Abstract
Positive caloric balance often causes pathologic adipocyte and adipose tissue anatomical and functional changes (termed adiposopathy or ‘sick fat’), which may lead to pathogenic adipocyte and adipose tissue responses and metabolic disease. Fat weight loss may improve adiposopathy, and thus improve metabolic disease in overweight patients. Unfortunately, the efficacy of non-surgical weight loss therapies is often limited due to redundant physiological systems that help ‘protect’ against starvation and/or negative caloric balance. One strategy to overcome these limitations is to combine weight loss drug therapies having complementary mechanisms of action, thereby affecting more than one physiologic process influencing body fat accumulation. Phentermine is a noradrenergic sympathomimetic amine approved for short-term treatment of obesity. Topiramate is a sulfamate-substituted monosaccharide derivative of the naturally occurring sugar monosaccharide D-fructose approved as a treatment for migraine headaches and seizure disorders. Although known to facilitate weight loss since its approval, topiramate monotherapy does not have a regulatory indication as an anti-obesity agent. Phentermine HCl/topiramate controlled-release (PHEN/TPM CR) is a combination agent containing immediate-release phentermine and controlled-release topiramate. Clinical trials involving thousands of patients demonstrate PHEN/TPM CR to be effective in improving the weight of patients, and also effective in improving adiposopathy-associated metabolic diseases. This review examines the pathophysiology of adiposopathy as a contributor to metabolic disease, the data supporting phentermine monotherapy, topiramate monotherapy and their combination as anti-obesity and anti-adiposopathy agents, and the preliminary evidence supporting PHEN/TPM CR as a generally well-tolerated and effective agent to improve metabolic disease.
CNS factors may increase anorexigenic POMC (which is cleaved to form melanocortins such as MSH) and CART expression, and decrease orexigenic expression of NPY and AgRP. Alterations in these appetitive effectors contribute to ‘second-order’ signaling through arcuate nucleus neuron projection to other hypothalamic regions. The net result is increased anorexigenic and increased catabolic effects, with decreased orexigenic and decreased anabolic effects. This figure is greatly simplified, and does not show all the interconnected signaling pathways between these CNS factors.
†The reported effects of these CNS factors on POMC and NPY are not always consistent in the literature, with more research needed to confirm these effects.
‡While CNS adiponectin may have anorexigenic effects Citation[175], adiponectin may not cross the blood–brain barrier Citation[176], so the physiological importance of adiponectin on the CNS is unclear Citation[140].
§Meant to reflect the fed versus non-fed state as reflected by relative physiologic increases or decreases in glucose levels. While the signaling may be glucose mediated, this is not necessarily meant to represent pathologic hyperglycemia.
AgRP: Agouti-related peptide; BDNF: Brain-derived neurotrophic factor; CART: Cocaine amphetamine-regulated transcript; CB1R: Cannabinoid 1 receptor; CRH: Corticotropin-releasing hormone; GLP-1: Glucagon-like peptide-1; MC3R: Melanocortin 3 receptor; MC4R: Melanocortin 4 receptor; MCH: Melanin-concentrating hormone; MSH: Melanocyte-stimulating hormone; NPY: Neuropeptide Y; POMC: Pro-opiomelanocortin; PYY 3–31: Peptide YY; TRH: Thyroid-releasing hormone; VIP: Vasoactive intestinal peptide.
Data taken from Citation[23,173,174].
![Figure 1. Simplified relationship between selected CNS factors† on anorexigenic and orexigenic effectors within the hypothalamus.CNS factors may increase anorexigenic POMC (which is cleaved to form melanocortins such as MSH) and CART expression, and decrease orexigenic expression of NPY and AgRP. Alterations in these appetitive effectors contribute to ‘second-order’ signaling through arcuate nucleus neuron projection to other hypothalamic regions. The net result is increased anorexigenic and increased catabolic effects, with decreased orexigenic and decreased anabolic effects. This figure is greatly simplified, and does not show all the interconnected signaling pathways between these CNS factors.†The reported effects of these CNS factors on POMC and NPY are not always consistent in the literature, with more research needed to confirm these effects.‡While CNS adiponectin may have anorexigenic effects Citation[175], adiponectin may not cross the blood–brain barrier Citation[176], so the physiological importance of adiponectin on the CNS is unclear Citation[140].§Meant to reflect the fed versus non-fed state as reflected by relative physiologic increases or decreases in glucose levels. While the signaling may be glucose mediated, this is not necessarily meant to represent pathologic hyperglycemia.AgRP: Agouti-related peptide; BDNF: Brain-derived neurotrophic factor; CART: Cocaine amphetamine-regulated transcript; CB1R: Cannabinoid 1 receptor; CRH: Corticotropin-releasing hormone; GLP-1: Glucagon-like peptide-1; MC3R: Melanocortin 3 receptor; MC4R: Melanocortin 4 receptor; MCH: Melanin-concentrating hormone; MSH: Melanocyte-stimulating hormone; NPY: Neuropeptide Y; POMC: Pro-opiomelanocortin; PYY 3–31: Peptide YY; TRH: Thyroid-releasing hormone; VIP: Vasoactive intestinal peptide.Data taken from Citation[23,173,174].](/cms/asset/a1648bc6-e607-4847-90ec-a0687963d677/ierk_a_11210709_f0001_b.jpg)
†Topiramate doses may be as low as 50 mg per day for prophylaxis of migraine headaches, and as high as 400 mg per day for treatment of seizure disorders.
‡Both phentermine and topiramate are approved drugs. Neither phentermine nor topiramate have product information that warns of potential adverse experiences with their combined use.
§The ‘low dose’ PHEN/TPM CR evaluated in clinical trials contained 23 mg of topiramate CR.
¶It is yet to be proven that controlled-release topiramate affords additional efficacy or safety advantages over non-controlled-release formulations.
PHEN/TPM CR: Phentermine HCl and topiramate controlled-release.
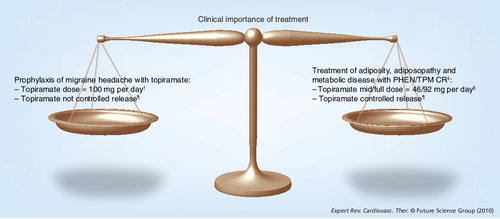
Clinical overview
Adiposity promotes the most common metabolic diseases encountered in clinical medicine Citation[1]. Many of these metabolic diseases are major atherosclerotic coronary heart disease (CHD) risk factors (e.g., diabetes mellitus, high blood pressure [BP] and dyslipidemia) Citation[2,3]. The association between increased adiposity, metabolic disease and CHD risk is not absolute Citation[4]. In some individuals, adiposity may elicit limited pathogenic adipose tissue endocrine and immune responses, which are made even less pathogenic if other body organs are able to mitigate these pathogenic adipose tissue responses. Such patients may develop fat-mass-related complications; however, adiposity-induced metabolic diseases may be avoided or delayed Citation[5]. Conversely, even modest fat weight gain among genetically or environmentally susceptible patients may incite pathogenic adipose tissue endocrine and immune responses that lead to metabolic disease Citation[6]. Thus, adiposity is most likely to ‘cause’ metabolic disease when fat weight gain results in pathogenic adipose tissue endocrine and immune adipose tissue responses in patients having other body organs (e.g., liver, muscle, brain etc.) with inadequate potential to prevent the pathologic metabolic consequences of dysfunctional adipose tissue Citation[2].
Adipose tissue as a pathogenic organ
Clinicians often apply the suffix ‘pathos’ to describe diseased organs, such as cardiomyopathy, myopathy, encephalopathy, ophthalmopathy, retinopathy, enteropathy, nephropathy, neuropathy and dermopathy. ‘Adiposopathy’ is a term applied to reflect anatomic abnormalities of adipocytes and adipose tissue that arises from fat weight gain, and that results in pathogenic endocrine and immune adipose tissue responses adversely affecting metabolic health. In lay terms, ‘adiposopathy’ can be characterized as representing ‘sick fat’, which is an endocrine ‘disease’ Citation[7].
Adipogenesis
The degree to which adiposity is manifest as adiposopathy largely depends on the effects of a positive caloric balance on adipogenesis. During positive caloric balance, adipocytes normally undergo increased recruitment, proliferation and differentiation; during a negative caloric balance, adipocytes shrink in size and/or may undergo apoptosis Citation[8–13]. If, during positive caloric balance, increased adipogenesis provides adequate, added adipose tissue functionality, then patients may avoid adverse metabolic consequences associated with adiposity. Conversely, if adipogenesis is impaired, then inadequate recruitment, proliferation and/or differentiation may cause adipocytes and adipose tissue to become dysfunctional, pathogenic, and contributory to metabolic disease. Specifically, if during positive caloric balance the recruitment and proliferation of new fat cells is impaired, then existing fat cells may become excessively enlarged. For decades, data support excessive adipocyte hypertrophy as being associated with adipocyte and adipose tissue dysfunction, which contributes to metabolic disease Citation[6,14–16].
Fat depots
The pathogenic potential of adipose tissue is not solely due to how calories are stored (adipocyte hypertrophy vs hyperplasia), but also due to where fat is stored Citation[6]. Visceral or intraperitoneal (omental, mesenteric and umbilical), extraperitoneal (peripancreatic and perirenal) and intrapelvic (gonadal/epididymal and urogenital) adipose tissues reportedly have higher metabolic activity compared with subcutaneous, peripheral adipose tissue (truncal, gluteofemoral, mammary and inguinal) Citation[6,17]. Due to differences in location and function, overweight patients with a disproportionate increase in visceral adiposity may have an increased risk of developing metabolic diseases Citation[5,18–23]. Conversely, some overweight individuals who have relatively unimpaired adipogenesis predominantly in peripheral subcutaneous adipose tissue may avoid clinically meaningful adverse adipose tissue pathogenic responses, and thus remain metabolically healthy Citation[6,18,24–27].
Although visceral adipose tissue accumulation is most often associated with metabolic disease Citation[28], other fat depots also have pathogenic potential Citation[6,19]. Adipose tissue has the potential for pathogenic responses from virtually all fat depots, such as significant immunologic abnormalities involving pericardial, perimuscular, perivascular, orbital and paraosseal adipose tissue Citation[29]. During positive caloric balance, impaired adipogenesis in subcutaneous adipose tissue may lead to increased storage of fat in other depots, resulting in visceral adipose tissue accumulation and visceral adipocyte hypertrophy, which is particularly pathogenic. Furthermore, an increase in adipocyte size and adipose tissue accumulation in the subcutaneous abdominal region is most often associated with an increased, not decreased, risk of metabolic disease Citation[6,22,30].
Free fatty acids
Circulating free fatty acid levels are determined by many factors, such as:
• Whether the patient is in a fasting (postabsorptive) state;
• The degree to which other body organs (e.g., muscle and liver) metabolize free fatty acids;
• The degree to which adipose tissue stores free fatty acids as triglycerides.
If adipocytes and/or adipose tissue are impaired in their ability to adequately store free fatty acids, then the resultant increase in circulating free fatty acids may contribute to metabolic disease. Because it is the largest fat depot (making up ≥80% of body fat), subcutaneous adipose tissue produces the majority of the free fatty acids, possibly including the majority of free fatty acids in the portal system Citation[19,31]. Nonetheless, an increase in visceral adipocyte hypertrophy and/or visceral adipose tissue often increases free fatty acid delivery to the liver through its portal drainage, which may promote hepatic ‘lipotoxicity’. The increased, lipotoxic free fatty acid delivery to the liver increases the risk of hepatic-mediated insulin resistance and dyslipidemia Citation[32,33].
In addition to its contribution to the portal system, subcutaneous adipose tissue contributes to most of the systemic circulating free fatty acids Citation[32,33], which are potentially lipotoxic to the muscle and pancreas, thus decreasing insulin sensitivity in muscle and possibly decreasing insulin secretion from the pancreas Citation[34–36]. In summary, while visceral adipose tissue accumulation is most associated with metabolic disease, both visceral and subcutaneous adipose tissue have pathogenic potential Citation[19].
Adipose tissue as an active endocrine & immune organ
Anatomically, excessive adipocyte hypertrophy (due to excessive fat content) adversely affects intracellular organelles, as evidenced by increased markers of intracellular endoplasmic reticulum stress and mitochondrial dysfunction Citation[37,38]. This supports the concept of ‘sick fat’ Citation[2,7], wherein adiposity lead to pathologic adipocyte and adipose tissue anatomic changes, which leads to pathogenic adipose tissue endocrine and immune responses, which then contribute to metabolic disease.
Adipose tissue is an active endocrine organ whose disruption can contribute to metabolic disease Citation[6,7,37,39–42]. Adipose tissue is also an active immune organ whose disruption can contribute to metabolic disease Citation[6,29,37,43–48]. Biopsy of the periumbilical subcutaneous (abdominal) region supports adiposity as increasing adipose tissue macrophage content and/or increasing markers of macrophage activation, representing potential pathogenic adipose tissue immune responses Citation[49]. Pathogenic alterations in adipose tissue endocrine and immune factors associated with adiposopathy contribute to metabolic disease, while improvements in adipose tissue endocrine and immune factors may improve metabolic disease Citation[6,23,25,50].
Interaction or ‘cross-talk’ with other body tissues
Adiposopathy alone does not cause metabolic disease. It is the interaction or ‘cross-talk’ with other body organs that substantially determines whether pathogenic adipocyte and adipose tissue endocrine and immune responses ultimately ‘cause’ metabolic disease Citation[2]. Many non-adipose tissue body organs modulate the pathogenic endocrine and immune responses from adipose tissue during positive caloric balance Citation[2]. One of the best described pathogenic responses of dysfunctional adipose tissue (‘sick fat’) is the increased net release, and thus increased circulating levels, of free fatty acids. Normally, after meals, circulating free fatty acids are substantially reduced (suppressed), due to increased insulin secretion that stimulates free fatty acid storage in adipose tissue and other body tissues. As insulin levels decrease in the hours after initial release, free fatty acid levels gradually rise Citation[51]. If, during positive caloric balance, fat weight gain results in excessive adipocyte hypertrophy and adipose tissue dysfunction, then this may result in insulin resistance and impaired storage of free fatty acids within adipocytes. The disruptive imbalance between lipolysis and lipogenesis in adipose tissue increases both fasting and postprandial circulating free fatty acids, relative to those without insulin resistance Citation[52]. If the liver is limited (‘inflexible’) in its ability to metabolize the increased delivery of free fatty acids, then intrahepatic lipid deposition (often reflected by ‘fatty liver’) may promote hepatic insulin resistance and dyslipidemia Citation[53]. Conversely, if the liver is ‘hyperflexible’, in its ability to manage the pathogenic responses of adipose tissue through genetic predisposition, or through the use of therapeutic agents (e.g., peroxisome proliferator-activated receptor [PPAR]-γ agonists), then the adverse metabolic disease consequences of increased free fatty acids may be averted Citation[2,52,54].
Similarly, some patients have an inherent or acquired impairment in the ability to metabolize intramuscular fat Citation[53]. A lack of ‘flexibility’ on the part of skeletal muscle may result in an inability to metabolize the adiposopathic increased free fatty delivery from the circulation. If so, then this may result in ectopic free fatty acid storage in muscle and the accumulation of ‘toxic’ intramyocellular lipids such as diacylglycerol, fatty acyl CoA and ceramides Citation[2]. This ‘lipotoxicity’ promotes insulin resistance in muscle Citation[55–57].
Therapeutically, hypocaloric nutritional intervention would not be expected to affect the inherent ‘flexibility’ for muscle to metabolize free fatty acids. However, such intervention would be expected to improve adipose tissue function, improve free fatty acid storage in adipose tissue, reduce circulating free fatty acids, reduce lipid delivery to skeletal muscle, and thus reduce lipid deposition in skeletal muscle. As a result, reduced fatty acid delivery and reduced lipid deposition in skeletal muscle may improve its insulin sensitivity Citation[57]. Similarly, some patients may have familial or genetic limitations in their ability to secrete insulin. The disease of Type 2 diabetes mellitus is often revealed when adiposity results in pathogenic adipose tissue endocrine and immune responses, including an increased release of free fatty acids. This exacerbates genetic limitations of the pancreas, potentially resulting in an even further impairment in insulin secretion Citation[58,59]. In addition, adipose tissue is also known to have substantial interactions and cross-talk with other body organs, such as the immune system, heart and vasculature, brain, endocrine glands, intestine, kidneys, and other body organs, all with potential physiologic and pathologic metabolic consequences Citation[2].
Adiposopathy
The goal of treating adiposity is to improve patient health. If the patient has fat mass-related morbidities, then fat weight loss may improve such conditions Citation[60]. If the patient has adiposopathy-induced metabolic disease (e.g., Type 2 diabetes mellitus, high BP and/or dyslipidemia), then fat weight loss interventions may reduce adipocyte hypertrophy, reduce visceral adiposity, improve adipocyte and adipose tissue pathogenic endocrine and immune responses, and thereby improve metabolic disease Citation[50,54].
However, reducing body fat is not the only way to improve adipose tissue function. Adding adipocyte or adipose tissue functionality is another approach. PPAR-γ agonists may improve metabolic diseases normally associated with an increase in body fat (Type 2 diabetes mellitus and dyslipidemia) by increasing adipogenesis (recruitment, proliferation and differentiation of new adipocytes) Citation[25,52]. PPAR-γ agonists illustrate how adding (functional) adipose tissue may be an effective treatment for metabolic diseases otherwise associated with adiposity and adiposopathy.
Conversely, not all weight loss interventions improve metabolic disease. Antiretroviral therapy for HIV is sometimes associated with ‘HIV lipodystrophy’, wherein weight loss is manifest by a disproportionate loss of subcutaneous adipose tissue relative to visceral adipose tissue. These HIV drug therapies may promote insulin resistance and dyslipidemia Citation[61], and illustrate how even weight loss can be associated with pathogenic adipocyte and adipose tissue dysfunction resulting in metabolic disease.
Currently, bariatric surgery appears to be the most effective intervention to reduce adiposity and adiposopathy through improvement in adipose tissue function and perhaps other mechanisms Citation[50]. Perhaps the most cost-effective treatment for adiposity and adiposopathy in the individual, willing patient is appropriate nutrition and increased physical activity Citation[2]. However, a challenge for the clinician and patient is that public health weight loss nutritional and physical activity initiatives, as well as commercial nutritional weight loss plans, have met with limited success in both individuals and populations Citation[62]. Other weight loss therapeutic options include anti-obesity drug therapies Citation[54]. Targeting weight loss is medically justified because even just a 5% weight loss in overweight patients may improve pathogenic adipose tissue responses Citation[63]. Thus, while many overweight patients may want (and sometimes expect) therapeutic interventions that result in weight loss of up to 100 lbs or more Citation[7], demonstrable and significant metabolic benefits can be achieved with ‘only’ a 5–10% reduction in body weight Citation[64,65].
Weight loss challenges
Unfortunately, despite available therapeutic options, genetic, physiologic, environmental and behavioral factors impede effective management of the unabated obesity epidemic Citation[201].
One potential physiologic impediment may be related to adipogenesis. Physiologically, during fat weight loss, a reduction in adipogenic markers does not necessarily reflect adipocyte dysfunction Citation[23,50]. Instead, it is physiologically appropriate that adipogenic processes diminish when the need for additional adipocytes and adipose tissue is diminished, as occurs during active weight loss. However, the potential for adipogenesis may be increased, as evidenced by enhanced preadipocyte adipogenic and anti-apoptotic protein expression Citation[66,67]. An increase in adipogenic potential may be a contributing physiologic cause for the increased potential for all-too-common fat regain among those who lose body fat.
Another important factor contributing to the lack of persistence with weight loss is that the human body is not very efficient in self-regulating against excessive energy storage. Conversely, the human body is often entirely too efficient in self-regulating against too little available energy through multiple redundant mechanisms intended to prevent starvation. It appears that conserving energy during a negative caloric balance represents a more urgent priority for survival than expending energy during positive caloric balance. While this may be advantageous in environments of food scarcity and when high physical activity is necessary for survival, it is potentially disadvantageous when food and transportation conveniences are readily available.
The major components of total daily energy expenditure include resting energy expenditure, non-resting energy expenditure (e.g., physical activity and exercise) and the thermal effect of food Citation[68]. Data suggest that having a low resting metabolic rate (i.e., having low resting energy expenditure) is not a major ‘cause’ of obesity in most patients, despite the common belief otherwise Citation[69]. Instead, resting energy expenditure is largely dependent upon the BMI. While individual variabilities exist, formulae that include BMI (and age), such as the Harris–Benedict equation, yield reasonable mean resting energy expenditure predictions for normal and obese individuals Citation[70]. Thus, in those without secondary causes of adiposity, patients with higher BMI have higher resting energy expenditure, while patients with lower BMI have lower resting energy expenditure. During dynamic weight loss (negative caloric balance), the resting metabolic rate often decreases below what is appropriate for BMI, and may Citation[71] or may not Citation[72] return to what is appropriate for BMI shortly upon stabilization of body weight and a return to energy balance. Non-resting energy expenditure may also decrease during weight loss due to improved skeletal muscle work efficiency Citation[73]. Whatever degree of conservation of energy occurs during dynamic weight loss, diminished total energy expenditure limits fat weight reduction during times of negative caloric balance, which is a limitation perhaps mitigated by engaging in routine physical exercise Citation[74].
An example of a neuroendocrine mechanism that helps account for this conservation involves leptin. Leptin is an adipocyte-released cytokine whose blood levels increase with adipocyte size and number. In obese patients with leptin deficiency, leptin administration reduces food intake and can result in dramatic weight loss Citation[75,76]. However, most evidence suggests leptin approximates its maximum physiological effects at the high end of normal physiologic levels Citation[5]. Thus, in those without leptin deficiency, the supraphysiologic levels of leptin found with obesity in humans do not effectively counteract persistent or worsening obesity. However, during weight loss, leptin levels decrease and low leptin levels can significantly favor positive caloric balance through increased appetite, among other possible mechanisms Citation[77]. Increased physical activity, such as through increased physical exercise, may enhance leptin sensitivity Citation[78], reduce the so-called ‘leptin resistance’ Citation[79], and better allow for improved longer term weight loss maintenance Citation[25]. Thus, in addition to improving cardiovascular health Citation[80], preserving lean body mass Citation[81,82] and potentially improving metabolic parameters during weight loss Citation[83], increased physical activity, such as through routine physical exercise, may also favorably affect leptin activity. This represents yet another potential justification for recommending increased physical activity, along with appropriate nutrition, towards the goal of maximizing chances of successful and persistent weight loss.
Physiological barriers such as these help to account for the frequent failure of nonsurgical weight loss interventions and a high rate of fat regain among overweight patients who have lost weight. Although not always effective Citation[84], a pharmaceutical approach to overcome these multiple barriers is through combining weight loss drug therapies having complementary mechanisms of action, thereby potentially affecting more than one physiologic process Citation[23]. Several combination anti-obesity strategies are in development Citation[3]. An example of one such complementary approach is the combined use of phentermine and topiramate. The remainder of this review will focus on how both these agents, alone and in combination, reduce body weight, improve adipocyte and adipose tissue function (as described previously), and improve metabolic disease.
Phentermine
Indication & history
Phentermine is an approved anti-obesity agent indicated as an adjunct to appropriate nutrition and physical exercise for short-term (up to 12 weeks) treatment of obesity. Significantly due to its generic status, phentermine is the most commonly prescribed prescription appetite suppressant Citation[3]. Phentermine resin was first approved by the US FDA in 1959 Citation[85]. Phentermine resin formulations allow for a slower gastrointestinal release after ingestion. Administration of phentermine resin often begins with a 15 mg dose, titrated to 30 mg per day if needed.
In the 1970s, phentermine hydrochloride (HCl) was developed, with (depending upon the manufacturer) doses ranging from 8 to 37.5 mg, which is generally equivalent to 6.4–30 mg of phentermine resin. The phentermine HCl salt easily dissociates in the GI tract, resulting in immediate-release of phentermine drug; phentermine HCl is absorbed from the GI tract approximately three-times faster than phentermine resin Citation[86]. Theoretically, immediate-release phentermine HCl has a more intense appetite-suppressant effect compared with phentermine resin, but for a shorter duration of time.
Mechanism of action
Anti-obesity therapies promote weight loss generally through decreased gastrointestinal absorption of energy (e.g., orlistat Citation[3] and some bariatric surgeries Citation[50]), increased energy expenditure and/or decreased energy (caloric) intake. Thyroid hormone is a classic example of an orally administered agent that increases energy expenditure through its CNS and systemic effects Citation[87–90]. As perhaps the first anti-obesity agent, thyroid hormone was used as early as 1893 to induce weight loss Citation[87]. However, excessive use of thyroid hormone has toxic effects upon the CNS and multiple other body organs. Dextrothyroxine was one of the five treatment groups evaluated in the Coronary Drug Project. The thyroid treatment arm in this study was stopped due to excessive mortality Citation[91]. Toxicity due to excessive thyroid hormone is why the product information has a black warning against the use of thyroid hormone for weight loss Citation[87].
Similarly, amphetamines were used as early as 1938 as weight loss treatments Citation[92]. The mechanism of action of amphetamines includes an increase in CNS dopamine and norepinephrine (both catecholamines), and serotonin (an indolamine) activity. Clinical trials in humans suggest sympathomimetic agents increase energy expenditure Citation[93,94]. In animals, amphetamines are reported to increase energy expenditure Citation[95], such as through increasing thermogenesis Citation[96]. Other animal data suggest that while amphetamines may increase locomotor activity, they may suppress the overall resting metabolic rate Citation[97]. Although one might suppose that amphetamines (like other stimulants) increase the metabolic rate, the reported human data are remarkably scarce regarding the effect of amphetamines on energy expenditure. One study suggested that dextroamphetamine or methylphenidate treatment of children with attention-deficit hyperactivity disorder tended to increase the resting metabolic rate but decrease physical activity, resulting in an overall decrease in energy expenditure. Thus, at least at doses typically prescribed for weight loss in obese patients, the long-term effects of amphetamines on total energy expenditure are unclear. What does seem to be clear is that administration of these monoamine neurotransmitters reduces appetite, as would be expected through their decreases in neuropeptide Y Citation[98], increases in pro-opiomelanocortin Citation[99] and increases the anorexigenic peptide located in the hypothalamus, termed ‘cocaine and amphetamine-regulated transcript’ (CART) Citation[100], among other potential effects . As with thyroid hormone, amphetamines have potential toxic CNS and other adverse systemic effects, including an increase in BP, tachycardia and euphoria. Amphetamines also have a high potential for abuse and addiction Citation[87].
In the USA, the authority to prescribe certain controlled substances is defined by Drug Enforcement Agency (DEA) scheduling. Schedule I controlled substances include drugs with a high potential for abuse and with no accepted medical use, such as heroin, peyote and others. Most physicians do not have a medical need to apply for the special permission required to prescribe Schedule I drugs. DEA Schedule II drugs include those with a high potential for abuse, but which have medical use. Common examples include cocaine (for topical use), methadone, morphine and amphetamines. Therapeutic forms of amphetamines include amphetamine, dextroamphetamine and methamphetamine. Depending upon the preparation, amphetamines have an indicated use to treat attention-deficit disorder and narcolepsy. Some amphetamines continue to have a regulatory indication to treat obesity, although they are rarely used for this purpose. Instead, the product information for amphetamines usually has a black box warning regarding the high potential for abuse, and risk of sudden death and serious cardiovascular events.
Phentermine is commonly described as a noradrenergic and possibly dopaminergic Citation[101] sympathomimetic amine, although some question whether phentermine causes clinically meaningful release of dopamine from central neurons, at least relative to amphetamine Citation[102]. Phentermine is chemically related to amphetamine, but does not have its addictive potential. Phentermine is listed as a Schedule IV drug (along with other drugs such as barbiturates, benzodiazepines and librium) because it has a low potential for abuse, and because it has a medical use for short-term treatment of obesity. describes first- and second-order hypothalamic signaling, which may be influenced by increased CNS noradrenergic activity Citation[103], as might be promoted by phentermine. While phentermine increases sympathetic activity (which reduces appetite), little evidence suggests that its weight loss effects are substantially due to increasing resting energy expenditure Citation[104].
Adverse experiences
Phentermine is contraindicated where sympathomimetic drug administration may pose a significant risk, such as in patients with unstable cardiovascular disease, moderate-to-severe high BP, hyperthyroidism and unstable cardiac dysrhythmias. Phentermine is not recommended in patients treated with monoamine oxidase inhibitors or in patients with a history of drug abuse (including excessive alcohol consumption). Phentermine should be used with caution in patients concurrently treated with other agents that affect the CNS agents, such as agents that increase adrenergic responses (i.e., decongestants). Phentermine’s side effects include palpitations, tachycardia, increase in BP, tremor, diaphoresis, overstimulation, anxiety, irritability, restlessness, dizziness, insomnia, euphoria, dysphoria, headache, dryness of the mouth and gastrointestinal complaints (i.e., diarrhea and constipation) Citation[3].
Phentermine, adiposopathy & metabolic disease
Although phentermine is among the oldest approved anti-obesity agents, it has among the least data with respect to its effects upon adiposopathy. Limited to no published data exist on phentermine monotherapy effects upon adipogenesis, free fatty acids and important adipose tissue endocrine and immune factors . Shorter term data suggest that the weight loss associated with phentermine may have some favorable lipid effects Citation[105], with variable effects regarding BP Citation[105,106]. However, published data on the long-term (≥1 year) effects of phentermine monotherapy on metabolic disease are lacking.
Topiramate
Indication & history
Topiramate is a sulfamate-substituted monosaccharide derivative of the naturally occurring sugar monosaccharide D-fructose. It was originally developed as a treatment for Type 2 diabetes mellitus Citation[60,107]. Despite promising early animal data, the direct antihyperglycemic effects of topiramate (independent of weight loss) were not confirmed in humans Citation[108]. However, some authors continue to believe topiramate has insulin secretagogue and insulin sensitization potential Citation[109].
Topiramate has undergone evaluation and development at least since 1979. Topiramate was approved in the USA as a marketed pharmaceutical in 1996 Citation[110]. Topiramate has a US regulatory indication for prophylaxis of migraine headaches and treatment of seizure disorders. Most applicable to this review is that while topiramate does not have a regulatory indication for weight loss Citation[111], some clinicians have used topiramate ‘off label’ for the purpose of weight reduction. This is because early on, even at the time of approval, the treatment emergent ‘adverse’ experiences described in its development reportedly included: “mild, dose-related weight loss was associated with topiramate therapy” Citation[112]. Since approval, clinical trial data have supported topiramate as having weight loss effects Citation[113–119].
An illustrative study was a placebo-controlled, 6-month clinical trial of topiramate (not controlled-release) in obese subjects starting with 16 mg once a day, which was increased to 16 mg twice a day, and then increased weekly by 16 mg twice a day until reaching the target dose of 64, 96, 192 or 384 mg per day Citation[116]. Mean weight loss from baseline for placebo was -2.6%. At the respective doses of topiramate, weight loss was -5.0, -4.8 and -6.3%. This suggested that most of the weight loss with topiramate occurred at the lower topiramate doses. A total of 21% of topiramate subjects withdrew because of adverse experiences (compared with 11% of placebo-administered patients). According to the authors: “The most frequent adverse events were related to the central or peripheral nervous system, including paresthesia, somnolence, and difficulty with memory, concentration, and attention. Most events were dose-related, occurred early in treatment, and usually resolved spontaneously” Citation[116].
Another study evaluated the long-term effects of topiramate (again, not controlled-release) in obese subjects participating in a nonpharmacologic weight-loss program Citation[114]. Topiramate produced significant weight loss of -7.0, -9.1 and -9.7% at respective doses of 96, 192 and 246 mg per day, compared with a loss of -1.7% in the placebo group. As before, most of the weight loss was achieved at the lower topiramate doses. Importantly, topiramate significantly improved BP, glucose and insulin levels. Regarding safety and tolerability, the authors stated: “The most common adverse events more frequently observed in topiramate-treated subjects occurred mostly during the titration phase and were related to the central or peripheral nervous system and included paresthesia, difficulty with concentration/attention, depression, difficulty with memory, language problems, nervousness, and psychomotor slowing.” The study was stopped early because the study sponsor elected to: “develop a new controlled-release formulation with the potential to enhance tolerability and simplify dosing” Citation[114]. However, this controlled-release monotherapy development program was later abandoned, presumably because of a lack of consistent benefits and sufficient efficacy, balanced against the adverse experiences found with higher topiramate monotherapy doses thought to be required to obtain an approvable weight-loss indication Citation[60].
Mechanism of action
Topiramate is a ‘neurostabilizer’ Citation[107]. Signaling from CNS neuron to neuron is propagated by action potentials, which result from ion (i.e., sodium and potassium) flux across voltage-gated ion channels in neuron cell walls. Seizures occur when excessive firing disrupts the normal neural signaling flow from postsynaptic depolarization, to action potential generation, to neurotransmitter release Citation[120]. Although not yet definitively proven, topiramate may reduce seizure activity by modifying excitatory voltage-activated sodium and calcium channels, antagonizing α-amino-3-hydroxy-5-methyl-4-isoxazole-propionic acid kainate (AMPA/KA) receptors, and enhancing γ-aminobutyric acid (GABA) receptor-mediated inhibitory currents Citation[121].
Similarly, although yet to be proven, topiramate has several potential mechanisms accounting for weight loss. As it is a CNS-acting drug, topiramate may have CNS effects that alter caloric balance. Topiramate’s antagonism of AMPA/KA receptors may reduce compulsive or addictive food cravings Citation[122], which is supported by topiramate’s effectiveness in improving binge eating disorder Citation[123] and effectiveness in reducing other addictive behaviors Citation[124]. Topiramate’s activation of GABA receptors may decrease night-time and deprivation-induced feeding Citation[125]. Decrease feeding during caloric deprivation would favor persistence of weight loss. While animal studies suggest topiramate may increase levels of neuropeptide Y (NPY) in the hypothalamus (an effect that would seem to promote positive caloric balance Citation[126,127]), topiramate may also increase levels of hypothalamic corticotropic-releasing hormone, which may be catabolic Citation[128]. Topiramate may also increase hypothalamic galanin, with unclear body weight implications Citation[128,129]. While some studies suggest leptin levels decrease with topiramate-induced weight reduction Citation[119], other reports suggest leptin levels may either not significantly decrease, or only modestly decrease with topiramate-induced weight loss, which is another effect that may favor persistence of weight loss Citation[130]. Anorexia is commonly reported with topiramate; however, topiramate may not reduce objective measures of appetite during weight loss Citation[131]. A lack of increased hunger and appetite during weight loss may reflect a cumulative effect of the anorectic effects of topiramate, balanced against the orexigenic effect of topiramate-promoted fat loss or related energy deficit through other mechanisms Citation[131]. Again, this would favor persistence of weight loss. Regarding energy expenditure, topiramate may decrease energy storage and usage efficiency, and thus increase energy expenditure Citation[132]. Topiramate may increase thermogenesis Citation[133].
Applicable to adipocyte function, topiramate (as well as related sulfamates) may inhibit adipocyte mitochondrial carbonic anhydrase isozyme V Citation[134], inhibiting carbonic anhydrase-mediated lipogenesis Citation[135,136]. Topiramate is also associated with decreased lipoprotein lipase activity in white adipose tissue, which would limit free fatty acid substrate for lipogenesis Citation[137]. Conversely, topiramate may increase lipoprotein lipase activity in brown adipose tissue, which may represent increased thermogenesis and increase lipoprotein lipase activity in skeletal muscle, further supporting the potential for substrate oxidation Citation[137]. Another effect on the level of the adipocyte is that topiramate may increase adiponectin levels Citation[138], which favorably affects many peripheral physiologic processes relevant to metabolic disease Citation[139]. While the clinical significance is unclear, increased circulatory adiponectin may have CNS effects regarding energy balance Citation[140].
Finally, clinical trials support topiramate as altering taste, which could conceivably affect caloric intake Citation[141], and topiramate is a carbonic anhydrase inhibitor, which may have anorexigenic effects (see later). Overall, topiramate has multiple potential mechanisms that could promote both weight loss, and persistence of weight loss.
Adverse experiences
Many of the adverse experiences reported with topiramate are dose-related Citation[112]. As with some other sulfa-based drugs (e.g., acetazolamide, hydrochlorothiazide and cotrimoxazole) topiramate can cause acute myopia, sudden onset of blurring of vision, redness of the sclera, photophobia and discomfort of the eyes resulting from secondary angle-closure glaucoma Citation[142,143]. Angle-closure glaucoma occurs when the aqueous humor produced in the anterior chamber (the space between the iris/lens and cornea) by ciliary processes is unable to be drained through the trabecular meshwork due to closure of the angle between the iris and the cornea. The increased intraocular pressure may adversely affect various portions of the eye, such as the cornea, sclera, iris, lens and optic nerve. This potential adverse experience usually occurs within hours to weeks after topiramate initiation. Treatment involves stopping the topiramate Citation[144]. The increased risk of secondary angle-closure glaucoma with topiramate is in contrast to primary angle-closure glaucoma, wherein treatment may include procedures such as iridoplasty or medications such as acetazolamide Citation[145]. Acetazolamide may improve glaucoma by inhibiting carbonic anhydrase isozymes in the ciliary processes of the eye, reducing bicarbonate and aqueous humor secretion, and thus reducing the elevated intraocular pressure Citation[146]. This is somewhat paradoxical, given that topiramate, like acetazolamide, is an inhibitor of carbonic anhydrase, and suggests that the secondary angle-closure glaucoma with topiramate is not related to its inhibition of carbonic anhydrase. Finally, the commonality of carbonic anydrase inhibitors helps explain why both acetazolamide and topiramate may be effective in treating conditions such as idiopathic intracranial hypertension Citation[147].
However, while topiramate-associated glaucoma may not be related to mild inhibition of carbonic anhydrase, other adverse experiences may be related to this effect. Carbonic anhydrase is a ubiquitous family of metalloenzymes that catalyze carbon dioxide and water to bicarbonate and protons. In human metabolism, nutrients (e.g., carbohydrates, protein and fats) are metabolized by body tissues to generate sources of energy (e.g., adenosine triphosphate), water and carbon dioxide. Carbonic anhydrase enzymes help transport carbon dioxide by converting tissue carbon dioxide to bicarbonate, which is then carried by red blood cells to the lungs, where pulmonary carbonic anhydrase converts the bicarbonate back to carbon dioxide, which then undergoes respiratory exchange for oxygen. The types of topiramate adverse experiences most likely due to carbonic anhydrase inhibition include metabolic acidosis Citation[148], increased risk of kidney stones Citation[149] and oligohidrosis (that generally reverses after stopping topiramate), which may contribute to hyperthermia (mostly described in children) Citation[150]. Other adverse experiences that may be related to carbonic anhydrase inhibition include an increase in paresthesias, which is among the most common adverse experiences associated with topiramate, as with other carbonic anhydrase inhibitors Citation[151]. Additional adverse experiences sometimes associated with topiramate as well as other carbonic anhydrase inhibitors include anorexia, fatigue, somnolence and depression Citation[152].
Special mention should be made of the potential CNS adverse experience of topiramate, which, as noted before, include difficulty with concentration, difficulty with memory and depression Citation[117]. These potential adverse experiences are important with any drug affecting the CNS, and particularly important with regards to anti-obesity drug development. Rimonabant is a cannabinoid receptor 1 (CB1) antagonist that underwent extensive, if not comprehensive, clinical trial evaluation. Rimonabant was not only effective in generating weight loss, but also improved adiposopathy and many metabolic diseases, such as dyslipidemia and Type 2 diabetes mellitus Citation[153]. However, rimonabant never obtained US regulatory approval because the FDA was more concerned about the potential for CNS adverse experiences than it was impressed by the numerous and significant improvements in metabolic disease – suggesting that the current criteria for anti-obesity drug development may need to be re-evaluated Citation[154]. Paramount in the termination of rimonabant and several other CB1 antagonist development programs was a modest increase in depression and anxiety Citation[155].
Because topiramate is a CNS-acting drug, and is being considered as a component of an anti-obesity agent, an understanding of its CNS effects becomes paramount. Publication of potential suicidality associated with topiramate is basically limited to case reports Citation[156]. Because the frequency of suicide with individual anticonvulsants was not sufficient for proper statistical analyses, in 2008 the FDA mandated a warning labeling for many anticonvulsant medications regarding the increased risk of suicidal thoughts and behaviors, which included labeling applicable to topiramate. A subsequent cohort study of a large database including 297,620 new episodes of treatment with an anticonvulsant agent identified 26 completed suicides, 801 attempted suicides and 41 violent deaths. This analysis was not designed to determine if the anticonvulsants caused, or contributed, to suicides, or if these findings were simply incidental to the use of the anticonvulsants. Topiramate was chosen as the reference drug in this study. This exploratory analysis suggested that gabapentin, lamotrigine, oxcarbazepine and tiagabine were associated with an increased risk of suicidal acts or violent deaths compared with topiramate Citation[157]. This might be interpreted as suggesting topiramate had the least potential to cause suicides as compared with the other approved and commonly prescribed anticonvulsant therapies. Finally, it is noteworthy that this study evaluated topiramate (not controlled-release) at doses typically used for anticonvulsant therapy.
Topiramate, adiposopathy & metabolic disease
Although topiramate does not have an indication for weight loss, it is interesting that topiramate appears to have more published data than phentermine (which does have an indication for weight loss) with respect to parameters associated with adiposopathy. While the effects upon adipogenesis are unknown, the medical literature does report data suggesting that topiramate has effects upon adipose tissue factors that would generally be considered favorable in preventing, or improving, metabolic disease .
Phentermine & topiramate controlled-release combination
Rationale & history
Common themes in the development of pharmacologic therapies for metabolic diseases (e.g., obesity, diabetes mellitus, hypertension and dyslipidemia) include:
• Drug treatment is intended to be an adjunct to appropriate nutrition, increased physical activity and other favorable lifestyle interventions;
• Monotherapy of any single therapeutic agent is often insufficient to achieve desired treatment goals;
• Compared with either agent alone, combining different therapeutic agents with complementary mechanisms of action may have greater efficacy at lower doses of each;
• Compared with either agent alone, lower doses of the constituent drug therapies may improve upon the intolerances and toxicities associated with higher doses.
The phentermine and topiramate controlled-release (PHEN/TPM CR; VI-0521; Qnexa®, Vivus, Inc., CA, USA ) development program was consistent with this approach. PHEN/TPM CR clinical trials were designed to evaluate the efficacy and safety of the combined use of lower doses of two different drugs with differing mechanisms of action for the purpose of weight reduction (e.g., reducing appetite and increasing satiety) over a 24-h period. By utilizing a more-than-one treatment target approach, the hope was to counteract redundant physiologic mechanisms that often limit fat weight loss and frequently promote fat weight regain in overweight patients.
The PHEN/TPM CR development program involved a once-a-day, co-formulated combination single capsule of phentermine HCl (which is readily dissolved and absorbed in the GI tract to provide therapeutic effects early in the day), added to controlled-release topiramate (to provide persistent therapeutic weight loss effects throughout the day). As noted before, the maximum approved dose of phentermine monotherapy, as found with the slower release resin formulation, is 30 mg per day, which is roughly equivalent to 37.5 mg of the immediate-release phentermine HCl. The maximum approved dose of topiramate monotherapy is 400 mg per day. In its development program, PHEN/TPM CR evaluated three combination doses. ‘Low dose’ was defined as phentermine HCl 3.75 mg/topiramate CR 23 mg; ‘mid dose’ was phentermine HCl 7.5 mg/topiramate CR 46 mg; and ‘full dose’ was phentermine HCl 15 mg/topiramate CR 92 mg. Thus, across the dose ranges, the PHEN/TPM CR combination agent contained 40% or less of the maximum approved dose of phentermine HCl (maximum dose phentermine HCl monotherapy = 37.5 mg per day), and less than 25% of the maximum approved dose of topiramate (maximum topiramate monotherapy dose = 400 mg per day). Finally, the clinical trial development program utilized a titration dosing schedule for the first 4 weeks.
A full discussion of the results of the clinical trials in the PHEN/TPM CR development program awaits peer-reviewed published data. However, much of the PHEN/TPM CR clinical trial data were presented as poster and podium abstracts at major scientific meetings. The study sponsor also issued press releases supplementing the scientifically presented data. Study sponsor press releases are generally good at reporting basic study details (as described herein), at least in part due to security regulations and requirements Citation[158]. However, press releases may be incomplete regarding nuanced study limitations that may be more fully vetted through peer review during the publication process Citation[158]. The same might be said about scientific abstracts. With these caveats in mind, summarizes preliminary disclosed data regarding some of the sentinel Phase III clinical trials of the PHEN/TPM CR combination agent.
Mechanism of action
The mechanism of action of PHEN/TPM CR would be expected to reflect the mechanism of action of the individual components previously described.
Adverse experiences
lists adverse experiences (regardless of cause) that occurred in over 5% of study participants in the two largest trials to date. Dry mouth and paresthesias (‘tingling’) occurred in approximately 20% of PHEN/TPM CR full-dose-administered subjects, with other adverse experiences occurring in less than 20% of study participants (e.g., constipation, altered taste, insomnia, headache, dizziness, nausea and blurred vision). However, from a persistence-of-use standpoint, it is often not the quantity or frequency of adverse experiences associated with a pharmaceutical, but rather the quality (degree) or severity of adverse experiences that is most clinically relevant. Many commonly prescribed drugs have a high frequency of adverse experiences that are not severe enough to negate their clinical utilization. The product information (PI) of amoxicillin/clavulanate potassium Citation[202] describes a 9% frequency of diarrhea/loose stools, with a warning of: “serious and occasionally fatal hypersensitivity (anaphylactic) reactions” in patients treated with penicillin. The PI of ibuprofen Citation[203] describes a 4–16% frequency of gastrointestinal complaints with a warning that: “serious gastrointestinal toxicity such as bleeding, ulceration and perforation can occur at any time, with or without warning symptoms.” The PI of metformin Citation[204] describes a 53% frequency of diarrhea, 26% frequency of nausea/vomiting, 12% frequency of flatulence and a warning of potential lactic acidosis that: “when it occurs, it is fatal in approximately 50% of cases.” The point is that simply having associated adverse experiences is not enough to prevent the clinical use of a drug. Instead, the degree of severity should be considered when determining the clinical importance of the adverse experiences associated with a pharmaceutical treatment.
provides data that somewhat reflect the severity of these adverse experiences through representing the percent of adverse experiences resulting in study discontinuation. The discontinuation rate due to adverse experiences associated with placebo was 9%, while the discontinuation rate due to PHEN/TPM CR low, mid and full dose was 12, 12 and 18%, respectively, with no particular adverse experience being an outlier causing disproportionate study discontinuation. Despite the modest increase in discontinuations due to adverse experiences in the PHEN/TPM CR group, it is interesting that and report a higher study completion rate in those in the PHEN/TPM CR group compared with the placebo group, particularly with the mid-to-full PHEN/TPM CR dose. This suggests that overall, more in the placebo group discontinued from the trials than the PHEN/TPM CR groups, due to reasons other than adverse experiences. One might speculate that the higher completion rate in the PHEN/TPM CR group might, at least in part, be due to the greater weight loss efficacy of PHEN/TPM CR over placebo, resulting in a greater inclination to remain in the studies. It is also possible that a topiramate-induced reduction in food-addictive behavior (as previously discussed) may have enhanced adherence and completion of study protocols. Finally, CNS stimulant medications may improve retention of previously required information, facilitate memory consolidation and conceivably have motivational effects that might affect clinical trial completion. However, previously published data that might support such effects typically did not include phentermine, are inconsistent and mostly studied in patients with attention-deficit hyperactivity disorder. Furthermore, while CNS stimulants might conceivably have the aforementioned effects, the evidence does not always support stimulants as being cognitive ‘enhancers’ Citation[159].
One of the main challenges of anti-obesity drug development, particularly from a regulatory perspective, is the effect of CNS-acting anti-obesity agents upon adverse experiences such as anxiety and depression. As opposed to several other development programs, study subjects in some of the PHEN/TPM CR trials were permitted to have mild depression, as long as their antidepressant therapy was stable prior to study entry. Preliminary reports suggest that PHEN/TPM CR does not significantly increase moderate or severe depression as measured by depression scales such as the Patient Health Questionnaire (PHQ) – 9. Other scales, such as the Columbia Suicide Severity Rating Scale reportedly demonstrated no increase in suicidality Citation[205].
PHEN/TPM CR, adiposopathy & metabolic disease
lists the effects of PHEN/TPM CR upon adipocyte and/or adipose tissue factors thought to contribute to metabolic disease. Most have yet to be evaluated or reported. describes the weight loss and improvement in metabolic diseases of PHEN/TPM CR in three sentinel clinical trials.
The 6-month EQUATE trial evaluated 756 generally healthy obese subjects with a BMI between 30 and 45 kg/m2. PHEN/TPM CR mid- and full-dose combination therapies significantly reduced body weight over the same doses of phentermine and topiramate monotherapies Citation[160]. Diabetes mellitus was an exclusion criterion. Nonetheless, a subset analysis demonstrated that both mid- and full-dose PHEN/TPM CR reduced hemoglobin A1c (HbA1c) Citation[6,161,206]. The most common adverse experiences for placebo, PHEN/TPM CR mid dose and PHEN/TPM CR full dose, respectively, were tingling (3, 16 and 23%), dry mouth (0, 13 and 18%), headache (13, 15 and 16%) and constipation (8, 7 and 15%) Citation[207].
The 1-year EQUIP study evaluated 1267 generally healthy obese subjects with a BMI of 35 kg/m2 or more. PHEN/TPM CR low and full dose significantly reduced body weight compared with placebo. Despite having minimal or drug-controlled metabolic abnormalities at baseline, PHEN/TPM CR low dose significantly reduced systolic BP and total cholesterol. PHEN/TPM CR full dose significantly reduced systolic BP, reduced diastolic BP, reduced triglycerides (TGs), reduced total cholesterol, reduced LDL cholesterol (LDL-C), and increased HDL (HDL-C) levels Citation[205]. The significant reduction in total body weight, the reduction in waist circumference, and the significant improvement in metabolic parameters compared with placebo supported PHEN/TPM CR as being effective in treating adiposopathy and its adverse metabolic consequences.
The 1-year CONQUER trial included 2487 obese patients with a BMI of 27 kg/m2 or more and common metabolic comorbidities associated with adiposopathy. This study demonstrated that PHEN/TPM CR significantly reduced body weight, and reduced waist circumference (an anatomical manifestation of adiposopathy) compared with placebo. In addition, PHEN/TPM CR mid dose significantly reduced BP, TG and total cholesterol, and increased HDL-C levels. PHEN/TPM CR full dose significantly reduced systolic BP, reduced diastolic BP, reduced TG, reduced total cholesterol, reduced LDL-C and increased HDL-C levels. Among the subset of patients with Type 2 diabetes mellitus upon study entry, both the mid- and full-dose PHEN/TPM CR significantly reduced HbA1c, fasting glucose, insulin levels (after an oral glucose tolerance test), and the homeostatic model assessment of insulin resistance Citation[162,205]. Among the subset of patients with high BP at study entry, both the mid- and full-dose PHEN/TPM CR significantly reduced systolic BP. Among the subset of patients with hypertriglyceridemia at study entry, both mid- and high-dose PHEN/TPM CR significantly reduced TG levels Citation[205].
A trial not listed in was a 56-week, randomized, double-blind, placebo-controlled trial of PHEN/TPM CR full dose in 130 obese subjects (BMI 27–45 kg/m2) with drug-naive Type 2 diabetes mellitus, or Type 2 diabetes mellitus treated with oral antihyperglycemic drugs Citation[163,208]. Patients with stable depression were eligible, as were those treated with selective serotonin reuptake inhibitors (SSRIs) or serotonin/norepinephrine reuptake inhibitors (SNRIs). Baseline mean age was 50 years, 90 were women, 40 were men, and over 90% of subjects completed the trial. PHEN/TPM CR produced a 9.4% reduction in body weight (vs a reduction of 2.7% with placebo), and a reduction in HbA1c of 1.6% (vs reduction of 1.1% with placebo). The high degree of HbA1c reduction with placebo was due to the increase in anti-diabetes mellitus therapies utilized in the placebo group during the trial versus a net decrease in anti-diabetes mellitus therapies utilized in the PHEN/TPM CR group. In addition to improvements in fasting and postprandial glucose levels, PHEN/TPM CR also resulted in a decrease in waist circumference, BP and triglyceride levels.
Taken in totality, these studies support PHEN/TPM CR as not only effective in improving the weight of patients, but also effective in improving the metabolic health of patients.
Conclusion
The PHEN/TPM CR development program involved the combined use of two pharmaceuticals known to have weight loss properties and complementary mechanisms of action. Its components were at doses lower than approved for their monotherapy treatment indications. This helps account for why the reported adverse experiences with PHEN/TPM CR were not severe enough to result in substantial discontinuance of drug therapy. In fact, the PHEN/TPM CR had higher persistence of use than the placebo group. Most importantly, PHEN/TPM CR improved adiposopathy, as evidenced by the significant loss of body weight, reduction in waist circumference and improvement in multiple metabolic parameters. This all supports PHEN/TPM CR as not only improving the weight of patients, but also improving the health of patients.
Expert commentary & five-year view
In early to mid-2010, only two anti-obesity drugs were approved for long-term use in the USA (i.e., orlistat and sibutramine). In early 2010, sibutramine was no longer approved in Europex Citation[3,23,164], leaving only orlistat. Obesity is the greatest epidemic in human existence, based upon the number of lives affected Citation[23]. Thus far, regulatory agencies have determined that the risks of all but one or two anti-obesity drug therapies exceed their potential benefits in treating adiposity, adiposopathy and metabolic disease.
The history of anti-obesity drug development has often been ‘challenging’ at best, and disastrous at worst Citation[23,87]. Adverse safety experiences with previous anti-obesity drugs may have tempered, and perhaps even jaded regulatory enthusiasm for approving future anti-obesity agents Citation[87]. Hopefully, a ‘new age’ in anti-obesity drug development will result from the increased recognition of adipose tissue being far more than simply an inert storage organ. Adipose tissue is an active endocrine and immune organ whose dysfunction promotes the most common metabolic diseases encountered in clinical practice (e.g., diabetes mellitus, high BP and dyslipidemia) Citation[5]. As such, the next 5–10 years may represent a renaissance in what was known in the 1940s to 1970s, which is that adipocytes Citation[14,15] and adipose tissue Citation[28] are potentially pathogenic. In other words, recognizing adipocyte hypertrophy and visceral adiposity as contributing, or in fact ‘causing’, metabolic disease is not a novel concept Citation[6]. It is certainly not novel to clinicians who, in the day-to-day management of patients, frequently observe onset or worsening of glucose, BP and lipid levels in their patients who gain body fat.
Towards the goal of ‘rediscovering’ the pathogenic metabolic potential of adipose tissue in human disease, the terms ‘adiposopathy’ and ‘sick fat’ as scientific and clinical terms, respectively, may afford advantages over older, less meaningful terms. ‘Obesity’, ‘metabolic syndrome’ and ‘ectopic fat’ are examples of terms that may soon become obsolete, or at least acquire different meanings than intended today. For example, if it is to continue to describe a ‘disease’ at all, the term ‘obesity’ may eventually refer to body-mass pathology alone Citation[23]. The potential of adipose tissue to contribute to metabolic disease is better assessed based upon its pathogenic endocrine and immunological responses, relative to a simple measurement of body weight Citation[17]. Likewise, the term ‘metabolic syndrome’ remains problematic for many reasons; most of all because it was never intended to represent or describe a unified pathophysiologic process Citation[1,165]. Broken down to its basic components, ‘metabolic syndrome’ is nothing more than a collection of common metabolic abnormalities that tend to cluster and which are CHD risk factors Citation[6]. The National Education Program, Adult Treatment Panel III includes increased waist circumference as one of the five components of defining ‘metabolic syndrome’. The International Diabetes Federation goes further in recognizing the pathogenic importance of pathogenic adipose tissue by requiring increased waist circumference as being present to meet the definition of the ‘metabolic syndrome’, which must then be accompanied by other metabolic abnormalities. These definitions provide implicit recognition of the pathogenic potential of adipose tissue with respect to promoting metabolic abnormalities and increasing CHD risk. Thus, one might argue that the term ‘metabolic syndrome’ might best be replaced by a term that better describes the underlying cause of the clustering of common atherosclerotic risk factors. ‘Adiposopathy’ is such a term Citation[1,2].
A third term that may be destined for evolution or dissolution is ‘ectopic fat’. Adipose tissue has biologic and physiologic importance, such as by providing heat insulation, mechanical cushion, endocrine functions, immune functions and storage of energy. Thus, the presence of adipose tissue is important for metabolic health; the absence of body fat is pathologic Citation[6]. Ectopic fat describes the situation wherein existing adipose tissue is no longer able to efficiently store excess energy. Fats (free fatty acids, triglycerides, etc.) are inappropriately transported and stored in body organs, increasing the risk of metabolic disease. Some of this excessive energy may be stored through a pathologic transfer of free fatty acids to non-adipose tissue organs (e.g., the liver and muscle), which contributes to metabolic disease Citation[6]. However, most excessive energy is stored in various adipose tissue depots. This results in the sometimes curious characterization of additional adipose tissue (such as increased visceral adipose tissue) as representing ‘ectopic fat’ Citation[166]. In fact, any excessive fat in any location is considered by some as representing ‘ectopic fat’. However, if all excessive fat storage is ‘ectopic’, then this raises the question as to the clinical utility of this term. One could argue that ‘adiposity’ is a term sufficient in describing excessive body fat, and the term ‘adiposopathy’ is more precise in describing when excessive body fat is pathogenic in causing or promoting adverse metabolic consequences leading to metabolic disease.
As the pathogenic potential of adipose tissue becomes more widely acknowledged, specific diagnostic criteria will need to be developed for adiposopathy or ‘sick fat’ Citation[1]. Once this process is complete, then perhaps regulatory agencies would be more open to establishing treatment indications to improve adiposopathy (a true disease) Citation[167], and thus free themselves from approval processes whose only definitive metrics are focused on reductions in body weight Citation[168,209]. In other words, the current FDA guidance for industry has comments such as how: “excessive body fat increases the risk of death and major comorbidities such as Type 2 diabetes, hypertension, dyslipidemia, cardiovascular disease, osteoarthritis of the knee, sleep apnea and some cancers” Citation[209], suggesting a belief that adiposity is merely associated with, rather than potentially causative of, fat-mass complications and metabolic disease. Furthermore, although current guidance does stress the importance of improvement in metabolic disease, the degree by which weight loss agents safely improve the weight of patients remains the focus, as opposed to the degree by which these agents improve the metabolic health of patients.
This brings us to PHEN/TPM CR. Phentermine is an anti-obesity drug indicated for short-term treatment of obesity. It is an approved pharmaceutical that has been in clinical use for approximately 50 years, and is the most widely prescribed anti-obesity agent. Phentermine has no reported adverse drug interactions when used with topiramate, and has no product information statements warning against its use with topiramate. Topiramate is an anti-seizure drug and migraine headache prophylactic drug. It is an approved pharmaceutical that has been in development since the 1970s and in clinical use for over a decade. It is among the most commonly prescribed anticonvulsant therapies. Topiramate has no reported adverse drug interactions when used with phentermine, and has no product information statements warning against its use with phentermine. Data in this review support PHEN/TPM CR as being no less safe or no less tolerated than what would be expected from the individual components. This might be anticipated given that the PHEN/TPM CR combination agent includes topiramate in a controlled-release formulation (although this has yet to be proven to afford benefits over non-controlled-released formulations), and utilizes doses of phentermine and topiramate that are a fraction of their respective maximum approved doses. The safety dataset will be substantially enhanced by the results of the long-term OB-305 extension study (SEQUEL), due sometime in latter 2010 or early 2011. If clinicians accept PHEN/TPM CR as being as safe as the individual components, then fundamental decisions will have to be made regarding the clinical importance of adiposity and adiposopathy.
Clinicians may question whether the weight loss with PHEN/TPM CR is sufficient to improve fat-mass diseases. The OB-204 study reported a significant improvement in sleep apnea with PHEN/TPM CR, which would seem to be a significant benefit in reducing fat mass Citation[210]. Clinicians may also question whether the statistically significant weight loss with PHEN/TPM CR represents clinically meaningful weight loss. Insight as to what may be ‘clinically meaningful’ might be derived from regulatory agency guidance. The FDA ‘Guidance for Industry: Developing Products for Weight Management’ Citation[209] has suggested regulatory metrics for approval of weight loss drugs and therapeutic biologics (termed ‘products’). According to the FDA, Phase I and II trials should characterize the pharmacokinetics and dose response of the product, over a range of doses and across a broad range of BMI (e.g., BMI ≥30 kg/m2; or ≥27 kg/m2 if with comorbidities) to determine no-effect, maximally tolerated doses and efficacy of all doses relative to placebo. The duration should be long enough to capture the product’s maximal or near-maximal weight loss effects. Primary end points should be mean absolute or percent change in body weight in the active versus placebo group, as well as the proportion of each treatment group with a weight loss of 5% or more of baseline weight. Other end points should include evaluations of weight-related comorbidities. The EQUATE study supports PHEN/TPM CR as fulfilling many of these criteria.
Phase III trials should be randomized, double-blind, placebo-controlled 1-year studies in patients with a BMI of 30 kg/m2 or more, or 27 kg/m2 or more if with comorbidities such as Type 2 diabetes mellitus, hypertension, dyslipidemia, sleep apnea and cardiovascular disease (with some having a BMI >40 kg/m2), and with representation from demographic, ethnic and racial groups having a high prevalence of obesity. The concomitant lifestyle modification program: “should strike an appropriate balance between effectiveness and simplicity.” A reasonable safety set would include 3000 subjects randomized to active drug and no fewer than 1500 randomized to comparator placebo. Recommended primary end points would be mean difference in percent loss of baseline body weight (active treatment vs placebo) and categorical (proportional) analysis of study participants who lost at least 5% of baseline body weight (active treatment vs placebo). Secondary end points should include changes in fasting glucose, insulin and HbA1c levels, BP, pulse and lipid levels. Waist circumference should also be measured; however, while acceptable as a surrogate in clinical practice, waist circumference is not considered an acceptable surrogate for visceral fat in the context of a clinical trial. More direct measures for visceral adiposity would include dual energy x-ray absorptiometry composition analysis, which is also important to ensure that the weight loss is primarily fat and not loss of lean body mass. Concomitant metabolic drug therapies should be assessed by protocol-defined algorithms to determine their initiation, withdrawal, increase in dose and decrease in dose during the course of the trials. ‘Effectiveness’ of a weight loss product is achieved if after 1 year of treatment, one of the two following benchmarks are met:
• The difference in mean weight loss between the active product and placebo-treated groups is at least 5%, and the difference is statistically significant;
• The proportion of subjects who lose 5% of baseline body weight or more in the active product group is at least 35%, is approximately double the proportion in the placebo-treated group, and the difference between groups is statistically significant.
Although overweight and obese study participants are expected to receive standard of care for metabolic comorbidities, and although no specific criteria are given, glycemic, BP and lipid measurements are expected to improve as would be anticipated with the degree of weight loss.
Other guidance recommendations are specific for individual weight loss agents. Weight management products that act upon the CNS should include an assessment of neuropsychiatric function through validated scales, as well as potentially additional studies to evaluate abuse liability. Weight management products that are therapeutic proteins should undergo assessment of immunogenic potential for 6–12 months. Combination weight management products should first have data comparing the combination product to its individual components of sufficient duration to capture their respective maximal or near-maximal weight effects. The combination weight management product should have greater weight loss efficacy compared with its individual components, with weight loss twice the individual components being preferred.
Special guidance is made regarding the evaluation of overweight and obese patients with Type 2 diabetes mellitus. Weight loss with bariatric surgery can substantially improve many metabolic parameters. Its favorable effects upon Type 2 diabetes mellitus are particularly noteworthy, with remission of Type 2 diabetes mellitus being as high as 80% Citation[50]. Conversely, several prior anti-obesity drug trials have often resulted in a less than robust response in weight loss and in improving diabetes mellitus, possibly because dramatic weight loss may be required to significantly improve fat function (particularly in older patients). This suggests that the degree of success of weight-loss interventions in improving diabetes mellitus may be dependent upon the age of the patient, the pathology of the diabetes mellitus, the duration of the diabetes mellitus, and the degree of potential improvement in the functionality of adipocytes and adipose tissue Citation[17], as well as other body organs. In their guidance, the FDA reflected this viewpoint by stating: “compared with nondiabetic patients, overweight and obese patients with Type 2 diabetes often respond less favorably to weight-management products.”
In consideration of all the above, supports PHEN/TPM CR as meeting many of the FDA weight loss guidance criteria. also supports PHEN/TPM CR as providing clinically meaningful improvements in metabolic diseases such as diabetes mellitus, hypertension and dyslipidemia. PHEN/TPM CR does not appear to significantly adversely increase depression, as assessed by common neuropsychiatric scales Citation[205]. Finally, as noted before, in a trial of overweight or obese patients with Type 2 diabetes mellitus, PHEN/TPM CR produced a robust 9.4% reduction in body weight (vs a reduction of 2.7% with placebo), and a substantial reduction in HbA1c of 1.6% (vs reduction of 1.1% with placebo) Citation[163,208]. The high degree of HbA1c reduction with placebo was at least partially due to an increase in anti-diabetes mellitus therapies. The even greater HbA1c reduction with PHEN/TPM CR was despite a net decrease in anti-diabetes mellitus therapies. It is unclear that these data suggest PHEN/TPM CR as having unique weight loss and/or antiglycemic properties that make it superior to other anti-obesity drug therapies in patients with Type 2 diabetes mellitus. To prove this, head-to-head controlled clinical trials would need to be performed. However, it is interesting to revisit the fact that topiramate was originally developed as an agent to treat Type 2 diabetes mellitus Citation[60,107–109].
Having met efficacy criteria, the main issue regarding the regulatory approval of PHEN/TPM CR would seem to be (not surprisingly) matters of safety Citation[211]. On 15 July 2010, a FDA advisory committee issued a mixed decision of 10 to 6 against endorsing PHEN/TPM CR as an approved pharmaceutical at that time. Reports suggested that: “the FDA and most panel members did not question the effectiveness of” PHEN/TPM CR; “several panel members said they could just as easily have voted the other way.” However, the main potential safety concerns noted were an increase in heart rate (“…in the 1-year cohort, small mean increases in heart rate were observed in the QNEXA treatment groups [0.6–1.6 beats per minute at the top dose] compared with placebo treatment groups…”) Citation[211], possible birth defects, impaired memory and concentration, metabolic acidosis with kidney stones and psychiatric problems (e.g., depression and suicidal ideations) Citation[212].
All of these potential adverse experiences were discussed previously in this review, except for the potential for birth defects. The pregnancy risks of pharmaceuticals are often characterized by the FDA according to categories. The safest are pregnancy categories A (e.g., thyroid medication at replacement doses) and B (e.g., amoxicillin). The least safe are pregnancy categories D (e.g., tetracycline) and X (e.g., statins). Phentermine and topiramate are both pregnancy category C Citation[211], which means that animal reproduction studies have shown an adverse effect on the fetus, but no adequate and well-controlled studies exist in humans, and the potential benefits may warrant use of the drug in pregnant women despite potential risks.
Regarding issues that may have influenced the split decision, some reports suggest that the FDA’s advisory committee’s decision was influenced by the controversy (just days before) involving matters of another FDA advisory committee’s deliberation regarding an otherwise unrelated anti-diabetes mellitus drug Citation[212]. With regard to the FDA’s initial reaction to the decision:
“Eric Colman, deputy director of the FDA’s Division of Metabolism and Endocrinology Products, noted that the agency’s current draft guidelines only call for one year of safety data. He told reporters after the meeting that based on the outcome of Thursday’s committee and the upcoming panels for lorcaserin and Contrave, the FDA likely will update that guidance before releasing a final version of the document. Colman said he was ‘surprised’ by the outcome of Thursday’s vote. ‘I’ve learned that you never know what is going to happen, what they are going to say, how they are going to vote,’ he told reporters. But Colman said he thought there was ‘a little bit of hesitancy’ from those who voted against approval, adding that those panelists ‘were not strongly against the drug, but had lingering concerns that were enough to vote no.’ While the FDA is requiring makers of Type II diabetes drugs to conduct cardiovascular outcomes trials, Colman noted that the agency has yet to mandate the same for weight loss drugs. ‘We’ve had those conversations,’ he said. ‘But the discussion hasn’t evolved to the point to where we are making it formal,’ Colman said, noting the panel’s concerns about increased heart rates in Vivus’ studies of Qnexa” Citation[213].
The reference to the regulatory requirements of cardiovascular outcomes pertains to the FDA guidance on the development of diabetes mellitus drug therapies, as issued in December 2008 (Box 1)Citation[214]. It is therefore possible that similar regulatory recommendations may soon apply to anti-obesity drug development, with the potential requirement of a planned or partially enrolled cardiovascular disease outcome study upon approval. Alternatively or additionally, longer term clinical trials might be required (longer than 1 year) to assess the safety risks associated with chronic anti-obesity therapy.
In addition to legitimate matters of safety, unique obstacles may exist to anti-obesity drug development. These impediments may include bias against pharmaceutical therapies for obesity, especially among those who believe obesity is mainly a behavioral problem that should only be managed through appropriate nutrition and lifestyle changes. But while true that adiposity often has a strong behavioral component, medical science has not historically withheld drug development or medical care on the basis that a disease or condition is substantially due to a lack of medically favorable personal behavior Citation[7]. As importantly, the resistance to anti-obesity drug development may also reflect medical and social biases against the obese, which have existed for centuries Citation[169], and manifest by a sense that treating adiposity is not as important as treating other diseases. In other words, perfectly valid clinical reasons exist why medical science currently has only one or two drugs available to treat the world’s greatest epidemic Citation[87]. But it is also reasonable to question the apparent belief among many that unless a weight-loss pharmaceutical has virtually no risk, then the benefits of weight loss are not sufficient to warrant approval. It is disturbing that adiposity and adiposopathy are on the brink of representing the first epidemics in history that medical science has implicitly decided are untreatable by pharmaceutical therapy.
As such, a most fundamental question facing researchers, regulatory agencies and clinicians is that relative to other diseases, how important is treating adiposity, adiposopathy and affiliated metabolic diseases (e.g., Type 2 diabetes mellitus, hypertension and dyslipidemia)? Through their prescribing habits, clinicians have already decided that the risk:benefit ratio of the 100 mg dose of topiramate (not controlled-release) is acceptable for prophylaxis of migraine headaches Citation[170]. Do clinicians likewise agree that PHEN/TPM CR (which incorporates, at most, 92 mg of topiramate in a controlled-release formulation) is acceptable for treatment of adiposity, adiposopathy and metabolic disease? Is preventing migraine headaches of greater or less clinical importance than improving the numerous metabolic abnormalities associated with adiposity and adiposopathy? . If clinicians ultimately agree that the pathologies of adiposity and adiposopathy are equally as worthy of treatment as preventing migraine headaches, then based upon available evidence, PHEN/TPM CR appears to not only improve the weight of patients, but also appears to improve the metabolic health of patients. This meets the evolving, suggested criteria for weight loss drug development, which states: “An emerging concept is that the development of anti-obesity agents must not only reduce fat mass (adiposity) but must also correct fat dysfunction (adiposopathy)” Citation[60].
Update
Following the online publication of this manuscript on 16 August 2010, the following updates have occurred:
• In October 2010, sibutramine (Meridia) was withdrawn from the US market due to its potential to increase the risk for cardiovascular events. This left only one drug, orlistat, approved for long-term treatment of obesity (see the section of this manuscript entitled ‘Expert commentary & five-year view, for more information).
• After the decision of the US FDA advisory panel (described in this manuscript), the FDA issued a Complete Response Letter to the manufacturer of phentermine HCl/topiramate controlled-release (PHEN/TPM CR), wherein additional data was requested Citation[218], including:
– Clinical: the FDA requested a comprehensive assessment of PHEN/TPM CR’s teratogenic potential, cardiovascular implications of the associated increase in heart rate, and data from the already completed SEQUEL study (OB-305) (see the section of this manuscript entitled ‘Expert commentary & five-year view’ for more information);
– Labeling: the FDA reserved the right to comment further on proposed labeling;
– Risk Evaluation and Mitigation Strategy (REMS): the FDA requested a further discussion of a previously submitted REMS plan to better determine the long-term health outcomes of PHEN/TPM CR;
– Safety update: the FDA requested a safety update of any new adverse experiences;
– Drug scheduling: the FDA stated that if approved, PHEN/TPM CR would be a Schedule IV drug due to the phentermine component (see the section of this manuscript entitled ‘Phentermine’ for more information).
Table 1. Examples of treatments for adiposopathy (‘sick fat’) and their effects upon illustrative and selected adipose tissue factors that may contribute to metabolic disease.
Table 2. Preliminary data of Phase III clinical trials of the once-a-day phentermine/topiramate controlled-release combination agent.
Table 3. Percent of adverse experiences occurring in over 5% of study participants (regardless of causality) with PHEN/TPM CR during the EQUIP (n = 1264) and CONQUER (n = 2485) trials.
Table 4. Discontinuations due to adverse experiences in the EQUIP and CONQUER studies.
Box 1. US FDA: 2008 guidance for evaluating cardiovascular risk associated with new anti-diabetes mellitus drug therapies.
“To establish the safety of a new antidiabetic therapy to treat Type 2 diabetes, sponsors should demonstrate that the therapy will not result in an unacceptable increase in cardiovascular risk. To ensure that a new therapy does not increase cardiovascular risk to an unacceptable extent, the development program for a new Type 2 antidiabetic therapy should include the following.
For new clinical studies in the planning stage:
• Sponsors should establish an independent cardiovascular end points committee to prospectively adjudicate, in a blinded fashion, cardiovascular events during all Phase II and Phase III trials. These events should include cardiovascular mortality, myocardial infarction, and stroke, and can include hospitalization for acute coronary syndrome, urgent revascularization procedures and possibly other end points.
• Sponsors should ensure that Phase II and Phase III clinical trials are appropriately designed and conducted so that a meta-analysis can be performed at the time of completion of these studies that appropriately accounts for important study design features and patient or study level covariates. To obtain sufficient end points to allow a meaningful estimate of risk, the Phase II and Phase III programs should include patients at higher risk of cardiovascular events, such as patients with relatively advanced disease, elderly patients and patients with some degree of renal impairment. Because these types of patients are likely to be treated with the antidiabetic agent, if approved, this population is more appropriate than a younger and healthier population for assessment of other aspects of the test drug’s safety.
• Sponsors also should provide a protocol describing the statistical methods for the proposed meta-analysis, including the end points that will be assessed. At this time, we believe it would be reasonable to include in a meta-analysis all placebo-controlled trials, add-on trials (i.e., drug versus placebo, each added to standard therapy), and active-controlled trials, and to preserve the study level randomized comparison but include, when possible in the meta-analysis, important identifiers of study differences or other factors (e.g., dose, duration of exposure, add-on drugs). It is likely that the controlled trials will need to last more than the typical 3–6 months duration to obtain enough events and to provide data on longer-term cardiovascular risk (e.g., minimum 2 years) for these chronically used therapies.
• Sponsors should perform a meta-analysis of the important cardiovascular events across Phase II and Phase III controlled clinical trials and explore similarities and/or differences in subgroups (e.g., age, sex, race), if possible.
For completed studies, before submission of the new drug application (NDA)/biologics license application (BLA):
• Sponsors should compare the incidence of important cardiovascular events occurring with the investigational agent to the incidence of the same types of events occurring with the control group to show that the upper bound of the two-sided 95 percent confidence interval for the estimated risk ratio is less than 1.8. This can be accomplished in several ways. The integrated analysis (meta-analysis) of the Phase II and Phase III clinical trials described above can be used. Or, if the data from all the studies that are part of the meta-analysis will not by itself be able to show that the upper bound of the two-sided 95 percent confidence interval for the estimated risk ratio is less than 1.8, then an additional single, large safety trial should be conducted that alone, or added to other trials, would be able to satisfy this upper bound before NDA/BLA submission. Regardless of the method used, sponsors should consider the entire range of possible increased risk consistent with the confidence interval and the point estimate of the risk increase. For example, it would not be reassuring to find a point estimate of 1.5 (a nominally significant increase), even if the 95 percent upper bound was less than 1.8.
• If the premarketing application contains clinical data that show that the upper bound of the two-sided 95 percent confidence interval for the estimated increased risk (i.e., risk ratio) is between 1.3 and 1.8, and the overall risk-benefit analysis supports approval, a postmarketing trial generally will be necessary to definitively show that the upper bound of the two-sided 95 percent confidence interval for the estimated risk ratio is less than 1.3. This can be achieved by conducting a single trial that is adequately powered or by combining the results from a premarketing safety trial with a similarly designed postmarketing safety trial. This clinical trial will be a required postmarketing safety trial.
• If the premarketing application contains clinical data that show that the upper bound of the two-sided 95 percent confidence interval for the estimated increased risk (i.e., risk ratio) is less than 1.3 and the overall risk-benefit analysis supports approval, a postmarketing cardiovascular trial generally may not be necessary.
• The report of this meta-analysis should contain sufficient detail for all the analyses; conventional graphical plots for meta-analysis finding by study, subgroup, and overall risk ratio; and all the analysis data sets that would allow a verification of the findings.”
Key issues
• Adipose tissue is an active endocrine and immune organ.
• An increase in fat storage in adipocytes can result in pathologic anatomical, mechanistic, endocrinologic and immunologic adipose tissue responses that may contribute to metabolic disease.
• Adiposopathy (‘sick fat’) is defined as pathogenic adipose tissue anatomical changes that often occur with positive caloric balance, particularly among those who are environmentally or genetically predisposed, which results in pathological endocrine and immune responses that are important contributors to metabolic disease.
• Phentermine is a noradrenergic sympathomimetic amine approved for short-term treatment of obesity.
• Topiramate is a sulfamate-substituted monosaccharide derivative of the naturally occurring sugar monosaccharide D-fructose approved as treatment for migraine headaches and seizure disorders, which also has weight loss effects.
• Phentermine HCl/topiramate controlled-release is a combination agent containing immediate-release phentermine and controlled-release topiramate that may not only improve the weight of patients, but may also improve adiposopathy-associated metabolic diseases.
Financial & competing interests disclosure
Harold Bays has served as a Clinical Investigator for (and has received research grants from) pharmaceutical companies such as Abbott, Aegerion, Akros, Amarin, Amgen, Amylin, Alteon, Arena, Arete, AstraZeneca, Aventis, Bayer, Boehringer, Bristol-Myers Squibb, Cargill, Ciba Geigy, Daiichi Sankyo, Eli Lilly, Esperion, Essentialis, Fujisawa, GelTex, Genentech, GlaxoSmithKline, Hoechst Roussel, Hoffman LaRoche, Home Access, InterMune, Intekrin, Ironwood Pharmaceuticals, ISIS, Johnson & Johnson, KOS, Kowa, Kyorin, Lederle, Marion Merrell Dow, Merck, Merck Schering Plough, Metabolex, Microbia, Miles, Neuromed, Nicox, Novartis, NovoNordisk, Obecure, Orexigen, Parke Davis, Pfizer, Pliva, Posen, Purdue, Reliant, Roche, Rorer, Regeneron, Sandoz, Sanofi, Sciele, Searle, Shionogi, Schering Plough, SmithKline Beecham, Surface Logix, Takeda, TAP, UpJohn, Upsher Smith, Warner Lambert, Vivus and Wyeth-Ayerst. Harold Bays has served as a consultant, speaker and/or advisor to and for pharmaceutical companies such as Abbott, Amarin, Arena, AstraZeneca, Aventis, Bayer, Bristol-Myers Squibb, Cerenis, Daiichi Sankyo, DSM Nutritional Products, Inc., Essentialis Therapeutics, GlaxoSmithKline, Ironwood Pharmaceuticals, Johnson & Johnson, KOS, Merck, Merck Schering Plough, Metabasis Therapeutics, Microbia, Novartis, Nicox, Ortho-McNeil, Parke Davis, Perenis Therapeutics, Pfizer, Reliant, Roche, Sandoz, Sanofi Aventis, Schering Plough, SmithKline Beecham, Surface Logix, Takeda, UpJohn, Vivus and Warner Lambert. The author has no other relevant affiliations or financial involvement with any organization or entity with a financial interest in or financial conflict with the subject matter or materials discussed in the manuscript apart from those disclosed.
No funding or writing assistance was utilized in the production of this manuscript.
Notes
Data taken from Citation[214].
References
- Bays H, Abate N, Chandalia M. Adiposopathy: sick fat causes high blood sugar, high blood pressure, and dyslipidemia. Future Cardiol.1(1), 39–59 (2005).
- Bays HE. ‘Sick fat,’ metabolic disease, and atherosclerosis. Am. J. Med.122(1 Suppl.), S26–S37 (2009).
- Bays HE, Rodbard RW, Schorr AB, González-Campoy JM. Adiposopathy: treating pathogenic adipose tissue to reduce cardiovascular disease risk. Curr. Treat. Options Cardiovasc. Med.9(4), 259–271 (2007).
- Lavie CJ, Milani RV, Ventura HO. Obesity and cardiovascular disease: risk factor, paradox, and impact of weight loss. J. Am. Coll. Cardiol.53(21), 1925–1932 (2009).
- Bays H, Ballantyne C. Adiposopathy: why do adiposity and obesity cause metabolic disease? Future Lipidol.1(4), 389–420 (2006).
- Bays HE, Gonzalez-Campoy JM, Bray GA et al. Pathogenic potential of adipose tissue and metabolic consequences of adipocyte hypertrophy and increased visceral adiposity. Expert Rev. Cardiovasc. Ther.6(3), 343–368 (2008).
- Bays HE, Gonzalez-Campoy JM, Henry RR et al. Is adiposopathy (sick fat) an endocrine disease? Int. J. Clin. Pract.62(10), 1474–1483 (2008).
- Roche AF. The adipocyte-number hypothesis. Child Dev.52(1), 31–43 (1981).
- Zhu X, He Q, Lin Z. Human preadipocyte culture and the establishment of hyperplasia and hypertrophy model. Zhonghua Zheng Xing Shao Shang Wai Ke Za Zhi15(3), 199–201 (1999).
- Dubois SG, Heilbronn LK, Smith SR, Albu JB, Kelley DE, Ravussin E. Decreased expression of adipogenic genes in obese subjects with Type 2 diabetes. Obesity (Silver Spring)14(9), 1543–1552 (2006).
- Gregoire FM. Adipocyte differentiation: from fibroblast to endocrine cell. Exp. Biol. Med. (Maywood)226(11), 997–1002 (2001).
- Haller H, Leonhardt W, Hanefeld M, Julius U. Relationship between adipocyte hypertrophy and metabolic disturbances. Endokrinologie74(1), 63–72 (1979).
- Smith U. Effect of cell size on lipid synthesis by human adipose tissue in vitro. J. Lipid Res.12(1), 65–70 (1971).
- Bray GA, Glennon JA, Salans LB, Horton ES, Danforth E Jr, Sims EA. Spontaneous and experimental human obesity: effects of diet and adipose cell size on lipolysis and lipogenesis. Metabolism26(7), 739–747 (1977).
- Salans LB, Bray GA, Cushman SW et al. Glucose metabolism and the response to insulin by human adipose tissue in spontaneous and experimental obesity. Effects of dietary composition and adipose cell size. J. Clin. Invest.53(3), 848–856 (1974).
- Hissin PJ, Foley JE, Wardzala LJ et al. Mechanism of insulin-resistant glucose transport activity in the enlarged adipose cell of the aged, obese rat. J. Clin. Invest.70(4), 780–790 (1982).
- Bays H, Dujovne CA. Adiposopathy is a more rational treatment target for metabolic disease than obesity alone. Curr. Atheroscler. Rep.8(2), 144–156 (2006).
- Karelis AD, St-Pierre DH, Conus F, Rabasa-Lhoret R, Poehlman ET. Metabolic and body composition factors in subgroups of obesity: what do we know? J. Clin. Endocrinol. Metab.89(6), 2569–2575 (2004).
- Bays HE. Anthropometric measurements and diabetes mellitus: clues to the ‘pathogenic’ and ‘protective’ potential of adipose tissue. Metab. Syndr. Relat. Disord.8(4), 307–315 (2010).
- Tchkonia T, Lenburg M, Thomou T et al. Identification of depot-specific human fat cell progenitors through distinct expression profiles and developmental gene patterns. Am. J. Physiol. Endocrinol. Metab.292(1), E298–E307 (2007).
- Bergman RN, Kim SP, Catalano KJ et al. Why visceral fat is bad: mechanisms of the metabolic syndrome. Obesity (Silver Spring)14(Suppl. 1), 16S–19S (2006).
- Weyer C, Foley JE, Bogardus C, Tataranni PA, Pratley RE. Enlarged subcutaneous abdominal adipocyte size, but not obesity itself, predicts Type II diabetes independent of insulin resistance. Diabetologia43(12), 1498–1506 (2000).
- Bays HE. Lorcaserin and adiposopathy: 5-HT2c agonism as a treatment for ‘sick fat’ and metabolic disease. Expert Rev. Cardiovasc. Ther.7(11), 1429–1445 (2009).
- Sims EA. Are there persons who are obese, but metabolically healthy? Metabolism50(12), 1499–1504 (2001).
- Bays H, Blonde L, Rosenson R. Adiposopathy: how do diet, exercise, weight loss and drug therapies improve metabolic disease? Expert Rev. Cardiovasc. Ther.4(6), 871–895 (2006).
- Lebovitz HE. The relationship of obesity to the metabolic syndrome. Int. J. Clin. Pract. Suppl.134, 18–27 (2003).
- Dusserre E, Moulin P, Vidal H. Differences in mRNA expression of the proteins secreted by the adipocytes in human subcutaneous and visceral adipose tissues. Biochim. Biophys. Acta1500(1), 88–96 (2000).
- Vague J. La differenciation sexuelle, facteur determinant des formes de l’obesite. Presse Med.30, 339–340 (1947).
- Schaffler A, Müller-Ladner U, Schölmerich J, Buchler C. Role of adipose tissue as an inflammatory organ in human diseases. Endocr. Rev.27(5), 449–467 (2006).
- Imbeault P, Lemieux S, Prud’homme D et al. Relationship of visceral adipose tissue to metabolic risk factors for coronary heart disease: is there a contribution of subcutaneous fat cell hypertrophy? Metabolism48(3), 355–362 (1999).
- Klein S. The case of visceral fat: argument for the defense. J. Clin. Invest.113(11), 1530–1532 (2004).
- Johnson JA, Fried SK, Pi-Sunyer FX, Albu JB. Impaired insulin action in subcutaneous adipocytes from women with visceral obesity. Am. J. Physiol. Endocrinol. Metab.280(1), E40–E49 (2001).
- Jensen MD. Is visceral fat involved in the pathogenesis of the metabolic syndrome? Human model. Obesity (Silver Spring)14(Suppl. 1), 20S–24S (2006).
- Jensen MD, Johnson CM. Contribution of leg and splanchnic free fatty acid (FFA) kinetics to postabsorptive FFA flux in men and women. Metabolism45(5), 662–666 (1996).
- Abate N, Garg A, Peshock RM, Stray-Gundersen J, Adams-Huet B, Grundy SM. Relationship of generalized and regional adiposity to insulin sensitivity in men with NIDDM. Diabetes45(12), 1684–1693 (1996).
- Goodpaster BH, Thaete FL, Simoneau JA, Kelley DE. Subcutaneous abdominal fat and thigh muscle composition predict insulin sensitivity independently of visceral fat. Diabetes46(10), 1579–1585 (1997).
- Bluher M. Adipose tissue dysfunction in obesity. Exp. Clin. Endocrinol. Diabetes117(6), 241–250 (2009).
- Ye J. Emerging role of adipose tissue hypoxia in obesity and insulin resistance. Int. J. Obes. (Lond.)33(1), 54–66 (2009).
- Kershaw EE, Flier JS. Adipose tissue as an endocrine organ. J. Clin. Endocrinol. Metab.89(6), 2548–2556 (2004).
- Jazet IM, Pijl H, Meinders AE. Adipose tissue as an endocrine organ: impact on insulin resistance. Neth. J. Med.61(6), 194–212 (2003).
- Miner JL. The adipocyte as an endocrine cell. J. Anim. Sci.82(3), 935–941 (2004).
- Ahima RS. Adipose tissue as an endocrine organ. Obesity (Silver Spring)14(Suppl. 5), 242S–249S (2006).
- Caspar-Bauguil S, Cousin B, Galinier A et al. Adipose tissues as an ancestral immune organ: site-specific change in obesity. FEBS Lett.579(17), 3487–3492 (2005).
- Fantuzzi G. Adipose tissue, adipokines, and inflammation. J. Allergy Clin. Immunol.115(5), 911–919 (2005).
- Trayhurn P, Wood IS. Signalling role of adipose tissue: adipokines and inflammation in obesity. Biochem. Soc. Trans.33(Pt 5), 1078–1081 (2005).
- Wellen KE, Hotamisligil GS. Obesity-induced inflammatory changes in adipose tissue. J. Clin. Invest.112(12), 1785–1788 (2003).
- Wisse BE. The inflammatory syndrome: the role of adipose tissue cytokines in metabolic disorders linked to obesity. J. Am. Soc. Nephrol.15(11), 2792–2800 (2004).
- Kougias P, Chai H, Lin PH, Yao Q, Lumsden AB, Chen C. Effects of adipocyte-derived cytokines on endothelial functions: implication of vascular disease. J. Surg. Res.126(1), 121–129 (2005).
- Ortega Martinez de V, Xu X, Koska J et al. Macrophage content in subcutaneous adipose tissue: associations with adiposity, age, inflammatory markers, and whole-body insulin action in healthy Pima Indians. Diabetes58(2), 385–393 (2009).
- Bays HE, Laferrere B, Dixon J et al. Adiposopathy and bariatric surgery: is ‘sick fat’ a surgical disease? Int. J. Clin. Pract.63(9), 1285–1300 (2009).
- Frayn KN, Summers LK, Fielding BA. Regulation of the plasma non-esterified fatty acid concentration in the postprandial state. Proc. Nutr. Soc.56(2), 713–721 (1997).
- Bays H, Mandarino L, DeFronzo RA. Role of the adipocyte, free fatty acids, and ectopic fat in pathogenesis of Type 2 diabetes mellitus: peroxisomal proliferator-activated receptor agonists provide a rational therapeutic approach. J. Clin. Endocrinol. Metab.89(2), 463–478 (2004).
- Storlien L, Oakes ND, Kelley DE. Metabolic flexibility. Proc. Nutr. Soc.63(2), 363–368 (2004).
- Bays HE, Rodbard RW, Schorr AB, González-Campoy JM. Treating pathogenic adipose tissue (adiposopathy) to reduce cardiovascular disease risk. Curr. Treat. Options Cardiovasc. Med.9(4), 259–271 (2007).
- Adams JM, Pratipanawatr T, Berria R et al. Ceramide content is increased in skeletal muscle from obese insulin-resistant humans. Diabetes53(1), 25–31 (2004).
- Chavez JA, Holland WL, Bar J, Sandhoff K, Summers SA. Acid ceramidase overexpression prevents the inhibitory effects of saturated fatty acids on insulin signaling. J. Biol. Chem.280(20), 20148–20153 (2005).
- Kelley DE, Goodpaster BH. Skeletal muscle triglyceride. An aspect of regional adiposity and insulin resistance. Diabetes Care24(5), 933–941 (2001).
- Grill V, Persson G, Carlsson S et al. Family history of diabetes in middle-aged Swedish men is a gender unrelated factor which associates with insulinopenia in newly diagnosed diabetic subjects. Diabetologia42(1), 15–23 (1999).
- Lillioja S, Mott DM, Spraul M et al. Insulin resistance and insulin secretory dysfunction as precursors of non-insulin-dependent diabetes mellitus. Prospective studies of Pima Indians. N. Engl. J. Med.329(27), 1988–1992 (1993).
- Bays HE. Current and investigational antiobesity agents and obesity therapeutic treatment targets. Obes. Res.12(8), 1197–1211 (2004).
- Villarroya F, Domingo P, Giralt M. Drug-induced lipotoxicity: lipodystrophy associated with HIV-1 infection and antiretroviral treatment. Biochim. Biophys. Acta1801(3), 392–399 (2010).
- Tsai AG, Wadden TA. Systematic review: an evaluation of major commercial weight loss programs in the United States. Ann. Intern. Med.142(1), 56–66 (2005).
- Varady KA, Tussing L, Bhutani S, Braunschweig CL. Degree of weight loss required to improve adipokine concentrations and decrease fat cell size in severely obese women. Metabolism58(8), 1096–1101 (2009).
- Ratner RE. An update on the Diabetes Prevention Program. Endocr. Pract.12(Suppl. 1), 20–24 (2006).
- Nair RP, Ren J. Pharmacotherapy of obesity – benefit, bias and hyperbole. Curr. Med. Chem.16(15), 1888–1897 (2009).
- Aubin D, Gagnon A, Grunder L, Dent R, Allen M, Sorisky A. Adipogenic and antiapoptotic protein levels in human adipose stromal cells after weight loss. Obes. Res.12(8), 1231–1234 (2004).
- Zhu M, Lee GD, Ding L et al. Adipogenic signaling in rat white adipose tissue: modulation by aging and calorie restriction. Exp. Gerontol.42(8), 733–744 (2007).
- Levine JA. Measurement of energy expenditure. Public Health Nutr.8(7A), 1123–1132 (2005).
- Flatt JP. Differences in basal energy expenditure and obesity. Obesity (Silver Spring)15(11), 2546–2548 (2007).
- Livingston EH, Kohlstadt I. Simplified resting metabolic rate-predicting formulas for normal-sized and obese individuals. Obes. Res.13(7), 1255–1262 (2005).
- Weinsier RL, Nagy TR, Hunter GR, Darnell BE, Hensrud DD, Weiss HL. Do adaptive changes in metabolic rate favor weight regain in weight-reduced individuals? An examination of the set-point theory. Am. J. Clin. Nutr.72(5), 1088–1094 (2000).
- Rosenbaum M, Hirsch J, Gallagher DA, Leibel RL. Long-term persistence of adaptive thermogenesis in subjects who have maintained a reduced body weight. Am. J. Clin. Nutr.88(4), 906–912 (2008).
- Goldsmith R, Joanisse DR, Gallagher D et al. Effects of experimental weight perturbation on skeletal muscle work efficiency, fuel utilization, and biochemistry in human subjects. Am. J. Physiol. Regul. Integr. Comp. Physiol.298(1), R79–R88 (2010).
- Stiegler P, Cunliffe A. The role of diet and exercise for the maintenance of fat-free mass and resting metabolic rate during weight loss. Sports Med.36(3), 239–262 (2006).
- Gibson WT, Farooqi IS, Moreau M et al. Congenital leptin deficiency due to homozygosity for the d133G mutation: report of another case and evaluation of response to four years of leptin therapy. J. Clin. Endocrinol. Metab.89(10), 4821–4826 (2004).
- Farooqi IS, O’Rahilly S. Leptin: a pivotal regulator of human energy homeostasis. Am. J. Clin. Nutr.89(3), 980S–984S (2009).
- Rosenbaum M, Goldsmith R, Bloomfield D et al. Low-dose leptin reverses skeletal muscle, autonomic, and neuroendocrine adaptations to maintenance of reduced weight. J. Clin. Invest.115(12), 3579–3586 (2005).
- Flores MB, Fernandes MF, Ropelle ER et al. Exercise improves insulin and leptin sensitivity in hypothalamus of Wistar rats. Diabetes55(9), 2554–2561 (2006).
- Bjorbaek C. Central leptin receptor action and resistance in obesity. J. Investig. Med.57(7), 789–794 (2009).
- King NA, Hopkins M, Caudwell P, Stubbs RJ, Blundell JE. Beneficial effects of exercise: shifting the focus from body weight to other markers of health. Br. J. Sports Med.43(12), 924–927 (2009).
- Chaston TB, Dixon JB, O’Brien PE. Changes in fat-free mass during significant weight loss: a systematic review. Int. J. Obes. (Lond.)31(5), 743–750 (2007).
- Metcalf B, Rabkin RA, Rabkin JM, Metcalf LJ, Lehman-Becker LB. Weight loss composition: the effects of exercise following obesity surgery as measured by bioelectrical impedance analysis. Obes. Surg.15(2), 183–186 (2005).
- Larson-Meyer DE, Redman L, Heilbronn LK, Martin CK, Ravussin E. Caloric restriction with or without exercise: the fitness versus fatness debate. Med. Sci. Sports Exerc.42(1), 152–159 (2010).
- Wadden TA, Berkowitz RI, Womble LG, Sarwer DB, Arnold ME, Steinberg CM. Effects of sibutramine plus orlistat in obese women following 1 year of treatment by sibutramine alone: a placebo-controlled trial. Obes. Res.8(6), 431–437 (2000).
- Colman E. Anorectics on trial: a half century of federal regulation of prescription appetite suppressants. Ann. Intern. Med.143(5), 380–385 (2005).
- Coyne TC. Phentermine – resin or salt – there are differences. Arch. Intern. Med.157(20), 2381–2382 (1997).
- Greenway FL, Caruso MK. Safety of obesity drugs. Expert Opin. Drug Saf.4(6), 1083–1095 (2005).
- Kim B. Thyroid hormone as a determinant of energy expenditure and the basal metabolic rate. Thyroid18(2), 141–144 (2008).
- al-Adsani H, Hoffer LJ, Silva JE. Resting energy expenditure is sensitive to small dose changes in patients on chronic thyroidxhormone replacement. J. Clin. Endocrinol. Metab.82(4), 1118–1125 (1997).
- Danforth E Jr, Burger A. The role of thyroid hormones in the control of energy expenditure. Clin. Endocrinol. Metab.13(3), 581–595 (1984).
- The Coronary Drug Project. Findings leading to further modifications of its protocol with respect to dextrothyroxine. The Coronary Drug Project research group. JAMA220(7), 996–1008 (1972).
- Lesses MF, Myerson A. Human autonomic pharmacology. XVI. Benzedrine sulfate as an aid in the treatment of obesity. 1938. Obes. Res.2(3), 286–292 (1994).
- Pasquali R, Casimirri F, Melchionda N et al. Effects of chronic administration of ephedrine during very-low-calorie diets on energy expenditure, protein metabolism and hormone levels in obese subjects. Clin. Sci. (Lond.)82(1), 85–92 (1992).
- Lorello C, Goldfield GS, Doucet E. Methylphenidate hydrochloride increases energy expenditure in healthy adults. Obesity (Silver Spring)16(2), 470–472 (2008).
- Jones JR, Caul WF, Hill JO. The effects of amphetamine on body weight and energy expenditure. Physiol. Behav.51(3), 607–611 (1992).
- Lang SS, Danforth E Jr, Lien EL. Anorectic drugs which stimulate thermogenesis. Life Sci.33(13), 1269–1275 (1983).
- Bushnell PJ. Differential effects of amphetamine and related compounds on locomotor activity and metabolic rate in mice. Pharmacol. Biochem. Behav.25(1), 161–170 (1986).
- Crowley WR, Ramoz G, Keefe KA, Torto R, Kalra SP, Hanson GR. Differential effects of methamphetamine on expression of neuropeptide Y mRNA in hypothalamus and on serum leptin and ghrelin concentrations in ad libitum-fed and schedule-fed rats. Neuroscience132(1), 167–173 (2005).
- Jaworska L, Budziszewska B, Lason W. The effect of repeated amphetamine administration on the proopiomelanocortin mRNA level in the rat pituitary: an in situ hybridization study. Drug Alcohol Depend.36(2), 123–127 (1994).
- Menyhert J, Wittmann G, Lechan RM, Keller E, Liposits Z, Fekete C. Cocaine- and amphetamine-regulated transcript (CART) is colocalized with the orexigenic neuropeptide Y and agouti-related protein and absent from the anorexigenic a-melanocyte-stimulating hormone neurons in the infundibular nucleus of the human hypothalamus. Endocrinology148(9), 4276–4281 (2007).
- Balcioglu A, Wurtman RJ. Effects of phentermine on striatal dopamine and serotonin release in conscious rats: in vivo microdialysis study. Int. J. Obes. Relat. Metab. Disord.22(4), 325–328 (1998).
- Alexander M, Rothman RB, Baumann MH, Endres CJ, Brasic JR, Wong DF. Noradrenergic and dopaminergic effects of (+)-amphetamine-like stimulants in the baboon Papio anubis. Synapse56(2), 94–99 (2005).
- Matera C, Wardlaw SL. Dopamine and sex steroid regulation of POMC gene expression in the hypothalamus. Neuroendocrinology58(5), 493–500 (1993).
- Hirsch J, Mackintosh RM, Aronne LJ. The effects of drugs used to treat obesity on the autonomic nervous system. Obes. Res.8(3), 227–233 (2000).
- Kim KK, Cho HJ, Kang HC, Youn BB, Lee KR. Effects on weight reduction and safety of short-term phentermine administration in Korean obese people. Yonsei Med. J.47(5), 614–625 (2006).
- Aronne LJ, Halseth AE, Burns CM, Miller S, Shen LZ. Enhanced weight loss following coadministration of pramlintide with sibutramine or phentermine in a multicenter trial. Obesity (Silver Spring) DOI: 10.1038/oby.2009.478 (2010) (Epub ahead of print).
- Maryanoff BE. Sugar sulfamates for seizure control: discovery and development of topiramate, a structurally unique antiepileptic drug. Curr. Top. Med. Chem.9(11), 1049–1062 (2009).
- Eliasson B, Gudbjornsdottir S, Cederholm J, Liang Y, Vercruysse F, Smith U. Weight loss and metabolic effects of topiramate in overweight and obese Type 2 diabetic patients: randomized double-blind placebo-controlled trial. Int. J. Obes. (Lond.)31(7), 1140–1147 (2007).
- Khanna V, Arumugam S, Roy S, Mittra S, Bansal VS. Topiramate and Type 2 diabetes: an old wine in a new bottle. Expert Opin. Ther. Targets12(1), 81–90 (2008).
- Beydoun A. Monotherapy trials of new antiepileptic drugs. Epilepsia38(Suppl. 9), S21–S31 (1997).
- Leombruni P, Lavagnino L, Fassino S. Treatment of obese patients with binge eating disorder using topiramate: a review. Neuropsychiatr. Dis. Treat.5, 385–392 (2009).
- Shorvon SD. Safety of topiramate: adverse events and relationships to dosing. Epilepsia37(Suppl. 2), S18–S22 (1996).
- Ben-Menachem E, Axelsen M, Johanson EH, Stagge A, Smith U. Predictors of weight loss in adults with topiramate-treated epilepsy. Obes. Res.11(4), 556–562 (2003).
- Wilding J, Van Gaal L, Rissanen A, Vercruysse F, Fitchet M. A randomized double-blind placebo-controlled study of the long-term efficacy and safety of topiramate in the treatment of obese subjects. Int. J. Obes. Relat. Metab. Disord.28(11), 1399–1410 (2004).
- Stenlof K, Rossner S, Vercruysse F, Kumar A, Fitchet M, Sjostrom L. Topiramate in the treatment of obese subjects with drug-naive Type 2 diabetes. Diabetes Obes. Metab.9(3), 360–368 (2007).
- Bray GA, Hollander P, Klein S et al. A 6-month randomized, placebo-controlled, dose-ranging trial of topiramate for weight loss in obesity. Obes. Res.11(6), 722–733 (2003).
- Astrup A, Toubro S. Topiramate: a new potential pharmacological treatment for obesity. Obes. Res.12(Suppl.), 167S–173S (2004).
- Astrup A, Caterson I, Zelissen P et al. Topiramate: long-term maintenance of weight loss induced by a low-calorie diet in obese subjects. Obes. Res.12(10), 1658–1669 (2004).
- Schutt M, Brinkhoff J, Drenckhan M, Lehnert H, Sommer C. Weight reducing and metabolic effects of topiramate in patients with migraine – an observational study. Exp. Clin. Endocrinol. Diabetes118(7), 449–452 (2010).
- Mizielinska SM. Ion channels in epilepsy. Biochem. Soc. Trans.35(Pt 5), 1077–1079 (2007).
- Braga MF, Aroniadou-Anderjaska V, Li H, Rogawski MA. Topiramate reduces excitability in the basolateral amygdala by selectively inhibiting GluK1 (GluR5) kainate receptors on interneurons and positively modulating GABAA receptors on principal neurons. J. Pharmacol. Exp. Ther.330(2), 558–566 (2009).
- Khazaal Y, Zullino DF. Topiramate-induced weight loss is possibly due to the blockade of conditioned and automatic processes. Eur. J. Clin. Pharmacol.63(9), 891–892 (2007).
- McElroy SL, Arnold LM, Shapira NA et al. Topiramate in the treatment of binge eating disorder associated with obesity: a randomized, placebo-controlled trial. Am. J. Psychiatr.160(2), 255–261 (2003).
- Khazaal Y, Zullino DF. Topiramate in the treatment of compulsive sexual behavior: case report. BMC Psychiatry6, 22 (2006).
- Turenius CI, Htut MM, Prodon DA et al. GABA(A) receptors in the lateral hypothalamus as mediators of satiety and body weight regulation. Brain Res.1262, 16–24 (2009).
- Erondu N, Gantz I, Musser B et al. Neuropeptide Y5 receptor antagonism does not induce clinically meaningful weight loss in overweight and obese adults. Cell Metab.4(4), 275–282 (2006).
- Erondu N, Wadden T, Gantz I et al. Effect of NPY5R antagonist MK-0557 on weight regain after very-low-calorie diet-induced weight loss. Obesity (Silver Spring)15(4), 895–905 (2007).
- Husum H, Van Kammen D, Termeer E, Bolwig G, Mathe A. Topiramate normalizes hippocampal NPY-LI in flinders sensitive line ‘depressed’ rats and upregulates NPY, galanin, and CRH-LI in the hypothalamus: implications for mood-stabilizing and weight loss-inducing effects. Neuropsychopharmacology28(7), 1292–1299 (2003).
- Heiman ML, Statnick MA. Galanin-like peptide functions more like leptin than like galanin. Endocrinology144(11), 4707–4708 (2003).
- Theisen FM, Beyenburg S, Gebhardt S et al. A prospective study of body weight and serum leptin levels in patients treated with topiramate. Clin. Neuropharmacol.31(4), 226–230 (2008).
- Tremblay A, Chaput JP. About the appetite-related effects of topiramate. Eur. J. Clin. Pharmacol.63, 893 (2007).
- Picard F, Deshaies Y, Lalonde J, Samson P, Richard D. Topiramate reduces energy and fat gains in lean (Fa/?) and obese (fa/fa) Zucker rats. Obes. Res.8(9), 656–663 (2000).
- Richard D, Picard F, Lemieux C, Lalonde J, Samson P, Deshaies Y. The effects of topiramate and sex hormones on energy balance of male and female rats. Int. J. Obes. Relat. Metab. Disord.26(3), 344–353 (2002).
- Winum JY, Scozzafava A, Montero JL, Supuran CT. Sulfamates and their therapeutic potential. Med. Res. Rev.25(2), 186–228 (2005).
- Poulsen SA, Wilkinson BL, Innocenti A, Vullo D, Supuran CT. Inhibition of human mitochondrial carbonic anhydrases VA and VB with para-(4-phenyltriazole-1-yl)-benzenesulfonamide derivatives. Bioorg. Med. Chem. Lett.18(16), 4624–4627 (2008).
- Vullo D, Franchi M, Gallori E, Antel J, Scozzafava A, Supuran CT. Carbonic anhydrase inhibitors. Inhibition of mitochondrial isozyme V with aromatic and heterocyclic sulfonamides. J. Med. Chem.47(5), 1272–1279 (2004).
- Richard D, Ferland J, Lalonde J, Samson P, Deshaies Y. Influence of topiramate in the regulation of energy balance. Nutrition16(10), 961–966 (2000).
- Wilkes JJ, Nguyen MT, Bandyopadhyay GK, Nelson E, Olefsky JM. Topiramate treatment causes skeletal muscle insulin sensitization and increased Acrp30 secretion in high-fat-fed male Wistar rats. Am. J. Physiol. Endocrinol. Metab.289(6), E1015–E1022 (2005).
- Kadowaki T, Yamauchi T, Kubota N, Hara K, Ueki K, Tobe K. Adiponectin and adiponectin receptors in insulin resistance, diabetes, and the metabolic syndrome. J. Clin. Invest.116(7), 1784–1792 (2006).
- Dridi S, Taouis M. Adiponectin and energy homeostasis: consensus and controversy. J. Nutr. Biochem.20(11), 831–839 (2009).
- Silberstein SD, Neto W, Schmitt J, Jacobs D. Topiramate in migraine prevention: results of a large controlled trial. Arch. Neurol.61(4), 490–495 (2004).
- Lachkar Y, Bouassida W. Drug-induced acute angle closure glaucoma. Curr. Opin. Ophthalmol.18(2), 129–133 (2007).
- Lee GC, Tam CP, Nesh-Meyer HV, Myers JS, Katz LJ. Bilateral angle closure glaucoma induced by sulphonamide-derived medications. Clin. Experiment. Ophthalmol.35(1), 55–58 (2007).
- Sachi D, Vijaya L. Topiramate induced secondary angle closure glaucoma. J. Postgrad. Med.52(1), 72–73 (2006).
- Lai JS, Tham CC, Chua JK et al. To compare argon laser peripheral iridoplasty (ALPI) against systemic medications in treatment of acute primary angle-closure: mid-term results. Eye (Lond.)20(3), 309–314 (2006).
- Mincione F, Scozzafava A, Supuran CT. The development of topically acting carbonic anhydrase inhibitors as anti-glaucoma agents. Curr. Top. Med. Chem.7(9), 849–854 (2007).
- Celebisoy N, Gokcay F, Sirin H, Akyurekli O. Treatment of idiopathic intracranial hypertension: topiramate vs acetazolamide, an open-label study. Acta Neurol. Scand.116(5), 322–327 (2007).
- Mirza N, Marson AG, Pirmohamed M. Effect of topiramate on acid-base balance: extent, mechanism and effects. Br. J. Clin. Pharmacol.68(5), 655–661 (2009).
- Vega D, Maalouf NM, Sakhaee K. Increased propensity for calcium phosphate kidney stones with topiramate use. Expert Opin. Drug Saf.6(5), 547–557 (2007).
- Galicia SC, Lewis SL, Metman LV. Severe topiramate-associated hyperthermia resulting in persistent neurological dysfunction. Clin. Neuropharmacol.28(2), 94–95 (2005).
- Vahedi K, Taupin P, Djomby R et al. Efficacy and tolerability of acetazolamide in migraine prophylaxis: a randomised placebo-controlled trial. J. Neurol.249(2), 206–211 (2002).
- Epstein DL, Grant WM. Carbonic anhydrase inhibitor side effects. Serum chemical analysis. Arch. Ophthalmol.95(8), 1378–1382 (1977).
- Bays H. Adiposopathy: the endocannabinoid system as a therapeutic treatment target for dysfunctional ‘sick’ fat. Californian J. Health Promot.19(1), 32–39 (2007).
- Di Marzo V, Despres JP. CB1 antagonists for obesity – what lessons have we learned from rimonabant? Nat. Rev. Endocrinol.5(11), 633–638 (2009).
- Lee HK, Choi EB, Pak CS. The current status and future perspectives of studies of cannabinoid receptor 1 antagonists as anti-obesity agents. Curr. Top. Med. Chem.9(6), 482–503 (2009).
- Christman DS, Faubion MD. Suicide attempt following initiation of topiramate. Am. J. Psychiatry164(4), 682–683 (2007).
- Patorno E, Bohn RL, Wahl PM et al. Anticonvulsant medications and the risk of suicide, attempted suicide, or violent death. JAMA303(14), 1401–1409 (2010).
- Kuriya B, Schneid EC, Bell CM. Quality of pharmaceutical industry press releases based on original research. PLoS One3(7), e2828 (2008).
- Advokat C. What are the cognitive effects of stimulant medications? Emphasis on adults with attention-deficit/hyperactivity disorder (ADHD). Neurosci. Biobehav. Rev.34(8), 1256–1266 (2010).
- Ryan D, Peterson C, Troupin B, Najarian T, Tam P, Day WP. Weight loss at 6 months with VI-0521 (PHEN/TPM combination) treatment. Presented at: 17th Annual European Congress on Obesity (ECO). Amsterdam, The Netherlands, 6–9 May 2009.
- Aronne LJ, Peterson C, Troupin B, Najarian T, Day W. Weight loss with VI-0521 stops progression toward Type 2 diabetes in obese non-diabetic subjects. Presented at: American Diabetes Association 69th Scientific Session. New Orleans, LA, USA, 5–9 June 2009.
- Garvey WT, Troupin B, Tam P, Najarian T, Peterson C, Day W. One year treatment with VI-0521 in Type 2 diabetes demonstrates continued glycemic improvement and weight loss. Presented at: American Diabetes Association 69th Scientific Session. New Orleans, LA, USA, 5–9 June 2009.
- Garvey WT, Troupin B, Tam P, Najarian T, Day W. Can weight loss treat diabetes? Results of one year of treatment with VI-0521 in obese Type 2 diabetics. Presented at: American Diabetes Association 69th Scientific Session. New Orleans, LA, USA, 5–9 June 2009.
- Sayburn A. Withdrawal of sibutramine leaves European doctors with just one obesity drug. Br. Med. J.340, c477 (2010).
- Bays H. Adiposopathy, metabolic syndrome, quantum physics, general relativity, chaos and the Theory of Everything. Expert Rev. Cardiovasc. Ther.3(3), 393–404 (2005).
- Smith U. Visceral fat, like epicardial fat, is an ectopic fat depot which reflects cardiometabolic risk on obesity. J. Int. Chair Cardiometab. Risk1(2), 17–19 (2008).
- Bays H. Adiposopathy – defining, diagnosing, and establishing indications to treat ‘sick fat’: what are the regulatory considerations? US Endocr. Dis.2, 12–14 (2006).
- Heal DJ, Prow MR, Gosden J, Luscombe GP, Buckett WR. A comparison of various antidepressant drugs demonstrates rapid desensitisation of a 2-adrenoceptors exclusively by sibutramine hydrochloride. Psychopharmacology (Berl.)107(4), 497–502 (1992).
- Sawbridge DT, Fitzgerald R. ‘Lazy, slothful and indolent’: medical and social perceptions of obesity in Europe to the eighteenth Century. Vesalius15(2), 59–70 (2009).
- Adelman J, Freitag FG, Lainez M et al. Analysis of safety and tolerability data obtained from over 1,500 patients receiving topiramate for migraine prevention in controlled trials. Pain Med.9(2), 175–185 (2008).
- Bays HE, Laferrere B, Dixon J et al. Adiposopathy and bariatric surgery: is ‘sick fat’ a surgical disease? A consensus from the adiposopathy and bariatric surgery group. Int. J. Clin. Pract.63(9), 1285–1300 (2009).
- Wilkes JJ, Nelson E, Osborne M, Demarest KT, Olefsky JM. Topiramate is an insulin-sensitizing compound in vivo with direct effects on adipocytes in female ZDF rats. Am. J. Physiol. Endocrinol. Metab.288(3), E617–E624 (2005).
- Bays H. The melanocortin system as a therapeutic treatment target for adiposity and adiposopathy. Drugs R. D.7(5), 289–302 (2006).
- Garfield AS, Heisler LK. Pharmacological targeting of the serotonergic system for the treatment of obesity. J. Physiol.587(Pt 1), 49–60 (2009).
- Qi Y, Takahashi N, Hileman SM et al. Adiponectin acts in the brain to decrease body weight. Nat. Med.10(5), 524–529 (2004).
- Spranger J, Verma S, Gohring I et al. Adiponectin does not cross the blood–brain barrier but modifies cytokine expression of brain endothelial cells. Diabetes55(1), 141–147 (2006).
Websites
- WHO. Global Strategy on Diet, Physical Activity and Health www.who.int/dietphysicalactivity/publications/facts/obesity/en/ (Accessed 24 April 2010)
- GlaxoSmithKline. Augmentin (amoxicillin/clavulanate potassium) Tablets Prescribing Information (revised September 2009) http://us.gsk.com/products/assets/us_augmentin.pdf (Accessed 7 August 2010)
- Pfizer. Motrin (Ibuprofen tablets, USP) Prescribing Information (revised July 2003) www.pfizer.com/files/products/uspi_motrin.pdf (Accessed 7 August 2010)
- Bristol-Myers Squibb Company. Glucophage (metformin hydrochloride) Tablets/Glucophage XR (metformin hydrochloride) Extended-Release Tablets Prescribing Information (revised January 2009; 2010) http://packageinserts.bms.com/pi/pi_glucophage.pdf (Accessed 7 August 2010)
- Wilson L, Day W, Look M, Tam P. EQUIP and CONQUER study results. Vivus Inc. (2009) http://files.shareholder.com/downloads/VVUS/0x0x318291/7cfa4590-cb7d-453d-86a8-c25d5f832bfd/Qnexa.pdf (Accessed 7 August 2010)
- Vivus. VIVUS Press Release: Weight Loss with Qnexa Stops Progression Toward Type 2 Diabetes in Obese Non-Diabetic Patients http://files.shareholder.com/downloads/VVUS/907582005x0x299840/f3d6134f-d6b4–437e-abcf-6c6ef1492227/VVUS_News_2009_6_6_General_Releases.pdf (Accessed 6 June 2009)
- Vivus. VIVUS Press Release: Results From Phase III EQUATE Trial of VIVUS’ Qnexa Highlighted at European Congress on Obesity: Study Results Demonstrated Significant Weight Loss in Obese Patients Treated with Qnexa http://ir.vivus.com/releasedetail.cfm?ReleaseID=382339 (Accessed 7 August 2010)
- Vivus. VIVUS Press Release: Qnexa Shows Significantly Improved Blood Sugar Control Through Weight Loss in Patients with Type 2 Diabetes http://files.shareholder.com/downloads/VVUS/907582005x0x300073/701e40a2–6f0a-4ea8–8d39-ccf23639530e/VVUS_News_2009_6_8_General_Releases.pdf (Accessed 8 June 2009)
- US Food and Drug Administration. Guidance for Industry: Developing Products for Weight Management www.fda.gov/downloads/Drugs/GuidanceComplianceRegulatoryInformation/Guidances/ucm071612.pdf (Accessed 20 May 2010)
- Vivus. VIVUS Press Release. VIVUS Announces Positive Results From Phase II Study of Qnexa in Obstructive Sleep Apnea http://ir.vivus.com/releasedetail.cfm?ReleaseID=434788 (Accessed 7 January 2010)
- VIVUS Inc. VI-0521 (QNEXA) Advisory Committee Briefing Document Link (Accessed 20 July 2010)
- Pollack A. New York Times. FDA Panel Votes Against Obesity Drug www.nytimes.com/2010/07/16/health/16obese.html (Accessed 18 July 2010)
- Young D. BioWorld. FDA Panel Rejects Vivus Obesity Pill Qnexa (16 July 2010) www.bioworld.com/servlet/com.accumedia.web.Dispatcher?next=bioWorldHeadlines_article&forceid=55171 (Accessed 17 July 2010)
- US FDA. Guidance for Industry: Diabetes Mellitus – Evaluating Cardiovascular Risk in New Antidiabetic Therapies to Treat Type 2 Diabetes (2008) www.fda.gov/downloads/Drugs/GuidanceComplianceRegulatoryInformation/Guidances/ucm071627.pdf (Accessed 4 July 2010)
- Vivus. VIVUS Press Release. Qnexa® Treatment Results in Improved Glucose Control in Obese Non-Diabetic Subjects http://files.shareholder.com/downloads/VVUS/907582005x0x263433/1186d22b-c010–4b6b-8af8–72ac310d4f47/VVUS_News_2009_1_12_General_Releases.pdf (Accessed 12 January 2009)
- Vivus. VIVUS Press Release: VIVUS Announces Positive Results From Two Phase III Studies; Obese Patients on Qnexa Achieve Average Weight Loss up to 14.7% and Significant Improvements in Co-Morbidities http://ir.vivus.com/releasedetail.cfm?ReleaseID=407933 (Accessed 7 August 2010)
- ClinicalTrials.gov http://clinicaltrials.gov (Accessed 12 April 2010)
- Vivus Incorporated Press Release. FDA Issues Complete Response Letter to VIVUS Regarding New Drug Application for QNEXA®; VIVUS to Submit Written Response Within Six Weeks http://ir.vivus.com/releasedetail.cfm?ReleaseID=524576 (Accessed 28 October 2010)