Abstract
Over the last decade, pseudoprogression as a clinically significant entity affecting both glioma patient management and the conduct of clinical trials has been recognized as a significant issue. The authors have summarized the literature relative to the incidence, chronological sequence, therapy-relatedness, impact of O-6-methylguanine-DNA methyltransferase methylation status and clinical features of pseudoprogression. Evidence regarding numerous neuroradiologic techniques to differentiate pseudoprogression from tumor recurrence is summarized. The implications of pseudoprogression on prognosis and clinical trial design are substantial, and are reviewed. Relative to this, the overlapping terms pseudoprogression and radiation necrosis are clarified to produce an appropriate basis for future consideration and research regarding this important biological phenomenon.
Medscape: Continuing Medical Education Online
This activity has been planned and implemented in accordance with the Essential Areas and policies of the Accreditation Council for Continuing Medical Education through the joint sponsorship of Medscape, LLC and Expert Reviews Ltd. Medscape, LLC is accredited by the ACCME to provide continuing medical education for physicians.
Medscape, LLC designates this Journal-based CME activity for a maximum of 1 AMA PRA Category 1 Credit(s)™. Physicians should claim only the credit commensurate with the extent of their participation in the activity.
All other clinicians completing this activity will be issued a certificate of participation. To participate in this journal CME activity: (1) review the learning objectives and author disclosures; (2) study the education content; (3) take the post-test with a 70% minimum passing score and complete the evaluation at www.medscape.org/journal/expertneurothera; (4) view/print certificate.
Release date: 2 April 2013; Expiration date: 2 April 2014
Learning objectives
Upon completion of this activity, participants will be able to:
• Analyze the prognosis of high-grade gliomas and the risk of radiation necrosis
• Distinguish the risk of pseudoprogression after chemoradiotherapy of gliomas
• Assess potential risk factors for pseudoprogression after chemoradiotherapy of gliomas
• Evaluate different imaging modalities in their ability to determine early progressive disease from pseudoprogression and post-treatment radiation effects
Financial & competing interests disclosure
EDITOR
Elisa Manzotti
Publisher, Future Science Group, London, UK.
Disclosure: Elisa Manzotti has disclosed no relevant financial relationships.
CME AUTHOR
Charles Vega, MD
Freelance writer and reviewer, Medscape, LLC
Disclosure: Charles Vega, MD, has disclosed no relevant financial relationships.
AUTHORS AND CREDENTIALS
Tim J Kruser, MD
Department of Human Oncology, University of Wisconsin School Of Medicine and Public Health, Madison, WI, USA
Disclosure: Tim J Kruser, MD, has disclosed no relevant financial relationships.
Minesh P Mehta, MD
Department of Radiation Oncology, Northwestern Feinberg School of Medicine, Chicago, IL, USA
Disclosure: Minesh P Mehta, MD, has or has had the following roles in 2011–2012: consultant to Abbott, Bristol-Meyers Squibb, Elekta, Merck, Novartis, Novocure, Tomotherapy and Vertex; stock options in Accuray, Colby and Pharmacyclics; data safety monitoring boards for Apogenix; protocol data review: Adnexus; Board of Directors for Pharmacyclics; Speaker for Merck, priME Oncology, Vindico and WebMD; Royalties from DEMOS Publishers, Elsevier; CME Products with MCM, Medscape.
H Ian Robins, MD, PhD
Department of Human Oncology, University of Wisconsin School Of Medicine and Public Health, Madison, WI, USA
Disclosure: H Ian Robins, MD, PhD, has or has had the following roles in 2011–2012: Consultant to Genentech, Abbott and Novocure.
Sequential contrast-enhanced T1 MRI following radiation plus temozolomide for high-grade glioma are demonstrated. 4 months after completion of chemoradiation, the patient was noted to have progressive contrast enhancement surrounding the resection cavity concerning for tumor recurrence. Temozolomide was continued to complete a 6-month adjuvant course, and imaging findings subsequently improved. The patient now remains without evidence of recurrent disease 3 years postchemoradiation.
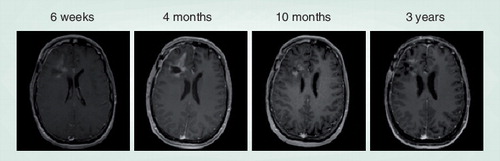
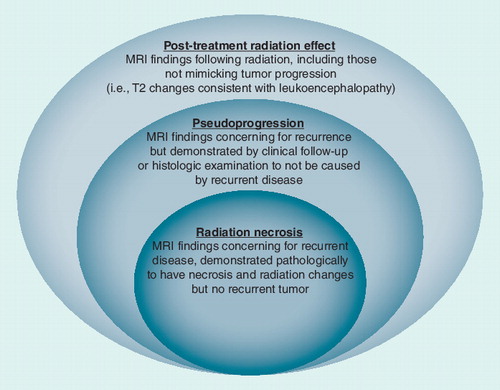
Management of high-grade gliomas (HGGs) has consisted of maximal resection and postoperative radiotherapy (RT) on the basis of randomized controlled trials dating back to the 1970s, which established the survival benefit of postoperative RT Citation[1–3]. Numerous attempts to improve survival have been unsuccessful, including radiation dose escalation Citation[4], stereotactic radiosurgery boost Citation[5], brachytherapy boost Citation[6] and hyperfractionation Citation[7]. Trials attempting to improve efficacy have commonly assessed outcomes not only in terms of overall survival, but also by assessing radiographic response and/or progression-free survival.
These latter end points have been measured using radiographic criteria described by Macdonald et al. in 1990 Citation[8]. The Macdonald criteria were based on contrast-enhanced computed tomography using maximal 2D-enhancing tumor area (the sum of the products of perpendicular diameters), as well as the use of corticosteroids and clinical neurological status to define disease complete response, partial response, stability or progression. These criteria have been widely used in HGG studies, and the described parameters are commonly used in conjunction with MRI findings of contrast enhancement. Historically, disease recurrence was inevitable, and outcomes with salvage treatments following recurrence were disappointing Citation[9].
A relatively recent development has altered the landscape of glioblastoma (GBM) treatment. The landmark study by Stupp et al. Citation[10] demonstrated the superiority of chemoradiotherapy (chemoRT) with concurrent and consolidative temozolomide (TMZ) compared with RT alone, and has established chemoRT as the standard of care for GBM. However, this intensification of therapy has been accompanied by an increased recognition of pseudoprogression (PsP), whereby a proportion of patients demonstrate increased contrast enhancement on follow-up MRI studies, with or without clinical symptoms, which subsequently subside without a change in therapy and therefore do not represent true early progressive disease (ePD) . A portion of the transient changes in T1-contrast MRI may be therapy-induced alterations in the blood–brain barrier. Some of these changes might represent radiation necrosis, as there is evidence that the rates of RT necrosis (a well-recognized phenomenon following RT) are increasing with the incorporation of TMZ into HGG management Citation[11]. Pseudoprogression and RT necrosis (as defined by surgical resection) likely lie along a spectrum of post-treatment radiation effects (PTREs). However, even after resection of a suspect lesion, a pathological diagnosis may not be well defined, as there may be minimal residual disease or sampling problems. At some institutions, the observation of mitoses is required in order for a pathologist to be comfortable with assigning a diagnosis of recurrent disease. Conceptually, one approach is to ask the question: “What significant part of the resected specimen accounted for the MRI changes?” Clearly, this increase in PTRE confounds follow-up MRI findings following glioma therapy, and as such, compromises the conduct of clinical trials.
Further complicating MRI interpretation is the established use of bevacizumab in the setting of recurrent GBM Citation[12]. This antiangiogenic therapy can normalize leaky tumor vasculature with an associated decrease in T1 enhancement (often referred to as pseudoresponse); this too further complicates the assessment of disease progression Citation[13]. Additionally, MRI interpretation is also potentially compromised by the use of bevacizumab in that it is a useful treatment modality for RT necrosis Citation[14].
Such issues, as well as other long-standing known limitations of the Macdonald criteria, led the Response Assessment in Neuro-Oncology Working Group to develop updated response criteria for HGG Citation[15]. While these new criteria will help guide clinical practice, much uncertainty remains when assessing response of new therapeutic interventions, as no neuroradiological techniques have to date been prospectively validated to distinguish PTRE from progressive disease. The purpose of this review is to summarize data regarding the incidence of PTRE with a focus on PsP and a clarification of the overlapping concepts of PsP and necrosis. As a corollary to this, the current clinical criteria and current neuroradiologic techniques that may hold the potential to further delineate PTRE, and more specifically PsP, from true tumor progression are presented.
Clinical significance
GBM remains a devastating diagnosis, with long-term survival extremely rare. Nonetheless, significant progress has been made over the past decade. A recent analysis of New Approaches to Brain Tumor Therapy (NABTT) Consortium data reflects this, as GBM patients enrolled on Phase II protocols of adjuvant therapies prior to the platform of concurrent TMZ with adjuvant RT demonstrated a 2-year survival rate of 8%. More recent Phase II studies building upon adjuvant chemoRT with TMZ have shown 2-year survival rates of 37% among patients with similar prognostic and demographic factors treated at the same participating institutions Citation[16]. Therefore, with improving outcomes over time, enthusiasm for radiographic follow-up and utilization of salvage therapies following upfront therapy is increasing. However, practice patterns for post-chemoRT imaging surveillance remain diverse and without definitive guidelines Citation[17].
With better outcomes and increasing use of post-RT surveillance imaging, accurate determination of true tumor progression, as opposed to PTRE, is important in clinical decision making. When early imaging changes are noted following adjuvant chemoRT, a decision must be made whether to continue adjuvant TMZ. Whether adherence to adjuvant TMZ impacts outcomes is currently unknown. Patients randomized to the TMZ arm in the Phase III EORTC study received a median of three cycles of adjuvant TMZ, with only 47% receiving the recommended six cycles Citation[10]. The vast majority (73%) of the patients discontinuing adjuvant TMZ within the first 6 months did so because of apparent disease progression, although the phenomenon of PsP was not well recognized at that time. One report has suggested that the adjuvant TMZ does have a survival benefit over nitrosurea-based chemotherapy Citation[18], raising the possibility that early discontinuation of TMZ because of PsP may have negatively impacted the outcomes of patients on the EORTC study. Alternatively, a study by Combs et al., which did not segregate patient on the basis of O-6-methylguanine-DNA methyltransferase (MGMT) methylation status, suggested that adjuvant TMZ (i.e., post-RT-TMZ) may not have a profound effect on outcome Citation[19].
As efficacy of salvage chemotherapies for recurrent HGG is generally disappointing, appropriate recognition of PsP is necessary to prevent errant discontinuation of adjuvant TMZ. Stricter adherence to adjuvant TMZ in the setting of early post-chemoRT imaging changes (some of which represents PsP) may in part have contributed to the improved outcomes on recent NABTT Phase II studies Citation[16]. However, even with continuation of adjuvant TMZ beyond 6 months, only approximately 35% of the patients remain without progression at 12 months Citation[20]. Patients with progressive HGG should ideally be enrolled in clinical trials. Clinical trials in this setting do not typically require histologic proof of recurrent disease. Thus, the possibility exists that patients with PsP that are inaccurately deemed to have ePD could be enrolled erroneously, overestimating the value of study agents in Phase II trials. In an attempt to obviate this confounding effect, current Radiation Therapy Oncology Group trials for newly diagnosed GBM discourage declaring ePD on the first post-RT MRI. Additionally, some Adult Brain Tumor Consortium trials require patients to be 3 months post-RT to enter a recurrent study. While this decreases the likelihood of a patient with PsP entering a recurrent study, it may prevent some patients with true ePD from entering clinical trials. Furthermore, PsP can occur later than 3 months, and such patients should be evaluated on a case-by-case basis prior to clinical trial enrollment. Given the cost, potential morbidity and possible sampling errors associated with biopsy or re-resection to establish a diagnosis of recurrence, noninvasive means of accurately distinguishing PsP from ePD are clearly needed.
Clinical experience & incidence
Pseudoprogression in the pre-temozolomide era
Transient or nonprogressive increases in contrast enhancement after RT, often with reversible neurological deterioration, were recognized before the introduction of concomitant RT and TMZ as standard therapy for HGG. An early report by Hoffman et al. Citation[21] analyzed 51 glioma patients who, within a prospective trial, had been treated with 60 Gy and BCNU ± hydroxurea, and had all survived at least 26 weeks. During the first 18 weeks, 25 out of 51 patients (49%) had findings (progression/worsening on at least two imaging or clinical factors) that were presumed to represent tumor progression. Seven of these 25 patients (28%) improved with no change in therapy. Notably, deterioration or worsening of findings after 18 weeks rarely improved.
A Norwegian report demonstrated that six out 112 patients (5.4%) with inoperable brain tumors (49 HGG; 63 low-grade gliomas) treated with intra-arterial chemotherapy followed by 54-Gy radiation developed transient radiation reactions 2–8 months later Citation[22]. Interestingly, they observed a mild form of reaction noted at 2–3 months following RT characterized by low-attenuation expansive areas within the irradiated volume, as well as a more severe form in some patients 6 months or more after RT, characterized by enhancing lesions and exacerbation of clinical signs, which likely represents radiation necrosis.
Similarly, other studies Citation[23–26] have demonstrated reactions following RT mimicking tumor progression, with subsequent stabilization or improvement. The most rigorous study of this phenomenon utilizing MRIs prior to TMZ investigated 32 patients with HGG on prospective studies treated with postoperative RT alone (control patients) at a single center in The Netherlands Citation[27]. Nine out of 32 (28%) patients demonstrated findings worrisome for progression on their first post-RT MRI scan (within 4 weeks). In three of these nine (33%; 9% of total cohort), the patients either improved or stabilized for at least 6 months following the initial concerning post-RT MRI scan. With these observations, the authors suggested that it would be prudent to exclude patients with recurrence within 3 months of RT from trials for recurrent HGG unless histologic proof of recurrence was obtained.
Pseudoprogression with radiation plus temozolomide
The first large report of early post-RT changes mimicking progressive tumor following chemoRT with TMZ was reported by Chamberlain et al. in 2007 Citation[28]. ChemoRT with TMZ was administered to 51 patients, who were re-evaluated with MRI 2–3 weeks after completion of RT. Of 26 patients (51%) with clinical and radiographic progression within 6 months, 15 underwent re-biopsy, with seven patients demonstrating no evidence of tumor at a median of 3 months following chemoRT. This observation raised the awareness of early post-RT changes mimicking tumor progression in the setting of RT with TMZ. The authors felt that these exaggerated changes may have occurred due to the radiosensitization effect of TMZ Citation[29] in this setting, mirroring the enhanced antitumor efficacy demonstrated in the EORTC trial Citation[10].
The term ‘pseudoprogression’ was subsequently introduced and further characterized in a larger series analyzed by Taal et al. Citation[30]. In 85 patients undergoing RT plus TMZ for malignant glioma, progression of ≥25% on MRI at 4 weeks post-RT was noted in 36 patients (42%). Of these 36 patients, half (n = 18) had either at least a 50% decrease in the enhancing lesion during further follow-up or remained clinically and neurologically stable for at least 6 months without any further treatment other than adjuvant TMZ, and were deemed to have PsP.
As clinical awareness of PsP has risen, a large body of literature has helped to further define the incidence and factors associated with PsP in the setting of RT plus TMZ for HGG Citation[11,31–45]. These observed rates of early progression and PsP noted in these studies are summarized in . Individual studies have differed in the time point at which early progression is defined, as well as the criteria used to define early progression. Furthermore, strict criteria do not exist to define subsequent stabilization/improvement that qualifies individual patients with early signs of progression as pseudoprogressors at later time points, and therefore variability exists between studies. Nonetheless, it appears that approximately 50% of the HGG patients treated with adjuvant RT plus TMZ can be expected to have early (1–6 months) post-RT imaging findings concerning for progression. Of these early progressors, approximately 40% improve or stabilize on subsequent clinical and radiographic assessments without a change in therapy, and are deemed to have PsP. Therefore, PsP is documented in nearly 20% of the HGG patients treated with adjuvant RT plus TMZ.
The precise contribution of TMZ to PsP remains poorly defined. As above, observations consistent with PsP existed prior to the routine incorporation of TMZ into adjuvant HGG therapy. However, many of these studies predated the regular use of post-therapy MRI, and the lower rates observed could thus be explained by less sensitive (computed tomography, clinical examination) measures of apparent early progression. Gerstner et al. Citation[35] examined this question by comparing rates of PsP in 47 glioblastoma patients treated with adjuvant RT alone and 45 patients treated with adjuvant RT plus TMZ. On the first post-RT MRI scan, 18 out of the 47 (38%) RT-only patients demonstrated enlargement, with 11 of these 18 (61%; 23% of entire RT-only cohort) subsequently found to have PsP. In the chemoRT patients, 24 out of 45 RT (53%) patients had enlargement on the first post-RT MRI, with 13 of these 24 (54%; 29% of entire RT plus TMZ cohort) subsequently deemed to have PsP. The odds ratio for PsP in the RT plus TMZ patients was 1.3 versus RT alone (95% CI: 0.52–3.4), which was not statistically significant (p = 0.55). Therefore, while it is likely that TMZ contributes to the development of PsP, the magnitude of its effect has not been established. In addition, it is likely that more rigorous post-therapy imaging has contributed to higher rates of PsP development/detection in recent years.
Pseudoprogression as a prognostic indicator
As summarized in , numerous studies Citation[36,37,41,42] have demonstrated statistically significant differences in overall survival for patients experiencing PsP compared with ePD. Brandes et al., as described below, demonstrated that the patients with MGMT promoter methylation (a known positive prognostic factor) are more likely to demonstrate PsP than those without MGMT promoter methylation Citation[46]. However, when accounting for MGMT status in a multivariate model, development of PsP remained independently associated with improved survival (p = 0.045). These findings have led to the belief that the clinical entity of PsP is a marker of enhanced antitumor efficacy in the era of concurrent TMZ, in keeping with the well-demonstrated overall survival benefit associated with TMZ. While logical, this conclusion appears premature, as patients with PsP have often not been demonstrated to have statistically improved outcomes compared with the patients with stable or improved post-RT MRIs , and in some studies their outcomes appear less impressive than those with neither PsP or ePD Citation[38,41,45]. Additionally, this conclusion would require evidence that TMZ increases the rates of PsP in HGG over RT alone. As described above, such evidence at this time is not supported in the literature Citation[35].
Determination of PTRE versus ePD
Chemoradiation-induced PsP and/or necrosis present with MRI findings indistinguishable from tumor recurrence on conventional contrast-enhanced MRI. However, while ePD indicates treatment failure and necessitates a change in therapy, PTRE indicates success of the treatment. Therefore, determination of ePD versus PTRE is vital. Numerous studies have investigated novel imaging modalities and parameters to distinguish PTRE from ePD and are reviewed below. Additionally, in recent studies highlighting the phenomenon of PsP, a number of clinical and tumor factors have been studied to assess the likelihood of whether an individual patient with early radiographic progression is demonstrating true ePD or PsP. However, given the small numbers of patients in individual studies, few factors have been identified. The presence of neurologic symptoms and tumor factors, that is, primarily MGMT status, appear to predict the likelihood of PsP as opposed to ePD. Whether anatomic location and/or treatment volume are related to the likelihood of PsP or ePD has not been studied, but also may warrant consideration in future studies.
Symptoms
Numerous reports have suggested that ePD is more likely to be accompanied by symptoms than radiographic changes seen with PsP. Taal et al. noted that 67% of the patients with ePD had accompanying neurologic deterioration, while only 33% of those with PsP had neurologic deterioration (p = 0.094 by Fisher’s exact test) Citation[30] . A similar trend was demonstrated in an Australian report Citation[36], with apparent clinical progression accompanying radiographic findings ultimately deemed PsP in only three of 14 patients (21%), whereas 14 of 27 (52%) of those with ePD were symptomatic (p = 0.096). Brandes et al. noted clinical deterioration in 21 out of 50 patients (42%) with enlarged imaging findings at 1 month post-chemoRT Citation[46]. Patients with PsP again appeared less likely to have accompanying symptoms (11 out of 32; 34%) than those with ePD (ten out of 18; 56%), although again this difference was not statistically different (p = 0.14).
Tumor factors (MGMT & others)
In addition to having prognostic value in patients undergoing chemoRT with TMZ Citation[47], promoter methylation of the MGMT gene has been reported to be associated with the development of PsP. Brandes et al. demonstrated that among patients with early changes and MGMT methylation, 91% had PsP, compared with 41% of the patients with unmethylated MGMT (p = 0.0002) Citation[46]. Of the 36 patients with MGMT methylation, 21 (58%) developed PsP, while only 11 out of the 67 patients with unmethylated MGMT promoters developed PsP (p = 0.00001 by Fisher’s exact test). Therefore, these data suggest that the patients with MGMT methylation are more likely to develop PsP, and also suggests that the vast majority (91%) of early imaging changes in patients with MGMT methylation represent PsP rather than ePD. Two more recent studies also demonstrated that patients with methylated MGMT had a significantly higher incidence of PsP than those with unmethylated MGMT Citation[38,39].
Other tumor biomarkers may be related to the development of PsP, although the evidence is weaker and the value to the clinician less certain. A Korean study examined p53 overexpression, and demonstrated that seven out of eight (88%) tumors with PsP had p53 overexpression, while only three out of ten tumors (30%) with ePD showed overexpression of p53 (p = 0.03) Citation[37]. However, another study examined p53 levels and showed no predictive value in the identification of PsP Citation[40]. This group, however, demonstrated that Ki67, a marker of cellular proliferation, was significantly higher in patients with PsP (median: 20%) compared with Ki67 levels in patients with ePD (median: 10%). All patients with PsP had tumors demonstrating Ki67 indices ≥20%, and the authors postulate that tumors with higher levels of cellular replication may show more significant early treatment effects. Further studies will be needed to assess the value of p53 and Ki67 as related to PsP development.
Imaging determination of post-treatment radiation effect versus tumor recurrence
Conventional MRI
Currently, no neuroradiographic techniques have been prospectively investigated with enough demonstrable sensitivity and specificity to reliably differentiate between PsP and ePD. While certain radiographic patterns on standard MRI sequences (axial T1, T2 and post-gadolinium T1-weighted) such as involvement of the corpus callosum with either subependymal spread or multiple enhancing lesions are more consistent with progressive disease than PsP Citation[48], these patterns are not prevalent or reliable enough to categorize all early radiographic changes in HGG patients as either postradiation change or ePD. A more recent study rigorously examined the utility of conventional MRI in differentiating PsP from ePD Citation[45]. This study of 321 patients analyzed 11 MRI features on the initial post-RT MRI and correlated these with the final diagnosis of PsP or ePD. Only subependymal enhancement was predictive for early progression (p = 0.001), but the negative-predictive value was only 41.8%, while the other ten features had no predictive value. These results highlight the similarities between PsP and ePD on conventional MRI, and the difficulty clinicians and neuroradiologists face when assessing these studies.
Thus, with conventional MRI, PsP can only be retrospectively ascertained. Advanced imaging techniques are currently being investigated to differentiate PsP from ePD, and include MR spectroscopy, diffusion-weighted MRI (DWI), MR perfusion imaging, diffusion-tensor imaging and PET-based strategies .
Diffusion-weighted MRI
DWI measures the degree of water diffusion within tissue, and has important utility in the diagnosis of acute stroke. Apparent diffusion coefficient (ADC) values quantify the mobility of water molecules at the cellular level, holding the potential to differentiate between necrosis, edema and recurrent tumor. Areas with high cellularity are known to have decreased ADC values, but necrosis, gliosis and fibrous scar tissue can also impact the ADC values in a region. The use of DWI for distinguishing treatment-related change from tumor recurrence was first investigated by Hein et al., who retrospectively established ADC maps from diffusion-weighted MRI in 18 HGG patients with enhancing lesions suspicious for recurrence following RT Citation[49]. Mean ADC values and ADC ratios (ADC of enhancing lesion to ADC of contralateral normal white matter) were determined, and recurrence or radiation injury was established either by histology or clinical course and subsequent imaging studies. ADC values and ADC ratios in the recurrent group were significantly lower than those in the nonrecurrent group.
These findings were mirrored by a prospective study from Japan of 20 lesions in 17 patients (14 with HGG) who had MRI findings consistent with those of tumor recurrence Citation[50]. DWI showed marked hypointensity in eight of the 12 radiation necrosis lesions, but none of the eight areas of recurrence demonstrated marked hypointensity. By examining five regions of interest within each area of contrast enhancement, it was demonstrated that the maximal ADC values were significantly smaller for the recurrence group than for the necrosis group. However, it should be noted that cases of radiation necrosis with low ADC values have been reported Citation[51]. The use of contrast-enhanced susceptibility-weighted imaging to guide regions for ADC measurements may further enhance the ability of DWI to differentiate tumor recurrence from PsP Citation[52]. These techniques are promising, with results suggesting a role for DWI in this arena, but further studies of a prospective nature are needed for validation.
MR perfusion
Dynamic susceptibility-weighted contrast-enhanced MRI (DSC) is a T2*-weighted technique to measure relative cerebral blood volume (rCBV) while dynamic contrast-enhanced MRI is a T1-based technique to allow for measurement of vascular permeability in tumors. However, rCBV can be modeled from dynamic contrast-enhanced data. These techniques allow for measurements of the vascular environment to determine levels of absolute cerebral blood flow, cerebral blood volume (CBV) and rCBV. Changes over time suggestive of tumor progression include increased blood volume and blood flow. This technique is increasingly used in determining recurrent tumor from radiation changes in areas of contrast enhancement. Hu et al. obtained DSC perfusion MRI prospectively in 13 HGG patients with contrast-enhancing lesions on follow-up MRI for whom resection was planned Citation[53]. DSC perfusion MR studies were obtained preoperatively, and stereotactic locations of biopsy were captured and coregistered to the preoperative perfusion imaging sets. The rCBV values at the biopsy sites were compared with the histopathology. Areas of post-treatment-related enhancement showed relative CBV values from 0.21 to 0.71, while CBV values for recurrent tumor ranged from 0.55 to 4.64. By choosing a value of 0.71, differentiation of the recurrent tumor from post-treatment-related enhancement was accomplished with a sensitivity of 91.7% and a specificity of 100%, albeit in only 13 patients.
These findings have been corroborated by other studies Citation[54–59]. Kong et al. prospectively analyzed 90 patients with GBM treated with adjuvant chemoRT, with 59 patients showing new or enlarging enhancing lesions following therapy Citation[38]. Mean rCBV values were significantly lower for PsP than for ePD (p = 0.003). A cutoff value for the rCBV ratio was determined, which allowed for 81.5% sensitivity and 77.8% specificity in determining PsP from ePD. Another group similarly showed that patients with PsP had lower median rCBV values compared with ePD; one rCBV threshold detected PsP with 100% sensitivity and 75% specificity, while another threshold achieved 100% specificity to detect PsP, but only 69% specificity Citation[60]. These studies highlight the potential for DSC MR perfusion to differentiate PsP from ePD. However, while many of these studies obtained these images in a prospective fashion, the rCBV threshold values have differed between studies, with values of 0.71 Citation[53], 1.49 Citation[38] and 1.75 Citation[54] reported in different studies. Therefore, optimal thresholds must be determined and validated prospectively to confirm that accuracy is sufficient for clinical use.
The issue of vascular leak in DSC perfusion MR studies deserves attention, as chemoRT can disrupt the blood–brain barrier, which may allow gadolinium-based contrast agents to leak into the interstitial fluid. This may lead to the underestimation of rCBV Citation[61]. Gahramanov et al. have examined ferumoxytol as a contrast agent as an alternative to gadolinium-based contrast in patients with apparent recurrent HGG on conventional MRI, as this iron oxide nanoparticle has a relative inability to cross the disrupted blood–brain barrier Citation[54,59]. In a study of 19 patients with apparent HGG recurrence on conventional MRI, rCBV values ≤1.75, as determined by feromoxytol, were associated with a median survival of 591 days, whereas rCBV values >1.75 were associated with a median survival of 163 days (p < 0.001). While subsequent determination of PsP or ePD was not specifically reported, this suggests that the perfusion MRI with ferumoxytol may distinguish between ePD and PsP, and appears to be a good prognostic tool in patients with conventional MR findings suggestive of recurrence following chemoRT for HGG Citation[59].
In addition to analysis of post-treatment imaging, DSC-MRI may have predictive utility when obtained during chemoRT. Tsien et al. hypothesized that voxel-by-voxel early change in function analysis would be more accurate than standard DSC-MRI for predicting outcomes Citation[43]. DSC-MRI were obtained in 27 HGG patients prior to and during weeks 1 and 3 of chemoRT on a prospective study. Average percent change of rCBV and cerebral blood flow were evaluated, and a voxel-by-voxel analysis (parametric response mapping) Citation[62] was performed in each patient. Fourteen patients were noted to have radiographic progression in the first 3 months, and six of these patients were noted to have PsP as determined by subsequent clinical course or resection showing RT effects only with no tumor. In patients with genuinely progressive disease, the decrease of rCBV at week 3 compared with baseline was significantly less than it was in either patients with PsP or stable findings. These findings indicate that DSC-MRI may have predictive value for subsequent PsP or ePD when obtained during the RT course.
Proton MR spectroscopic imaging (1H MRSI)
MR spectroscopy (MRS) can detect different metabolites in tissue. Metabolites commonly detected in the brain include choline (Cho), N-acetylaspartate (NAA), creatinine (Cr), lipid and lactate. Tumors typically demonstrate elevated Cho levels due to increased cell membrane phospholipids, with decreased NAA in comparison with normal white matter, while necrotic tissue shows elevated lipid and lactate peaks. MRSI has been investigated in differentiating PTRE from ePD. Early studies utilized single-voxel MRS, which assesses metabolite concentrations at a single voxel, but leads to difficulties in interpretation given the heterogeneity of contrast-enhancing lesions in the post-RT setting, with results rarely completely discriminatory Citation[63]. Pure tumor or pure necrosis were often clearly differentiated, but areas with mixed components were difficult to characterize via MRS Citation[64,65].
However, multivoxel MRS techniques may improve accuracy in differentiating PTRE from ePD. Weybright et al. utilized 2D chemical shift imaging MRS in 29 patients with new contrast-enhancing lesions in the region of previously treated brain tumors (24/29 gliomas) Citation[66]. Mean Cho/Cr ratios were higher for tumors compared with PTRE, and when a cutoff value was retrospectively applied, 27 of the 28 patients could be correctly differentiated between recurrence and PTRE. Zeng et al. examined multivoxel 3D-MRS in 28 patients with new contrast-enhancing lesions in the region of previously resected and irradiated HGG Citation[67]. The Cho/NAA and Cho/Cr ratios were significantly higher in recurrent tumor than in PTRE. The sensitivity, specificity and diagnostic accuracy of 3D-MRS were 94.1, 100 and 96.2%, respectively.
MRS has also been combined with DWI to assess PTRE from recurrent tumor Citation[68,69]. In a study of 55 patients, 2D-MRS and DWI were performed, with spectral data for NAA, Cho, Cr, lipid and lactate analyzed in conjunction with the ADC. Similar to other studies, Cho/NAA and Cho/Cr ratios were higher in recurrent tumor than in regions of PTRE, and ADC values and ADC ratios were higher in areas of PTRE than in areas of recurrent tumor Citation[69]. MRS data correctly classified 85.5% of subjects as either PTRE or recurrence, while the addition of ADC information correctly classified 96.4% of subjects. This study and similar studies combining MRS with other MRI techniques Citation[70] demonstrate that combinatorial analyses may be more effective than single-modality analyses for differentiating PTRE from recurrence, but prospective validation is still needed.
PET-based imaging
FDG-PET
Given the difficulty of differentiating PTRE and tumor recurrence with traditional contrast-enhanced MRI sequences, PET has been investigated in this setting. PET imaging with 18F-labeled fluorodeoxyglucose (FDG) has utility in many oncologic settings, but is limited in its applicability in the brain due to high glucose utilization of normal brain that results in high background activity. An early study of FDG-PET evaluated 84 patients with findings suggestive of recurrent intracranial tumor or PTRE. PET had a high rate of both false-positive and negative findings, and the authors concluded that sensitivity and specificity rates for recurrent tumor were unacceptably low Citation[71]. A more recent study demonstrated a sensitivity of only 70% for recurrent glioma with FDG-PET Citation[72]. While HGG typically demonstrate increased glucose metabolism, inflammatory lesions such as post-RT necrosis can also demonstrate increased FDG-PET activity, and in general the amount of overlap in terms of FDG-PET activity between recurrent HGG and RT necrosis is too considerable for FDG-PET to be useful for lesions that are equivocal on MRI Citation[73]. The limitations FDG-PET for differentiating PTRE from tumor recurrence have prompted evaluations of novel PET tracers with lower background brain activity compared with FDG-PET for evaluation of tumor recurrence.
Novel tracers
A number of novel tracers are under investigation in attempts to improve the ability to differentiate PTRE and recurrent HGG. 3´-deoxy-3´-18F-fluoro-thymidine (18F-FLT)-PET has demonstrated promise in detecting recurrent HGG Citation[74], and to prognosticate during treatment for recurrent HGG Citation[75], but has not yet specifically been analyzed for differentiating PTRE and tumor recurrence. More promising are novel amino acid tracers, including 11C-methionine (C-Met)-PET, O-(2-[18F]fluoroethyl)-l-tyrosine (FET)-PET and 18F-3,4-dihydroxy-6-[18F]-fluoro-l-phenylalanine (FDOPA)-PET, while 13N-NH3-PET also demonstrated efficacy in distinguishing recurrent glioma from RT necrosis in one small study Citation[76]. In general, these tracers exhibit a lower background brain activity as compared with FDG-PET.
11C-Met is a widely studied amino acid tracer whose uptake into tumors is mediated by neural l-amino acid transporters Citation[77]. An initial study to evaluate C-Met-PET in distinguishing recurrent glioma from PTRE was performed in 11 patients who had received stereotactic radiosurgery after primary treatment Citation[78]. Histologic examination after C-Met-PET demonstrated recurrent glioma in six cases, while five cases were deemed RT necrosis either via histologic assessment or stable radiographic findings for >5 months. In this small study, C-Met-PET showed a sensitivity of 100%, specificity of 60% and accuracy of 82% for detecting tumor recurrence. This study was promising, but two subsequent studies of C-Met-PET have been conflicting. Terakawa et al. examined 26 patients with glioma Citation[79], and demonstrated that standardized uptake values (SUVs) tended to be higher for tumor recurrence than necrosis. However, sensitivity and specificity were disappointing at only 75 and 75%, respectively. A more recent, larger study directly compared C-Met-PET and FDG-PET for the evaluation of recurrence in 35 patients with primary brain tumors Citation[80]. C-Met-PET was shown to be superior to FDG-PET, with a sensitivity of 95% and specificity of 89%, and also demonstrated better interobserver agreement than FDG-PET. However, C-MET-PET appears to be less effective than DW-MRI for differentiating PTRE from tumor recurrence in HGG under direct comparison Citation[58]. 11C-Met has a short half-life (20 min), limiting its availability, and more studies of this tracer are needed before routine clinical use can be justified.
FET is an 18F-labeled synthetic amino acid with a much longer half-life, and therefore does not require an on-site cyclotron. FET-PET has been investigated in the setting of post-treatment glioma recurrence in two studies. Rachinger et al. examined 45 patients with a history of glioma following various therapies Citation[81]. Recurrence was diagnosed either pathologically or by subsequent clinical course in 31 patients, while 14 patients were shown to not have recurrence at time of imaging. Sensitivity and specificity of MRI were 50 and 94%, respectively, while FET-PET had a sensitivity of 100% and specificity of 93%. FET-PET revealed the correct diagnosis in 44 patients, versus 36 patients (out of 45) for MRI, rendering this modality significantly more accurate than MRI. A subsequent study enrolled 31 patients with findings concerning for glioma recurrence on both MRI and FET-PET (SUVmax/background ratio >2), and prospectively determined the positive-predictive value of FET-PET Citation[82]. All patients underwent multimodality-guided biopsy, and FET-PET results were concordant in 26 out of 31 patients, for a positive-predictive value of 84%. Therefore, there remains interest in utilizing FET-PET in future studies to evaluate PTRE and tumor recurrence.
18F-FDOPA is an 18F-labeled dopamine precursor that is attractive for imaging in the setting of suspected tumor recurrence, because as opposed to contrast-enhanced MRI, FDOPA-PET is believed to require active transport mechanisms for tissue uptake rather than depending on blood–brain barrier breakdown. A preliminary study from UCLA (CA, USA) showed that FDOPA-PET is more accurate than FDG-PET for diagnosing gliomas, especially for low-grade tumors Citation[83]. This study also demonstrated that in a second subset of 51 patients, 47 who were being evaluated for possible tumor recurrence, a sensitivity of 97% and specificity of 86% for tumor recurrence could be attained with FDOPA-PET. This group further suggested in another publication that FDOPA-PET may have a complementary role to MRI as FDOPA-PET may detect recurrence earlier than MRI in some cases, and may better differentiate nonenhancing tumor from other causes of MRI T2-weighted signal change such as edema Citation[84]. While these results with FDOPA-PET appear promising, prospective studies cleanly designed to assess the ability of FDOPA-PET for distinguishing PTRE from recurrent disease are still lacking.
PsP & RT necrosis: clinical overlap, operational ambiguity & histologic uncertainty
Current literature demonstrates and continues to propagate the confusion between PsP and RT necrosis. PsP has commonly been defined as a subacute, post-treatment reaction with increased contrast enhancement and edema that mimics tumor progression, but subsequently stabilizes and/or regresses without intervention. Typically, studies have deemed a case as demonstrating PsP if there are concerning imaging studies on the initial post-chemoRT MRI (4–6 weeks post-therapy), but then subsequent scans (often after two more cycles of TMZ) show improvement or stabilization Citation[30,34,35,42,46]. Radiation necrosis has been described as a severe local tissue reaction with disruption of the blood–brain barrier, necrosis and edema, with or without mass effect on MRI Citation[85]. Some authors have contrasted RT necrosis as differing from PsP in that it is classically a late effect, typically occurring 3–12 months following treatment, but with the possibility of occurring years following RT Citation[86,87]. It is assumed that RT necrosis generally stabilizes or worsens rather than showing spontaneous resolution. This contention is derived from the observation that progressive lesions that require surgery for symptomatic management (and do not show tumor recurrence) are typically found to have elements of RT necrosis. While these generalizations may be largely accurate, the defining difference between PsP and RT necrosis is that PsP is a clinical diagnosis, and RT necrosis is a pathologic diagnosis.
Thus, the current operational definition of PsP is based on clinical course in the absence of intervention, that is, repeat surgery or biopsy. The authors argue that all RT necrosis (in the absence of coinciding recurrent tumor) should also be considered a form of PsP. Radiation necrosis can only be diagnosed pathologically. Therefore, biopsy and/or resection that histologically diagnoses RT necrosis precludes assessment of the subsequent clinical course (were it to be unaltered by tissue sampling/resection). Indeed, some clinical studies on PsP have commonly included cases with resection showing RT necrosis Citation[28,30,33,36,43–45], whereas others have defined PsP by imaging findings and subsequent clinical course alone Citation[17,35,37,40,41]. Recent studies have shown that RT necrosis is not uncommonly seen within 6 months following adjuvant chemoRT Citation[28,44,88]; therefore, any attempt to differentiate PsP and RT necrosis based on time from completion of RT is done so arbitrarily.
Furthermore, it is not known whether cases of PTRE that subsequently improve without intervention (the current definition of PsP) were entirely, in part, or not at all caused by RT necrosis, as this cannot be known without tissue confirmation. While it is possible, and indeed likely, that clinically observed PsP is reflecting transient changes in vascular permeability resulting in contrast enhancement without frank necrosis, only tissue analysis can preclude RT necrosis in these situations. As such, the authors argue that RT necrosis is a subset of PsP, rather than definable as a separate entity . Furthermore, it should be noted that PTRE can be present without mimicking glioma recurrence. Rarely, late changes on MRI that mimic recurrence can be demonstrated upon resection to be neither recurrence nor frank necrosis (i.e., CNS tissue with radiation-induced changes).
RT necrosis as a cause of PsP
RT necrosis is a well-recognized side effect following RT for gliomas. The primary risk factor for RT necrosis is total radiation dose; RT necrosis rarely develops at doses lower than 50 Gy when utilizing standard fractions of 1.8–2.0 Gy, and thresholds of 54 Gy have been suggested Citation[89]. Additional risk factors include high dose per fraction Citation[90] and reirradiation to the brain Citation[90]. Furthermore, the use of chemotherapy appears to increase the risk of RT necrosis Citation[91]. Historically, in the absence of chemotherapy, rates of RT necrosis with doses of 60 Gy have approximated 1% Citation[92]. However, these estimates are likely low, as patients with HGG have limited life expectancy, and rates of reoperation and autopsy are low in these patients. Shaw et al. noted a 2-year actuarial risk of RT necrosis of 5% when treating low-grade glioma with 64.8 Gy in 1.8-Gy fractions Citation[93].
In the largest series to date, Ruben et al. examined RT necrosis rates in 426 glioma patients undergoing RT (HGG in 405 patients) Citation[91]. An incidence of 4.9% was noted, with actuarial incidences of 5.1, 9.3 and 13.3% at 1, 2 and 3 years, respectively. The shortest latent period to diagnosis of necrosis was 2.1 months, with a mean interval of 11.6 months; 85% of the cases had manifested by 2 years. Only one of the 154 patients receiving less than 61.2 Gy in 34 fractions developed RT necrosis, while 6.5% receiving 60 Gy in 2-Gy fractions developed RT necrosis.
Some have postulated that PsP and RT necrosis exist at different time points along the same spectrum of PTRE Citation[85], but the relationship between RT necrosis and PsP has not been firmly established to date. As above, the authors argue that RT necrosis is a subset of PsP, and not definable as separate from PsP as it can only be established via pathologic analysis. This dichotomization has been noted by others, including a recent study examining RT necrosis in the setting of adjuvant chemoRT with TMZ. The authors noted that 11 of the 14 patients found to have RT necrosis upon surgical re-resection had MRI evidence of ePD within 4 months of chemoRT, and would have been categorized as having PsP without the surgical procedure to verify necrosis Citation[88].
Conclusion
Clearly, the recognition of PsP is a fundamental issue to providing optimal patient care. Furthermore, ongoing and future clinical studies, both in the newly diagnosed and recurrent setting, must take PsP into account at the time of trial design; failure to do so may cloud results and lead to either underdetection of meaningful advances, or perhaps more likely, wasteful follow-up studies of falsely promising signals based on errant patient enrollment. Neuro-oncologists must consider PsP in patients following adjuvant RT alone or chemoRT. Based on a summary of available clinical reports, approximately 50% of HGG can be expected to manifest early changes (within 6 months) suggestive of progressive disease on post-therapy MRI scans; approximately 40% of these changes (or 18–20% of all HGG patients) will be demonstrated via clinical course and/or pathologic analysis to represent PsP. Pseudoprogression, while most commonly described in HGG, can also be observed in low-grade glioma patients Citation[13]. True ePD appears more likely to be associated with symptoms at the time of concerning MRI findings than PsP. Furthermore, MGMT methylated disease is more than twice as likely to manifest PsP (rather than ePD) in comparison with nonmethylated patients (in the first 3–6 months following adjuvant chemoRT) Citation[46]. Conventional MRI has little value in differentiating PsP from true ePD. While numerous studies of more advanced neuroradiologic techniques have demonstrated considerable potential in differentiating PsP from ePD, the majority of these have been retrospective, and no technique has been prospectively validated. Continued research is obviously needed to help delineate PsP from the standpoint of patient care, as well as the conduct of future clinical trials. Taken collectively, it is hoped that this review will provide a framework for clinicians to approach this increasingly recognized clinical problem in neuro-oncology.
Expert commentary
In this review, the authors highlight the dichotomy between PsP (as defined by clinical course in the absence of intervention, including reoperation or biopsy) and RT necrosis (which can only be defined upon reoperation or biopsy), and suggest that the separation is not clear cut. The authors suggest that most RT necrosis, without simultaneous pathologic demonstration of recurrent tumor, must be considered PsP, regardless of the time interval following RT (or chemoRT). The exception is the instances (rare in the management of HGG) in which radiation necrosis occurs many years following therapy. This scenario is more common in the context of the treatment of head and neck and nasopharyngeal tumors, where there is no question of PsP in such cases, as these diseases do not progress in the brain, and the appropriate diagnosis in this situation remains radiation necrosis. While the clinical awareness of PsP (including early RT necrosis) has risen during a time frame when standard therapy includes chemoRT with TMZ, the role of TMZ in this phenomenon remains undefined.
Five-year view
Patient outcomes in HGG have shown continued gradual improvement over the past 5–10 years as more efficacious salvage therapies have been implemented, such as bevacizumab. Currently, difficulty in determining PTRE from recurrent tumor often leads to a delay in delivery of salvage therapy. Prospective evaluations of advanced neuroradiologic techniques will allow for more accurate delineation of PsP from recurrent tumor, with appropriate delivery of early salvage therapy for those with recurrence, while allowing those without recurrence to avoid toxicity associated with cytotoxic therapy and/or surgical intervention. As such, enhanced detection of recurrent tumor holds the potential to improve survival in patients with early recurrence, while improving quality of life for patients who demonstrate PsP but are without true ePD.
Table 1. Rates of pseudoprogression in studies of radiotherapy plus temozolomide.
Table 2. Median survival (in months) in patients with high-grade glioma undergoing adjuvant radiotherapy with concurrent temozolomide, as assessed by early radiographic findings and subsequent determination of pseudoprogression.
Table 3. Overview of imaging techniques utilized for discriminating between early progressive disease and pseudoprogression.
Key issues
• Intensification of therapy for high-grade glioma (HGG) with utilization of concurrent temozolomide has been accompanied by an increased recognition of pseudoprogression (PsP).
• PsP and radiation necrosis lie along a spectrum of post-treatment radiation effect.
• To date, no neuroradiological techniques have been prospectively validated to distinguish post-treatment radiation effect from progressive disease. However, progress in this area is clearly being made, as reviewed.
• Currently, approximately 50% of the HGG patients treated with adjuvant chemoradiotherapy have early (1–6 months) imaging findings concerning for progression. Approximately 40% of these improve or stabilize, and are deemed to have PsP.
• Therefore, comprehensive review of the available clinical data shows that nearly 20% of HGG patients develop PsP.
• Early signals suggest temozolomide may increase the rates of PsP compared with RT alone, and that PsP may be associated with improved outcome; however, data in regard to these findings are conflicting and more definitive, prospective analyses are needed.
References
- Walker MD, Alexander E Jr, Hunt WE et al. Evaluation of BCNU and/or radiotherapy in the treatment of anaplastic gliomas. A cooperative clinical trial. J. Neurosurg. 49(3 ), 333–343 (1978).
- Kristiansen K, Hagen S, Kollevold T et al. Combined modality therapy of operated astrocytomas grade III and IV. Confirmation of the value of postoperative irradiation and lack of potentiation of bleomycin on survival time: a prospective multicenter trial of the Scandinavian Glioblastoma Study Group. Cancer 47(4 ), 649–652 (1981).
- Walker MD, Green SB, Byar DP et al. Randomized comparisons of radiotherapy and nitrosoureas for the treatment of malignant glioma after surgery. N. Engl. J. Med. 303(23 ), 1323–1329 (1980).
- Nelson DF, Diener-West M, Horton J, Chang CH, Schoenfeld D, Nelson JS. Combined modality approach to treatment of malignant gliomas – re-evaluation of RTOG 7401/ECOG 1374 with long-term follow-up: a joint study of the Radiation Therapy Oncology Group and the Eastern Cooperative Oncology Group. NCI Monogr. 6, 279–284 (1988).
- Souhami L, Seiferheld W, Brachman D et al. Randomized comparison of stereotactic radiosurgery followed by conventional radiotherapy with carmustine to conventional radiotherapy with carmustine for patients with glioblastoma multiforme: report of Radiation Therapy Oncology Group 93-05 protocol. Int. J. Radiat. Oncol. Biol. Phys. 60(3 ), 853–860 (2004).
- Selker RG, Shapiro WR, Burger P et al.; Brain Tumor Cooperative Group. The Brain Tumor Cooperative Group NIH Trial 87-01: a randomized comparison of surgery, external radiotherapy, and carmustine versus surgery, interstitial radiotherapy boost, external radiation therapy, and carmustine. Neurosurgery 51(2 ), 343–355; discussion 355 (2002).
- Prados MD, Wara WM, Sneed PK et al. Phase III trial of accelerated hyperfractionation with or without difluromethylornithine (DFMO) versus standard fractionated radiotherapy with or without DFMO for newly diagnosed patients with glioblastoma multiforme. Int. J. Radiat. Oncol. Biol. Phys. 49(1 ), 71–77 (2001).
- Macdonald DR, Cascino TL, Schold SC Jr, Cairncross JG. Response criteria for Phase II studies of supratentorial malignant glioma. J. Clin. Oncol. 8(7 ), 1277–1280 (1990).
- Nieder C, Adam M, Molls M, Grosu AL. Therapeutic options for recurrent high-grade glioma in adult patients: recent advances. Crit. Rev. Oncol. Hematol. 60(3 ), 181–193 (2006).
- Stupp R, Mason WP, van den Bent MJ et al.; European Organisation for Research and Treatment of Cancer Brain Tumor and Radiotherapy Groups; National Cancer Institute of Canada Clinical Trials Group. Radiotherapy plus concomitant and adjuvant temozolomide for glioblastoma. N. Engl. J. Med. 352(10 ), 987–996 (2005).
- Peca C, Pacelli R, Elefante A et al. Early clinical and neuroradiological worsening after radiotherapy and concomitant temozolomide in patients with glioblastoma: tumour progression or radionecrosis? Clin. Neurol. Neurosurg. 111(4 ), 331–334 (2009).
- Cohen MH, Shen YL, Keegan P, Pazdur R. FDA drug approval summary: bevacizumab (Avastin) as treatment of recurrent glioblastoma multiforme. Oncologist 14(11 ), 1131–1138 (2009).
- Robins HI, Lassman AB, Khuntia D. Therapeutic advances in malignant glioma: current status and future prospects. Neuroimaging Clin. N. Am. 19(4 ), 647–656 (2009).
- Levin VA, Bidaut L, Hou P et al. Randomized double-blind placebo-controlled trial of bevacizumab therapy for radiation necrosis of the central nervous system. Int. J. Radiat. Oncol. Biol. Phys. 79(5 ), 1487–1495 (2011).
- Wen PY, Macdonald DR, Reardon DA et al. Updated response assessment criteria for high-grade gliomas: response assessment in neuro-oncology working group. J. Clin. Oncol. 28(11 ), 1963–1972 (2010).
- Grossman SA, Ye X, Piantadosi S et al.; NABTT CNS Consortium. Survival of patients with newly diagnosed glioblastoma treated with radiation and temozolomide in research studies in the United States. Clin. Cancer Res. 16(8 ), 2443–2449 (2010).
- Sanghera P, Rampling R, Haylock B et al. The concepts, diagnosis and management of early imaging changes after therapy for glioblastomas. Clin. Oncol. (R. Coll. Radiol.) 24(3 ), 216–227 (2012).
- Rhee DJ, Kong DS, Kim WS et al. Efficacy of temozolomide as adjuvant chemotherapy after postsurgical radiotherapy alone for glioblastomas. Clin. Neurol. Neurosurg. 111(9 ), 748–751 (2009).
- Combs SE, Wagner J, Bischof M et al. Postoperative treatment of primary glioblastoma multiforme with radiation and concomitant temozolomide in elderly patients. Int. J. Radiat. Oncol. Biol. Phys. 70(4 ), 987–992 (2008).
- Malkoun N, Chargari C, Forest F et al. Prolonged temozolomide for treatment of glioblastoma: preliminary clinical results and prognostic value of p53 overexpression. J. Neurooncol. 106(1 ), 127–133 (2012).
- Hoffman WF, Levin VA, Wilson CB. Evaluation of malignant glioma patients during the postirradiation period. J. Neurosurg. 50(5 ), 624–628 (1979).
- Watne K, Hager B, Heier M, Hirschberg H. Reversible oedema and necrosis after irradiation of the brain. Diagnostic procedures and clinical manifestations. Acta Oncol. 29(7 ), 891–895 (1990).
- Boldrey E, Sheline G. Delayed transitory clinical manifestations after radiation treatment of intracranial tumors. Acta Radiol. Ther. Phys. Biol. 5, 5–10 (1966).
- Graeb DA, Steinbok P, Robertson WD. Transient early computed tomographic changes mimicking tumor progression after brain tumor irradiation. Radiology 144(4 ), 813–817 (1982).
- Griebel M, Friedman HS, Halperin EC et al. Reversible neurotoxicity following hyperfractionated radiation therapy of brain stem glioma. Med. Pediatr. Oncol. 19(3 ), 182–186 (1991).
- Rider WD. Radiation damage to the brain – a new syndrome. J. Can. Assoc. Radiol. 14, 67–69 (1963).
- de Wit MC, de Bruin HG, Eijkenboom W, Sillevis Smitt PA, van den Bent MJ. Immediate post-radiotherapy changes in malignant glioma can mimic tumor progression. Neurology 63(3 ), 535–537 (2004).
- Chamberlain MC, Glantz MJ, Chalmers L, Van Horn A, Sloan AE. Early necrosis following concurrent Temodar and radiotherapy in patients with glioblastoma. J. Neurooncol. 82(1 ), 81–83 (2007).
- Chakravarti A, Erkkinen MG, Nestler U et al. Temozolomide-mediated radiation enhancement in glioblastoma: a report on underlying mechanisms. Clin. Cancer Res. 12(15 ), 4738–4746 (2006).
- Taal W, Brandsma D, de Bruin HG et al. Incidence of early pseudo-progression in a cohort of malignant glioma patients treated with chemoirradiation with temozolomide. Cancer 113(2 ), 405–410 (2008).
- Brandes AA, Tosoni A, Spagnolli F et al. Disease progression or pseudoprogression after concomitant radiochemotherapy treatment: pitfalls in neurooncology. Neuro-oncology 10(3 ), 361–367 (2008).
- Chaskis C, Neyns B, Michotte A, De Ridder M, Everaert H. Pseudoprogression after radiotherapy with concurrent temozolomide for high-grade glioma: clinical observations and working recommendations. Surg. Neurol. 72(4 ), 423–428 (2009).
- Clarke JL, Iwamoto FM, Sul J et al. Randomized phase II trial of chemoradiotherapy followed by either dose-dense or metronomic temozolomide for newly diagnosed glioblastoma. J. Clin. Oncol. 27(23 ), 3861–3867 (2009).
- Fabi A, Russillo M, Metro G, Vidiri A, Di Giovanni S, Cognetti F. Pseudoprogression and MGMT status in glioblastoma patients: implications in clinical practice. Anticancer Res. 29(7 ), 2607–2610 (2009).
- Gerstner ER, McNamara MB, Norden AD, Lafrankie D, Wen PY. Effect of adding temozolomide to radiation therapy on the incidence of pseudo-progression. J. Neurooncol. 94(1 ), 97–101 (2009).
- Gunjur A, Lau E, Taouk Y, Ryan G. Early post-treatment pseudo-progression amongst glioblastoma multiforme patients treated with radiotherapy and temozolomide: a retrospective analysis. J. Med. Imaging Radiat. Oncol. 55(6 ), 603–610 (2011).
- Kang HC, Kim CY, Han JH et al. Pseudoprogression in patients with malignant gliomas treated with concurrent temozolomide and radiotherapy: potential role of p53. J. Neurooncol. 102(1 ), 157–162 (2011).
- Kong DS, Kim ST, Kim EH et al. Diagnostic dilemma of pseudoprogression in the treatment of newly diagnosed glioblastomas: the role of assessing relative cerebral blood flow volume and oxygen-6-methylguanine-DNA methyltransferase promoter methylation status. AJNR. Am. J. Neuroradiol. 32(2 ), 382–387 (2011).
- Park CK, Kim J, Yim SY et al. Usefulness of MS-MLPA for detection of MGMT promoter methylation in the evaluation of pseudoprogression in glioblastoma patients. Neuro-oncology 13(2 ), 195–202 (2011).
- Pouleau HB, Sadeghi N, Balériaux D, Mélot C, De Witte O, Lefranc F. High levels of cellular proliferation predict pseudoprogression in glioblastoma patients. Int. J. Oncol. 40(4 ), 923–928 (2012).
- Roldán GB, Scott JN, McIntyre JB et al. Population-based study of pseudoprogression after chemoradiotherapy in GBM. Can. J. Neurol. Sci. 36(5 ), 617–622 (2009).
- Sanghera P, Perry J, Sahgal A et al. Pseudoprogression following chemoradiotherapy for glioblastoma multiforme. Can. J. Neurol. Sci. 37(1 ), 36–42 (2010).
- Tsien C, Galbán CJ, Chenevert TL et al. Parametric response map as an imaging biomarker to distinguish progression from pseudoprogression in high-grade glioma. J. Clin. Oncol. 28(13 ), 2293–2299 (2010).
- Yaman E, Buyukberber S, Benekli M et al. Radiation induced early necrosis in patients with malignant gliomas receiving temozolomide. Clin. Neurol. Neurosurg. 112(8 ), 662–667 (2010).
- Young RJ, Gupta A, Shah AD et al. Potential utility of conventional MRI signs in diagnosing pseudoprogression in glioblastoma. Neurology 76(22 ), 1918–1924 (2011).
- Brandes AA, Franceschi E, Tosoni A et al. MGMT promoter methylation status can predict the incidence and outcome of pseudoprogression after concomitant radiochemotherapy in newly diagnosed glioblastoma patients. J. Clin. Oncol. 26(13 ), 2192–2197 (2008).
- Hegi ME, Diserens AC, Gorlia T et al. MGMT gene silencing and benefit from temozolomide in glioblastoma. N. Engl. J. Med. 352(10 ), 997–1003 (2005).
- Mullins ME, Barest GD, Schaefer PW, Hochberg FH, Gonzalez RG, Lev MH. Radiation necrosis versus glioma recurrence: conventional MR imaging clues to diagnosis. AJNR. Am. J. Neuroradiol. 26(8 ), 1967–1972 (2005).
- Hein PA, Eskey CJ, Dunn JF, Hug EB. Diffusion-weighted imaging in the follow-up of treated high-grade gliomas: tumor recurrence versus radiation injury. AJNR. Am. J. Neuroradiol. 25(2 ), 201–209 (2004).
- Asao C, Korogi Y, Kitajima M et al. Diffusion-weighted imaging of radiation-induced brain injury for differentiation from tumor recurrence. AJNR. Am. J. Neuroradiol. 26(6 ), 1455–1460 (2005).
- Tung GA, Evangelista P, Rogg JM, Duncan JA 3rd. Diffusion-weighted MR imaging of rim-enhancing brain masses: is markedly decreased water diffusion specific for brain abscess? AJR. Am. J. Roentgenol. 177(3 ), 709–712 (2001).
- Al Sayyari A, Buckley R, McHenery C, Pannek K, Coulthard A, Rose S. Distinguishing recurrent primary brain tumor from radiation injury: a preliminary study using a susceptibility-weighted MR imaging-guided apparent diffusion coefficient analysis strategy. AJNR. Am. J. Neuroradiol. 31(6 ), 1049–1054 (2010).
- Hu LS, Baxter LC, Smith KA et al. Relative cerebral blood volume values to differentiate high-grade glioma recurrence from posttreatment radiation effect: direct correlation between image-guided tissue histopathology and localized dynamic susceptibility-weighted contrast-enhanced perfusion MR imaging measurements. AJNR. Am. J. Neuroradiol. 30(3 ), 552–558 (2009).
- Gahramanov S, Raslan AM, Muldoon LL et al. Potential for differentiation of pseudoprogression from true tumor progression with dynamic susceptibility-weighted contrast-enhanced magnetic resonance imaging using ferumoxytol vs. gadoteridol: a pilot study. Int. J. Radiat. Oncol. Biol. Phys. 79(2 ), 514–523 (2011).
- Hu X, Wong KK, Young GS, Guo L, Wong ST. Support vector machine multiparametric MRI identification of pseudoprogression from tumor recurrence in patients with resected glioblastoma. J. Magn. Reson. Imaging 33(2 ), 296–305 (2011).
- Narang J, Jain R, Arbab AS et al. Differentiating treatment-induced necrosis from recurrent/progressive brain tumor using nonmodel-based semiquantitative indices derived from dynamic contrast-enhanced T1-weighted MR perfusion. Neuro-oncology 13(9 ), 1037–1046 (2011).
- Fatterpekar GM, Galheigo D, Narayana A, Johnson G, Knopp E. Treatment-related change versus tumor recurrence in high-grade gliomas: a diagnostic conundrum – use of dynamic susceptibility contrast-enhanced (DSC) perfusion MRI. AJR. Am. J. Roentgenol. 198(1 ), 19–26 (2012).
- Kim YH, Oh SW, Lim YJ et al. Differentiating radiation necrosis from tumor recurrence in high-grade gliomas: assessing the efficacy of 18F-FDG PET, 11C-methionine PET and perfusion MRI. Clin. Neurol. Neurosurg. 112(9 ), 758–765 (2010).
- Gahramanov S, Muldoon LL, Varallyay CG et al. Pseudoprogression of glioblastoma after chemo- and radiation therapy: diagnosis by using dynamic susceptibility-weighted contrast-enhanced perfusion MR imaging with ferumoxytol versus gadoteridol and correlation with survival. Radiology 266(3), 842–852 (2012).
- Graber JJ, Young RJ, Gupta A et al. Magnetic resonance (MR) perfusion imaging to differentiate early progression from pseudoprogression following chemoradiotherapy for glioblastoma (GBM). J. Clin. Oncol. 29 , Abstract 2009 (2011).
- Gahramanov S, Muldoon LL, Li X, Neuwelt EA. Improved perfusion MR imaging assessment of intracerebral tumor blood volume and antiangiogenic therapy efficacy in a rat model with ferumoxytol. Radiology 261(3 ), 796–804 (2011).
- Galbán CJ, Chenevert TL, Meyer CR et al. The parametric response map is an imaging biomarker for early cancer treatment outcome. Nat. Med. 15(5 ), 572–576 (2009).
- Schlemmer HP, Bachert P, Herfarth KK, Zuna I, Debus J, van Kaick G. Proton MR spectroscopic evaluation of suspicious brain lesions after stereotactic radiotherapy. AJNR. Am. J. Neuroradiol. 22(7 ), 1316–1324 (2001).
- Rock JP, Hearshen D, Scarpace L et al. Correlations between magnetic resonance spectroscopy and image-guided histopathology, with special attention to radiation necrosis. Neurosurgery 51(4 ), 912–919; discussion 919 (2002).
- Rock JP, Scarpace L, Hearshen D et al. Associations among magnetic resonance spectroscopy, apparent diffusion coefficients, and image-guided histopathology with special attention to radiation necrosis. Neurosurgery 54(5 ), 1111–1117; discussion 1117 (2004).
- Weybright P, Sundgren PC, Maly P et al. Differentiation between brain tumor recurrence and radiation injury using MR spectroscopy. AJR. Am. J. Roentgenol. 185(6 ), 1471–1476 (2005).
- Zeng QS, Li CF, Zhang K, Liu H, Kang XS, Zhen JH. Multivoxel 3D proton MR spectroscopy in the distinction of recurrent glioma from radiation injury. J. Neurooncol. 84(1 ), 63–69 (2007).
- Bobek-Billewicz B, Stasik-Pres G, Majchrzak H, Zarudzki L. Differentiation between brain tumor recurrence and radiation injury using perfusion, diffusion-weighted imaging and MR spectroscopy. Folia Neuropathol. 48(2 ), 81–92 (2010).
- Zeng QS, Li CF, Liu H, Zhen JH, Feng DC. Distinction between recurrent glioma and radiation injury using magnetic resonance spectroscopy in combination with diffusion-weighted imaging. Int. J. Radiat. Oncol. Biol. Phys. 68(1 ), 151–158 (2007).
- Matsusue E, Fink JR, Rockhill JK, Ogawa T, Maravilla KR. Distinction between glioma progression and post-radiation change by combined physiologic MR imaging. Neuroradiology 52(4 ), 297–306 (2010).
- Ricci PE, Karis JP, Heiserman JE, Fram EK, Bice AN, Drayer BP. Differentiating recurrent tumor from radiation necrosis: time for re-evaluation of positron emission tomography? AJNR. Am. J. Neuroradiol. 19(3 ), 407–413 (1998).
- Santra A, Kumar R, Sharma P et al. F-18 FDG PET-CT in patients with recurrent glioma: comparison with contrast enhanced MRI. Eur. J. Radiol. 81(3 ), 508–513 (2012).
- Chen W. Clinical applications of PET in brain tumors. J. Nucl. Med. 48(9 ), 1468–1481 (2007).
- Chen W, Cloughesy T, Kamdar N et al. Imaging proliferation in brain tumors with 18F-FLT PET: comparison with 18F-FDG. J. Nucl. Med. 46(6 ), 945–952 (2005).
- Wardak M, Schiepers C, Dahlbom M et al. Discriminant analysis of 18F-fluorothymidine kinetic parameters to predict survival in patients with recurrent high-grade glioma. Clin. Cancer Res. 17(20 ), 6553–6562 (2011).
- Xiangsong Z, Weian C. Differentiation of recurrent astrocytoma from radiation necrosis: a pilot study with 11N-NH3 PET. J. Neurooncol. 82(3 ), 305–311 (2007).
- Schober O, Duden C, Meyer GJ, Müller JA, Hundeshagen H. Non selective transport of [11C-methyl]-l-and d-methionine into a malignant glioma. Eur. J. Nucl. Med. 13(2 ), 103–105 (1987).
- Tsuyuguchi N, Takami T, Sunada I et al. Methionine positron emission tomography for differentiation of recurrent brain tumor and radiation necrosis after stereotactic radiosurgery – in malignant glioma. Ann. Nucl. Med. 18(4 ), 291–296 (2004).
- Terakawa Y, Tsuyuguchi N, Iwai Y et al. Diagnostic accuracy of 11C-methionine PET for differentiation of recurrent brain tumors from radiation necrosis after radiotherapy. J. Nucl. Med. 49(5 ), 694–699 (2008).
- Tripathi M, Sharma R, Varshney R et al. Comparison of F-18 FDG and C-11 methionine PET/CT for the evaluation of recurrent primary brain tumors. Clin. Nucl. Med. 37(2 ), 158–163 (2012).
- Rachinger W, Goetz C, Pöpperl G et al. Positron emission tomography with O-(2-[18F]fluoroethyl)-l-tyrosine versus magnetic resonance imaging in the diagnosis of recurrent gliomas. Neurosurgery 57(3 ), 505–511; discussion 505 (2005).
- Mehrkens JH, Pöpperl G, Rachinger W et al. The positive predictive value of O-(2-[18F]fluoroethyl)-l-tyrosine (FET) PET in the diagnosis of a glioma recurrence after multimodal treatment. J. Neurooncol. 88(1 ), 27–35 (2008).
- Chen W, Silverman DH, Delaloye S et al. 18F-FDOPA PET imaging of brain tumors: comparison study with 18F-FDG PET and evaluation of diagnostic accuracy. J. Nucl. Med. 47(6 ), 904–911 (2006).
- Ledezma CJ, Chen W, Sai V et al. 18F-FDOPA PET/MRI fusion in patients with primary/recurrent gliomas: initial experience. Eur. J. Radiol. 71(2 ), 242–248 (2009).
- Brandsma D, Stalpers L, Taal W, Sminia P, van den Bent MJ. Clinical features, mechanisms, and management of pseudoprogression in malignant gliomas. Lancet Oncol. 9(5 ), 453–461 (2008).
- Giglio P, Gilbert MR. Cerebral radiation necrosis. Neurologist 9(4 ), 180–188 (2003).
- Kumar AJ, Leeds NE, Fuller GN et al. Malignant gliomas: MR imaging spectrum of radiation therapy- and chemotherapy-induced necrosis of the brain after treatment. Radiology 217(2 ), 377–384 (2000).
- Rusthoven KE, Olsen C, Franklin W et al. Favorable prognosis in patients with high-grade glioma with radiation necrosis: the University of Colorado reoperation series. Int. J. Radiat. Oncol. Biol. Phys. 81(1 ), 211–217 (2011).
- Marks JE, Baglan RJ, Prassad SC, Blank WF. Cerebral radionecrosis: incidence and risk in relation to dose, time, fractionation and volume. Int. J. Radiat. Oncol. Biol. Phys. 7(2 ), 243–252 (1981).
- Floyd NS, Woo SY, Teh BS et al. Hypofractionated intensity-modulated radiotherapy for primary glioblastoma multiforme. Int. J. Radiat. Oncol. Biol. Phys. 58(3 ), 721–726 (2004).
- Ruben JD, Dally M, Bailey M, Smith R, McLean CA, Fedele P. Cerebral radiation necrosis: incidence, outcomes, and risk factors with emphasis on radiation parameters and chemotherapy. Int. J. Radiat. Oncol. Biol. Phys. 65(2 ), 499–508 (2006).
- DeAngelis LM. Brain tumors. N. Engl. J. Med. 344(2 ), 114–123 (2001).
- Shaw E, Arusell R, Scheithauer B et al. Prospective randomized trial of low- versus high-dose radiation therapy in adults with supratentorial low-grade glioma: initial report of a North Central Cancer Treatment Group/Radiation Therapy Oncology Group/Eastern Cooperative Oncology Group study. J. Clin. Oncol. 20(9 ), 2267–2276 (2002).
Pseudoprogression after glioma therapy: a comprehensive review
To obtain credit, you should first read the journal article. After reading the article, you should be able to answer the following, related, multiple-choice questions. To complete the questions (with a minimum 70% passing score) and earn continuing medical education (CME) credit, please go to www.medscape.org/journal/expertneurothera. Credit cannot be obtained for tests completed on paper, although you may use the worksheet below to keep a record of your answers. You must be a registered user on Medscape.org. If you are not registered on Medscape.org, please click on the New Users: Free Registration link on the left hand side of the website to register. Only one answer is correct for each question. Once you successfully answer all post-test questions you will be able to view and/or print your certificate. For questions regarding the content of this activity, contact the accredited provider, [email protected]. For technical assistance, contact [email protected]. American Medical Association's Physician's Recognition Award (AMA PRA) credits are accepted in the US as evidence of participation in CME activities. For further information on this award, please refer to http://www.ama-assn.org/ama/pub/category/2922.html. The AMA has determined that physicians not licensed in the US who participate in this CME activity are eligible for AMA PRA Category 1 Credits™. Through agreements that the AMA has made with agencies in some countries, AMA PRA credit may be acceptable as evidence of participation in CME activities. If you are not licensed in the US, please complete the questions online, print the AMA PRA CME credit certificate and present it to your national medical association for review.
Activity Evaluation: Where 1 is strongly disagree and 5 is strongly agree
1. You are seeing a 60-year-old man following treatment for glioblastoma multiforme with radiation therapy (RT) plus temozolomide. What should you consider regarding his overall prognosis and risk for radiation necrosis?
□ A The 2-year survival rate remains less than 10% with modern chemoradiotherapy (chemoRT)
□ B Most patients treated with temozolomide beyond 6 months have no evidence of disease progression
□ C Higher total dose of radiation and dose per fraction contribute to increased rates of radiation necrosis
□ D The mean interval to the diagnosis of radiation necrosis is approximately 2 months
2. The patient has a follow-up MRI of the head 3 months after completing his treatment. What is the approximate likelihood that he will have pseudoprogression (PsP)?
□ A 70%
□ B 50%
□ C 40%
□ D 20%
3. Which of the following factors is most important in predicting this patient’s risk of PsP?
□ A Tumor location in the temporal lobe
□ B Higher dose of radiation
□ C Nonmethylation of MGMT
□ D Emergence of a new neurologic symptom
4. The patient has a questionable finding on MRI. What should you consider regarding other imaging modalities to help identify possible early progressive disease (ePD)?
□ A Mean relative cerebral blood volume is higher in PsP than ePD on MR perfusion studies
□ B Cho/NAA and Cho/Cr ratios are higher in post-treatment radiation effects compared with ePD on proton MR spectroscopic imaging
□ C PET imaging with fluorodeoxyglucose (FDG) is ideal for follow-up of glioblastoma after chemoRT
□ D There are no reliable techniques to differentiate ePD from PsP on conventional MRI