes bilateral infiltrative process in the orbit fat and adjacent structures. The lacrimal gland is most frequently involved followed by the apex, diffuse disease, extraocular muscles and optic nerves. OIS may spread intracranially to involve the cavernous sinus, infratemporal fossa and nasopharynx via the pterygopalatine fossa. Orbital pseudotumors may be associated with systemic disorders, including Wegener granulomatosis, sarcoidosis, vasculitis and lymphoma Citation[46,47].
Orbital inflammatory syndrome is a common cause of asymmetrical extraocular muscle enlargement with blurred margins. The most commonly affected muscle is the lateral rectus. The tendinous portions of the extraocular muscles are involved, as are the adjacent soft tissues in the intraconal or extraconal spaces. Fat appears infiltrated and streaky. MRI is better than other imaging procedures in showing a focal or diffuse infiltrative soft tissue mass that is hypointense to muscle on T1-WI, iso- or hypointense on T2-WIs, and that enhances after contrast injection. CT scan and US provide effective guidance for performing fine-needle aspirations Citation[37,38,40,41,46].
Optic neuritis
Optic neuritis in childhood is less frequent than in adults, has no gender preference, and is typically bilateral and anterior. Its cause in children is frequently unknown; the most plausible reasons being a demyelination process, viral illness or postimmunization. Its association with multiple sclerosis is not as strong as it is in adults Citation[41,42,47,48].
Magnetic resonance imaging is the most sensitive procedure for evaluating this entity. It shows diffuse swelling and enlargement of the optic nerve, with high T2-WI signal and localized or diffuse enhancing regions on postcontrast fat-suppressed T1-WI Citation[41,43,48,49].
Thyroid orbitopathy
Thyroid eye disease is the most common cause of unilateral and bilateral proptosis in adults (usually middle-aged women) as it enlarges the extraocular muscles. In children it is less common. There is a poor correlation between the amount of orbital involvement and severity of thyroid disease. Orbitopathy may precede thyroid disease or occur with euthyroid or hypothyroid states, but is more common with Graves’ disease. The inferior rectus is most often involved followed by the medial rectus and superior muscle complex. There is a tendency to bilateral and often symmetric involvement. Isolated lateral rectus involvement is rare Citation[41,47].
By imaging, there is an increased volume of orbital fat, a nonspecific sign. Initially the fat is unaffected, but as the inflammation advances, it becomes ‘dirty’. Enlarged muscles produce apical crowding and venous congestion. Involved muscles are usually fusiform in appearance with a characteristic sparing of their tendinous portions. These imaging features are well diagnosed and monitored with CT scan or MRI Citation[40,41,46]. When using CT scan, one needs to remember that iodinated contrast affects the treatment of systemic thyroid disease. A fat-saturated T2-WI sequence can be used to predict and monitor response to therapy by distinguishing between muscle inflammation and fibrosis. On the basis of T2-WI relaxation times, patients with high T2-WI signal intensity values may benefit from corticosteroid therapy Citation[41,46].
Common tumors
Optic nerve gliomas
Optic nerve gliomas are the most common neoplasias of the optic nerves in children. They present in the first decade of life, 50% in patients less than 5 years of age Citation[38,39,43,47]. More than half of patients have neurofibromatosis (NF) type 1, and optic pathway glioma is the most common CNS neoplasm in NF1. These tumors can involve one or both optic nerves (unilateral involvement is common except in NF1), chiasm, and optic tracts. Bilateral disease is pathognomonic for NF1. Almost all optic nerve gliomas are considered WHO grade I pilocytic juvenile astrocytomas. Optic nerve gliomas in children are benign, well differentiated and typically slow growing. There is usually a disparity between the amount of vision loss and tumor size. The tumors diffusely expand the nerve in a fusiform fashion with characteristic kinking and buckling and widen the optic canal (90%) Citation[41,48,49]. The classic appearance at CT or MRI is specific Citation[50]. Imaging varies if there is cyst formation or mucinous components. Areas of necrosis and hemorrhage are rare. Calcification is also rare before radiotherapy. MRI is the preferred technique because it better demonstrates the chiasm and optic tracts. Screening of the brain should be performed simultaneously to the orbit examination. The best sequence is fat-suppressed T1-WI after contrast administration. Tumors appear iso- to hypointense to the optic pathway on T1-WI, slightly T2-WI hyperintense, and show moderate-to-marked contrast enhancement Citation[37,38,40,41,48–50].
Optic nerve meningioma
Optic nerve meningioma comprise approximately one-third of primary orbital tumors in the adult population, but are rare in the pediatric age group. They can be associated with NF2, prior radiotherapy or meningiomatosis. On imaging studies, meningiomas may show calcification, hyperostosis and optic canal widening or narrowing, which can compress the optic nerve. MRI usually demonstrates that they are isointense to the optic nerves on T1- and T2-WIs, although they may have low signal on both sequences. Cystic components may be identified between the tumor and globe due to focal sheath dilatation (capping cyst). Optic nerve meningioma enhances uniformly, producing a tramtrack sign on axial images due to the enveloped nonenhancing and attenuated optic nerve, best shown in MRI postcontrast fat-suppressed T1-WIs Citation[40,41,48].
Retinoblastoma
Retinoblastoma is the most common intraocular malignant tumor in childhood. It is an embryonal tumor arising from the retina in infants, and typically presents before 5 years of age (80% occur in infants <2 years of age). Incidence ranges from 1 in 17,000 to 1 in 24,000 live births Citation[38,47,49–51]. There is no gender or race predominance. There are heritable and nonheritable forms of Rb, the former being autosomal dominant. The sporadic form (90%) is most frequent and is unilateral. When Rb is hereditary, 85% are bilateral and there is an increased risk of developing second malignancies. In 2–8% of patients with bilateral Rbs, a tumor may develop intracranially, typically a pinealoblastoma. Those patients are younger, with a mean age of 7 months.
The most frequent presenting sign of Rb is leukocoria, a white pupillary reflex. The main differential diagnosis includes:
• Persistent hyperplasic primary vitreous, a congenital developmental anomaly of the eye resulting from failure of the embryological primary vitreous and hyaloid vasculature to regress;
• Coat’s disease, a typically unilateral disease characterized by abnormal development of blood vessels behind the retina;
• Toxocara canis, an infectious disease of the eye associated with exposure to infected puppies;
• Retinopathy of prematurity associated with low-birth-weight infants who receive supplemental oxygen in the period immediately after birth.
The most common spread pattern of Rb is a mixture of endophytic and exophytic growth. Hematogeneous metastases go to the lungs, bones, brain and other viscera, while lymphatic metastases are found in regional nodes Citation[49]. Current histopathologic risk factors for metastasis include invasion of the optic nerve, anterior eye segment or massive invasion of choroid and sclera. Tumor angiogenesis is also a histopathologic risk factor for local tumor invasion and systemic dissemination Citation[52].
Because inappropriate intraocular procedures can result in disease dissemination, indirect approaches are required to make an accurate diagnosis of Rb. On imaging, Rb presents as a nodular mass in the posterior globe with calcifications in 95% of cases. In children younger than 3 years of age intraocular calcification is highly suspicious of Rb, but in extraocular metastatic Rb calcification is rare Citation[38,50]. The size of the globe is generally normal. The best imaging technique for demonstrating the characteristic calcifications is CT scan, but as stated earlier it is being used less to avoid radiation exposure. MRI is best in assessing extension and spread of tumor, showing it to be of intermediate-to-mild hyperintensity on T1- and hypointense on T2-WIs. Retinal detachment and cystic changes may be apparent. Invasion through the optic disc into the nerve is relatively common in advanced diseases. Rbs present variable degrees of enhancement after gadolinium administration Citation[50,51]. Graaf et al. found that the degree of abnormal anterior eye segment enhancement on MRI reflects angiogenesis in the iris and is also a hallmark of advanced disease because its degree correlates with tumor volume and optic nerve invasion Citation[52]. In our experience, Rb shows restricted diffusion on DWI due to hypercellularity .
Melanoma
Ocular melanoma is the most common primary intraocular malignancy in adults Citation[44,47]. In childhood, orbital melanoma is rare; half of cases occur around the iris. The growth rate of childhood melanoma is the best indicator of malignancy. Congenital melanosis, ocular melanocytosis, oculodermal melanocytosis, and uveal nevi are conditions that predispose to uveal melanoma.
Melanomas are seen as well-circumscribed hyperdense masses on CT. MRI characteristics of melanotic melanomas are related to the paramagnetic properties of melanin. Most of them are hyperintense on T1- and hypointense on T2-WIs, and show contrast enhancement. They can also show restricted diffusion Citation[53]. Calcification is rare, but exudative or hemorrhagic retinal detachment may be present Citation[44,47,49].
Rhabdomyosarcoma
Rhabdomyosarcoma (Rms) is a rare tumor with an annual incidence of 4.3 cases per million children. The orbit is the primary site in approximately 10% of these tumors. From the ophthalmologist’s standpoint, Rms is the most common malignant orbital tumor of childhood Citation[54]. Although Rms was once believed to arise from extraocular muscles, it is now accepted that orbital Rms develops from undifferentiated mesenchymal cells that have the capacity to differentiate into striated muscle Citation[54].
The child usually presents with rapidly progressive proptosis and ocular motility restriction. The differential diagnosis includes benign and malignant neoplasms, vascular tumors, leukemia, Burkitt lymphoma, allergic sino-orbital aspergillosis, orbital pseudotumor, orbital cellulites, histiocytosis and metastatic neuroblastoma.
Ultrasound shows a heterogeneous, well-defined, irregular mass of low or medium echogenicity contiguous with the muscles Citation[37]. CT scan demonstrates an irregular, moderately well-defined soft tissue orbital mass isodense to the extraocular muscles. Most tumors occupy the extraconal space, with approximately 50% extending intraconally. These hypercellular tumors may erode bone and extend intracranially, reflecting their aggressive behavior Citation[54].
On MRI, the tumor may appear isointense to muscle and hypointense to fat on T1-WI, and usually hyperintense to both muscles and fat on T2-WI. Rms generally show moderate and uniform contrast enhancement Citation[37].
Metastatic tumors
In children, unlike adults, metastatic involvement of the orbit is less common than primary tumors. Neuroblastoma is the most common source, generally occurring below 2 years of age. From 8 to 20% of patients diagnosed with neuroblastoma have orbital involvement, and 40% of these tumors are bilateral. Ewing sarcoma and rhabdomyosarcoma may occasionally metastasize to the orbit Citation[37]. Ocular metastases typically occur in the posterior segment of the globe and usually thicken the uveal tract diffusely. Extraocular muscles are involved in less than 10% of patients by metastases Citation[41,43,44,47]. MRI is the method of choice for their detection. Metastases appear as focal or extensive ill-defined infiltrating soft tissue lesions. Signal characteristics are heterogeneous depending on the amount of necrosis and hemorrhage. Partial orbital wall destruction may be seen (better with CT) usually in the superolateral wall. Tumor enhances after intravenous contrast administration Citation[37,39,47,48].
Vascular lesions
Infantile hemangioma
Infantile hemangioma is the most common tumor in the orbits in children with an incidence of 4–5.6%. Infantile hemangiomas have a predictable biologic behavior consisting of three phases. They appear at birth or approximately 2 weeks postpartum, grow fast during the first 6 months of life, stabilize during the second year, and then slowly involute over 5 years. Generally, the faster the lesion enlarges, the faster it involutes. The most frequent ocular complications associated with infantile hemangiomas are related to amblyopia. Infantile hemangiomas are more common in females. There are no hereditary or familial patterns and no geographic predilection, although they are uncommon in African–Americans Citation[37,39,40,55–58].
The most useful immunohistochemical marker of infantile hemangioma is glucose transporter protein isoform 1 (GLUT1), which is expressed by endothelial cells of infantile hemangiomas at all stages and not in other benign vascular anomalies Citation[56,57].
With Valsalva maneuver infantile hemangiomas enlarge and change in color. A prominent arterial supply is usually demonstrable from either the external or internal carotid circulation. These tumors may bleed profusely Citation[38]. Orbital infantile hemangiomas may be accompanied by hemangiomas elsewhere, the airway being one of the most important to detect because they can cause respiratory difficulties. US can be used to follow-up and monitor growth or involution Citation[58].
Imaging appearance is that of a well-circumscribed lobular homogeneous soft-tissue mass, typically retrobulbar. It is usually extraconal, but may be intraconal or both. The orbit may be enlarged and intracranial extension is not common. Their signal intensity is determined by fibrosis, calcification, thrombosis and hemosiderin deposition Citation[41]. During the proliferative phase, they are iso- to hypointense to muscle on T1- and moderately hyperintense with associated flow voids on T2-WIs. Intense contrast enhancement is present. During the involuting phase, they appear heterogeneous and enhance to a lesser degree. The presence of fibrofatty tissues characterizes the final involution phase Citation[37,38,40,41,55,58].
Congenital nonprogressive hemangiomas
Congenital nonprogressive hemangiomas differ from the classic infantile hemangiomas in that they are fully formed at birth, follow a static or rapidly involuting course, the latter much more rapid than that of infantile hemangiomas, and are histologically and immunophenotypically distinct from classic infantile hemangioma. They are subdivided into rapidly involuting and noninvoluting types often referred to as RICH and NICH, respectively. Unlike infantile hemangiomas, but similar to vascular malformations, congenital hemangiomas occur equally in males and females. Diagnosis can be made with confidence clinically, although the differential diagnosis may include arteriovenous malformation or infantile hemangioma. Histological confirmation may be needed, supplemented by negative GLUT1 immunohistochemistry. By imaging, their presentation is similar to infantile hemangiomas Citation[55–57].
Lymphatic malformations
Lymphatic malformations are developmental errors in morphogenesis of the lymphatic vascular system, and occur sporadically. Most lymphatic malformations are evident at birth or during the first or second years of life Citation[37,41,56,57]. They may be extraconal and/or intraconal, and represent a network of veno–lymphatic channels with foci of lymphoid tissues. This explains their propensity for sudden increase in size due to hemorrhage or viral infection. The more superficially located they are, the larger their lymphatic components are. Sudden intralesional hemorrhage is frequent, but otherwise they gradually and progressively enlarge during the first two decades of life Citation[38]. They are often associated with fat and bony overgrowth.
On MRI, lymphatic malformations are multilobulated, poorly circumscribed, heterogeneous, nonencapsulated and multicompartmental lesions containing septae. A majority of the lesion is often T1-WI hypointense and T2-WI hyperintense, and may be predominantly cystic. They may bleed or have proteinaceous contents, giving variable signal intensities in all sequences. Fluid levels and blood products in different stages of degradation may also be seen. Flow voids are absent, and this helps distinguish them from infantile hemangiomas. After intravenous contrast administration, little enhancement, if any, is appreciated Citation[38,39]. The use of contrast material with fat saturation may help in identifying their venous components and solid vascular tissues. Nonenhancing regions correspond to lymphatic components of the lesion. Calcification is rare Citation[37,38,41,58].
Expert commentary
While avoiding the radiation exposure associated with CT scanning and the operator-dependent image quality associated with US, MRI provides exquisite anatomical resolution. However, the most important advantage of MRI over other imaging techniques is its ability to image the retro-orbital visual pathways and related structures in great detail. In addition, MRI offers physiologic information not obtainable with other imaging studies. DWI is rapidly carried out and although it results in distorted images, it offers findings that are specific for collections comprised of pus such as those occurring in medial subperiosteal and lacrimal gland abscess. DWI may also aid in the diagnosis of hypercellular lesions and help monitor their response to treatment. DWI is also very promising in ischemic lesions of the optic nerve and retina. It is now routinely use in the evaluation of cerebral ischemia. MRS helps in formulating a differential diagnosis for suprasellar/chiasmatic lesions, many of which are not amenable to biopsy and/or resection. DTI and DTT offer the possibility of visualizing, tracking and assessing myelination of the optic nerves and tracts, and may be useful in the planning of resection of lesions that are located close to these structures. Perfusion images are unique in that they may measure the blood flow and volume of masses and lesions, and be helpful in their initial diagnosis and follow-up.
Five-year view
Diseases affecting the orbit in the pediatric population are not uncommon. Their clinical diagnosis and imaging evaluation needs to be prompt as the patient’s outcome depends on establishing the correct diagnosis and adequate treatment. For these reasons, the use of imaging should continue to increase and it is important to keep in mind the radiation exposure due to CT scan and the inherent limitations of MRI, which are mostly due to motion-related artifacts. Newer techniques in MRI permit assessment of a lesion’s cellularity (MRS and DWI), vascularity (perfusion techniques), presence of calcifications and hemorrhage (gradient echo-recalled imaging sequences), tumor invasion (contrast enhancement) and detection of pus (DWI), and although all of the techniques have some drawbacks, their greater benefits and advantages should continue to place MRI at the forefront of orbital imaging. Up to now, most of these techniques have been studied in adult patients, but it is our feeling that they will soon become very important in the pediatric population since other less invasive methods such as US are difficult to use in children.
Key issues
• Radiation exposure associated with computed tomography (CT) is a concern in both adults and children, but children are particularly at risk because they are considerably more sensitive to radiation, have a longer life expectancy and receive a higher dose of radiation when adults CT settings are used (thus all equipment should be calibrated for use in children).
• There are three ways to reduce the overall radiation dose from CT: decrease the number of CT studies, replace CT with a different imaging technique when practical (sonography and/or MRI) and reduce the CT-related dose in individual patients by adjusting CT parameters.
• Rapidly evolving MRI techniques provide faster acquisition time and higher image resolution, which is important in pediatric imaging.
• Recently, some advanced MRI techniques have been used in pediatric orbital imaging in order to help confirm the clinical diagnosis, provide early detection of disease, use as an alternative to CT and to help in preoperative evaluation.
• The spectrum of congenital anomalies involving the orbit is wide, and we have focused on two of the most common: dermoid and epidermoid cysts that usually have signal intensities on MRI which are typical. Epidermoids show restriction of water diffusion on diffusion-weighted imaging and appear as bright lesions.
• Infectious and inflammatory diseases are frequent disorders in children, with preseptal cellulitis the most common. Imaging is not required unless it is complicated by suspicion of an abscess, thrombosis or meningitis. Painless exophthalmos is a useful clinical sign for differentiating infections from inflammatory orbital pseudotumor.
• A large number of neoplasias, both benign and malignant, may present in children’s orbits. The most important are optic nerve glioma and retinoblastoma. The former is usually seen in neurofibromatosis type 1. CT for calcification detection is no longer required for the imaging diagnosis of retinoblastoma. The diagnosis may be suggested based on clinical and MRI findings.
• Infantile hemangioma is the most prevalent vascular lesion in children. The diagnosis is usually clinical, and imaging can show the classical presentation sharing three distinct phases of involution in addition to mapping their extent and differentiating them from other lesions.
References
- Mills DM, Tsai S, Meyer DR, Belden C. Pediatric ophthalmic computed tomographic scanning and associated cancer risk. Am. J. Ophthalmol.142(6), 1046–1053 (2006).
- Brenner DJ, Hall EJ. Computed tomography – an increasing source of radiation exposure. N. Engl. J. Med.357(22), 2277–2284 (2007).
- Frush DP, Donnelly LF, Rosen NS. Computed tomography and radiation risks: what pediatric health care providers should know. Pediatrics112(4), 951–957 (2003).
- Pierce DA, Preston DL. Radiation related risks at low doses among atomic bomb survivors. Radiat. Res.154(2), 178–186 (2000).
- Semelka RC, Armao DM, Elias J, Huda W. Imaging strategies to reduce the risk of radiation in CT studies, including selective substitution with MRI. J. Magn. Reson. Imaging25(5), 900–909 (2007).
- Lee AG, Johnson MC, Policeni BA, Smoker WRK. Imaging for neuro-ophthalmic and orbital disease – a review. Clin. Experiment. Ophthalmol.37(1), 30–53 (2009).
- Galluzzi P, Hadjistilianou T, Cerase A, De Francesco S, Toti P, Venturi C. Is CT still useful in the study protocol of retinoblastoma? AJNR Am. J. Neuroradiol.30(9), 1760–1765 (2009).
- MacKenzie JD, Vasanawala SS. Advances in pediatric MR imaging. Magn. Reson. Imaging Clin. N. Am.16(3), 385–402 (2008).
- Bammer R, Holdsworth SJ, Veldhuis WB, Skare ST. New methods in diffusion-weighted and diffusion tensor imaging. Magn. Reson. Imaging Clin. N. Am.17(2), 175–204 (2009).
- Conneely MF, Hacein-Bey L, Jay WM. Magnetic resonance imaging of the orbit. Semin. Ophthalmol.23(3), 179–189 (2008).
- Casselman J, Mermuys K, Delanote J, Ghekiere J, Coenegrachts K. MRI of the cranial nerves – more than meets the eye: technical considerations and advanced anatomy. Neuroimag. Clin. N. Am.18(2), 197–231 (2008).
- Georgouli T, James T, Tanner S et al. High-resolution microscopy coil MR-eye. Eye22(8), 994–996 (2008).
- Kolk A, Stimmer H, Klopfer M et al. High resolution magnetic resonance imaging with an orbital coil as an alternative to computed tomography scan as the primary imaging modality of pediatric orbital fractures. J. Oral Maxillofac. Surg.67(2), 348–356 (2009).
- Chavhan GB, Babyn PS, Thomas B, Shroff MM, Haacke EM. Principles, techniques, and applications of T2*-based MR imaging and its special applications. Radiographics29(5), 1433–1449 (2009).
- Schaefer PW, Grant PE, Gonzalez RG. Diffusion-weighted MR imaging of the brain. Radiology217(2), 331–345 (2000).
- Sepahdari AR, Aakalu VK, Edward RK et al. MRI of orbital cellulitis and orbital abscess: the role of diffusion-weighted imaging. AJR Am. J. Roentgenol.193(3), W244–W250 (2009).
- Willinek WA, Kuhl CK. 3.0 T neuroimaging: technical considerations and clinical applications. Neuroimag. Clin. N. Am.16(2), 217–228 (2006).
- Kapur R, Sepahdari AR, Mafee MF, Putterman AM, Aakalu V, Wendel LJA. MR imaging of orbital inflammatory syndrome, orbital cellulitis, and orbital lymphoid lesions: the role of diffusion-weighted imaging. AJNR Am. J. Neuroradiol.30(1), 64–70 (2009).
- Mathur S, Karimi A, Mafee MF. Acute optic nerve infarction demonstrated by diffusion-weighted imaging in a case of rhinocerebral mucormycosis. AJNR Am. J. Neuroradiol.28(3), 489–490 (2007)
- Hatipoglu HG, Gurbuz MO, Yuksel E. Restricted diffusion in the optic nerve and retina demonstrated by MRI in rhino-orbital mucormycosis. J. Neuroophthalmol.29(1), 13–15 (2009).
- Klein JP, Cohen AB, Kimberly WT et al. Diffusion-weighted magnetic resonance imaging of bilateral simultaneous optic nerve infarctions. Arch. Neurol.66(1), 132–133 (2009).
- Karagülle T, Erden A, Erden I, Zilelioğlu G. Nasolacrimal system: evaluation with gadolinium-enhanced MR dacryocystography with a three-dimensional fast spoiled gradient-recalled technique. Eur. Radiol.12(9), 2343–2348 (2002).
- Nucifora PGP, Verma R, Lee S, Melhem ER. Diffusion-tensor MR imaging and tractography: exploring brain microstructure and connectivity. Radiology245(2), 367–384 (2007).
- Garaci FG, Bolacchi F, Cerulli A et al. Optic nerve and optic radiation neurodegeneration in patients with glaucoma: in vivo analysis with 3-T diffusion-tensor MR imaging. Radiology252(2), 496–501 (2009).
- Xu J, Sun S, Naismith RT, Snyder AZ, Cross AH, Song S. Assessing optic nerve pathology with diffusion MRI: from mouse to human. NMR Biomed.21(9), 928–940 (2008).
- Trip SA, Wheeler-Kingshott C, Jones SJ et al. Optic nerve diffusion tensor imaging in optic neuritis. NeuroImage30(2), 498–505 (2006).
- Salmela MB, Cauley KA, Nickerson JP, Koski CJ, Filippi CG. Magnetic resonance diffusion tensor imaging (MRDTI) and tractography in children with septo-optic dysplasia. Pediatr. Radiol.40(5), 708–713 (2010).
- Kim S, Melhem ER. Science to practice: does diffusion-tensor MR imaging provide accurate tracing of specific white matter tracts that correspond to actual anatomic and functional units in the central nervous system? Radiology249(3), 725–727 (2008).
- Berman J. Diffusion MR tractography as a tool for surgical planning. Magn. Reson. Imaging Clin. N. Am.17(2), 205–214 (2009).
- Yamamoto A, Miki Y, Urayama S et al. Diffusion tensor fiber tractography of the optic radiation: analysis with 6-, 12-, 40-, and 81-directional motion-probing gradients, a preliminary study. AJNR Am. J. Neuroradiol.28(1), 92–96 (2007).
- Salmela MB, Cauley KA, Andrews T, Gonyea JV, Tarasiewicz I, Filippi CG. Magnetic resonance diffusion tensor imaging of the optic nerves to guide treatment of pediatric suprasellar tumors. Pediatr. Neurosurg.45(6), 467–471 (2009).
- Packer RJ, MacDonald T, Vezina G. Central nervous system tumors. Pediatr. Clin. North Am.55(1), 121–145 (2008).
- Panigrahy A, Nelson MD Jr, Blüml S. Magnetic resonance spectroscopy in pediatric neuroradiology: clinical and research applications. Pediatr. Radiol.40(1), 3–30 (2010).
- Saleem SN, Said AM, Lee DH. Lesions of the hypothalamus: MR imaging diagnostic features. RadioGraphics27(4), 1087–1108 (2007).
- Paldino MJ, Barboriak DP. Fundamentals of quantitative dynamic contrast-enhanced MR imaging. Magn. Reson. Imaging Clin. N. Am.17(2), 277–289 (2009).
- Jost SC, Ackerman JW, Garbow JR, Manwaring LP, Gutmann DH, McKinstry RC. Diffusion-weighted and dynamic contrast-enhanced imaging as markers of clinical behavior in children with optic pathway glioma. Pediatr. Radiol.38(12), 1293–1299 (2008).
- Gorospe L, Royo A, Berrocal T, García-Raya P, Moreno P, Abelairas J. Imaging of orbital disorders in pediatric patients. Eur. Radiol.13(8), 2012–2026 (2003).
- Mafee MF, Mafee RF, Malik M, Pierce J. Medical imaging in pediatric ophthalmology. Pediatr. Clin. North Am.50(1), 259–286 (2003).
- Barkovich AJ. Pediatric Neuroimaging (4th Edition). Lippincott Williams & Wilkins, PA, USA (2005).
- Goh PS, Gi MT, Charlton A, Tan C, Gangadhara Sundar JK, Amrith S. Review of orbital imaging. Eur. J. Radiol.66(3), 387–395 (2008).
- Aviv RI, Miszkiel K. Orbital imaging: Part 2. Intraorbital pathology. Clin. Radiol.60(3), 288–307 (2005)
- LaRocca V, Gorelick G, Kaufman LM. Medical imaging in pediatric neuro-ophthalmology. Neuroimaging Clin. N. Am.15(1), 85–105 (2005).
- Weber AL, Caruso P, Sabates NR. The optic nerve: radiologic, clinical, and pathologic evaluation. Neuroimaging Clin. N. Am.15(1), 175–201 (2005).
- Mafee MF, Karimi A, Shah J, Rapoport M, Ansari SA. Anatomy and pathology of the eye: role of MR imaging and CT. Neuroimaging Clin. N. Am.15(1), 23–47 (2005).
- Prentiss KA, Dorfman DH. Pediatric ophthalmology in the emergency department. Emerg. Med. Clin. North. Am.26(1), 181–198 (2008).
- Wippold FJ 2nd; Expert Panel on Neurologic Imaging. Orbits, vision, and visual loss. AJNR Am. J. Neuroradiol.31(1), 196–198 (2010).
- Pramanik BK, Yousem DM. Orbit and visual pathways. In:Disease of the Brain, Head & Neck, Spine. Diagnostic Imaging and Interventional Techniques (40th Anniversary IDKD). Hodler J, von Schulthess GK, Zollikofer CHL (Eds). Springer-Verlag, Milan, Italy, 130–134 (2008).
- Belden CJ. MR imaging of the globe and optic nerve. Neuroimaging Clin. N. Am.14(4), 809–825 (2004).
- Jäger HR, Miszkiel KA. Pathology of the optic nerve. Neuroimaging Clin. N. Am.18(2), 243–259 (2008).
- Chung EM, Specht CS, Schroeder JW. From the archives of the AFIP: pediatric orbit tumors and tumorlike lesions: neuroepithelial lesions of the ocular globe and optic nerve. Radiographics27(4), 1159–1186 (2007).
- Apushkin MA, Apushkin MA, Shapiro MJ, Mafee MF. Retinoblastoma and simulating lesions: role of imaging. Neuroimaging Clin. N. Am.15(1), 49–67 (2005).
- Graaf P, Van der Valk P, Moll AC et al. Contrast-enhancement of anterior eye segment in patients with retinoblastoma: correlation between clinical, MR imaging, and histopathologic findings. AJNR Am. J. Neuroradiol.31, 237–245 (2010).
- Hayashida Y, Hirai T, Morishita S et al. Diffusion-weighted imaging of metastatic brain tumors: comparison with histologic type and tumor cellularity. AJNR Am. J. Neuroradiol.27(7), 1419–1425 (2006).
- Karcioglu ZA, Hadjistilianou D, Rozans M, DeFrancesco S. Orbital rhabdomyosarcoma. Cancer Control11(5), 328–333 (2004)
- Viswanathan V, Smith ER, Mulliken JB et al. Infantile hemangiomas involving the neuraxis: clinical and imaging findings. AJNR Am. J. Neuroradiol.30(5), 1005–1013 (2009).
- North PE. Vascular tumors and malformations of infancy and childhood. Pathol. Case Rev.13, 213–235 (2008).
- North PE, Waner M, Buckmiller L, James CA, Mihm MC Jr. Vascular tumors of infancy and childhood: beyond capillary hemangioma. Cardiovasc. Pathol.15(6), 303–317 (2006).
- Bilaniuk LT. Vascular lesions of the orbit in children. Neuroimaging Clin. N. Am.15(1), 107–120 (2005).
Advances in pediatric orbital magnetic resonance imaging
To obtain credit, you should first read the journal article. After reading the article, you should be able to answer the following, related, multiple-choice questions. To complete the questions and earn continuing medical education (CME) credit, please go to www.medscapecme.com/journal/expertophth. Credit cannot be obtained for tests completed on paper, although you may use the worksheet below to keep a record of your answers. You must be a registered user on Medscape.com. If you are not registered on Medscape.com, please click on the New Users: Free Registration link on the left hand side of the website to register. Only one answer is correct for each question. Once you successfully answer all post-test questions you will be able to view and/or print your certificate. For questions regarding the content of this activity, contact the accredited provider, [email protected]. For technical assistance, contact [email protected]. American Medical Association’s Physician’s Recognition Award (AMA PRA) credits are accepted in the US as evidence of participation in CME activities. For further information on this award, please refer to www.ama-assn.org/ama/pub/category/2922.html. The AMA has determined that physicians not licensed in the US who participate in this CME activity are eligible for AMA PRA Category 1 Credits™. Through agreements that the AMA has made with agencies in some countries, AMA PRA credit is acceptable as evidence of participation in CME activities. If you are not licensed in the US and want to obtain an AMA PRA CME credit, please complete the questions online, print the certificate and present it to your national medical association.
Activity Evaluation: Where 1 is strongly disagree and 5 is strongly agree
1. A 3-year-old boy presents to his primary care provider with a suspected orbital mass. Which of the following factors should be considered by the ordering physician in determining appropriate diagnostic imaging?
□ A Children are at similar risk to adults for radiation exposure associated with computed tomography (CT)
□ B To reduce risk, CT equipment should be calibrated for use in children
□ C It is seldom feasible to substitute magnetic resonance imaging (MRI) for orbital CT scanning
□ D The only way to reduce the overall radiation dose from CT is to reduce the number of CT studies performed
2. The primary care provider consults with a pediatric neurologist about the imaging decision. Which of the following statements about MRI orbital imaging is incorrect?
□ A MRI is ineffective in imaging the retro-orbital visual pathways
□ B Rapidly evolving MRI techniques allow faster acquisition time and higher image resolution than ultrasound
□ C Diffusion-weighted imaging (DWI) is rapidly performed and results in distorted images, but often yields specific findings
□ D Diffusion tensor imaging (DTI) incorporates directionality into DWI to study tissue microstructure and brain anatomy
3. Orbital MRI of the child shows restriction of water diffusion on DWI appearing as a bright lesion, containing adnexal structures, in the anterior superolateral orbit. Which of the following diagnoses is most likely?
□ A Epidermoid cyst
□ B Dermoid cyst
□ C Orbital pseudotumor
□ D Optic nerve glioma