ABSTRACT
Numerous paleolimnological studies of Arctic lakes and ponds have shown marked shifts in both algal and invertebrate taxa within the past ∼150 years that are consistent with recent climatic warming. However, the magnitude and timing of changes are often non-uniform, with large, deep lakes frequently exhibiting muted assemblage shifts relative to smaller ponds. The hypothesis that duration and extent of ice cover exerts an overriding influence on habitat availability for biota has been commonly invoked to explain these differences, and many studies indicate that changes in ice cover are important drivers of recent biological changes. However, a detailed paleolimnological comparison of two lakes from the same region that have similar water chemistry but different ice cover regimes has not yet been attempted. Here we examine the influence of prolonged ice cover on the rate, magnitude, and direction of fossil diatom species shifts over time in two remarkably similar and adjacent Ellesmere Island lakes that mainly differ in their periods of ice cover. These two lakes exhibit strikingly different paleolimnological diatom profiles, despite their physical proximity, similar depths, and nearly identical water chemistry. In the lake characterized by prolonged ice cover, we find little evidence of diatom-inferred environmental change over its recent history, while diatom assemblages have undergone dramatic changes in the lake with the shorter duration of ice cover. This study supports the general hypothesis that changes in ice cover are a principle determinant of shifting diatom assemblages in High Arctic lakes.
Introduction
High latitude regions are especially sensitive to climatic changes (CitationACIA, 2004) and therefore represent critical reference areas for studies of long-term environmental change. As long-term monitoring data are lacking with respect to both temporal and spatial scales, proxy indicators of environmental change must be relied upon to reconstruct environmental histories in this climatically sensitive area (CitationPienitz et al., 2004). The abundance of lakes and ponds in the Canadian High Arctic allows for regional assessments of environmental change using paleolimnological techniques. The accumulation of allochthonous and autochthonous biological, chemical, and physical indicators in lacustrine sediments provides a rich archive of information about past environments (CitationSmol, 2008). Diatoms are siliceous unicellular algae (class Bacillariophyceae) that are particularly useful environmental indicators because they are ubiquitous, respond rapidly to changing conditions, and different species often have distinct optima with respect to many important environmental variables (CitationStoermer and Smol, 1999). Diatoms have been especially effective biomonitors in Arctic regions (CitationDouglas and Smol, 1999; CitationDouglas et al., 2004; CitationSolovieva et al., 2005) where other sources of proxy data are sometimes lacking.
In a recent analysis of 55 paleolimnological profiles from circumpolar lakes and ponds, CitationSmol et al. (2005) summarized the often dramatic changes in biological microfossils, including diatoms, which have occurred over the last ∼150 years. Assemblage changes were found to be consistent with climatic warming, with the greatest changes observed in the northernmost regions. Interpretations based on diatoms are also consistent with studies using non-biological proxies (e.g. CitationSmith et al., 2004). Changes in lake ice cover were first proposed by CitationSmol (1983) as a major cause for some of the striking species changes, and this idea was developed further by CitationSmol (1988) and CitationDouglas and Smol (1999). Briefly, during cooler periods, the persistence of ice cover can reduce primary production by limiting light penetration into the lake. Under warmer conditions, ice cover is reduced, more light is available for photosynthesis, and as a result more habitat becomes available for colonization by algae. However, other factors, such as lake depth, also play a role in how changes in climate, manifested through changes in lake ice, could affect diatom flora. For example, in relatively deep, extensively ice-covered lakes near Alert and Resolute Bay (), diatom communities showed subtle shifts that began over the last few decades (CitationDoubleday et al., 1995; CitationMichelutti et al., 2003a; CitationAntoniades et al., 2005). In contrast, small, shallow ponds at Cape Herschel (Ellesmere Island) and Isachsen (Ellef Ringnes Island) () tracked relatively dramatic changes in diatoms beginning much earlier, in the late 19th or early 20th century, respectively (CitationDouglas et al., 1994; CitationAntoniades et al., 2005). Likewise, across the circumpolar north, many deeper lakes have recorded increases in planktonic species during recent history (CitationSorvari et al., 2002; CitationRühland et al., 2003), whereas shallow ponds have been characterized by shifts in taxa indicative of greater periphytic (aquatic plant) habitat availability (CitationSmol et al., 2005). Both the development of plankton (in deeper lakes) and periphyton (in shallow ponds), as well as the delayed impact of warming in some locations, could be explained by changes in the persistence of ice cover, and associated limnological changes in the physical, chemical, and biological characteristics of deeper versus shallower lakes (CitationSmol, 1988; CitationDouglas and Smol, 1999).
FIGURE 1 Map showing the locations of the study site (star) and the other sites (numbered) mentioned in the text: 1. Alert, 2. Hazen Plateau, 3. Agassiz Ice Cap, 4. Fosheim Peninsula, 5. Cape Herschel, 6. Isachsen, Ellef Ringnes Island, 7. Char Lake, Cornwallis Island.
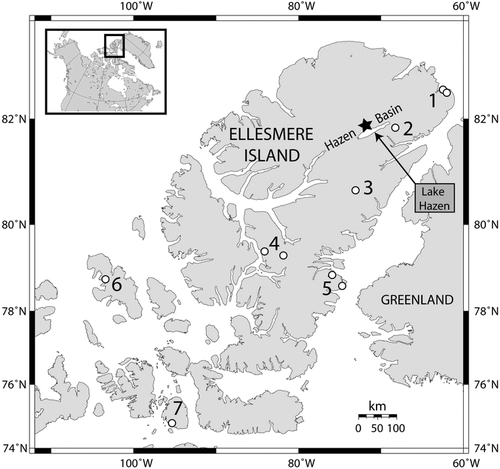
While a large diatom calibration set has been developed to infer ice cover duration in subarctic lakes in Fennoscandia (CitationThompson et al., 2005), the ice cover hypothesis has yet to be explicitly tested using a paleolimnological approach. In this paper, we test the hypothesis that ice cover is the overriding influence on diatom communities in High Arctic lakes by examining diatom assemblage shifts in two small, adjacent lakes, Skeleton Lake and Lake EP2, in northern Ellesmere Island, which are very similar in all limnological respects (e.g. location, morphology, and water chemistry variables that influence diatoms) other than duration of ice cover.
Although instrumental records of climate change do not exist for the study sites and the Lake Hazen region of northern Ellesmere Island, previous diatom-based paleolimnological investigations suggest that environmental changes have occurred at Alert (CitationDoubleday et al., 1995; CitationAntoniades et al., 2005), the Hazen Plateau (CitationSmith, 2002), and the Fosheim Peninsula (CitationWolfe, 2000; CitationPerren et al., 2003). Likewise, glacier mass balance records (CitationBraun et al., 2004), melt records from the nearby Agassiz Ice Cap (CitationKoerner and Fisher, 1990), and instrumental data from Alert and Eureka weather stations (CitationMeteorological Service of Canada, 2006) indicate a regional warming in recent decades. Thus, while environmental change has occurred in this region, ice cover continues to persist longer on one lake than the other likely due to local cooling caused by shading from a nearby hill. If climate modulated changes in the duration and extent of ice cover is truly the dominant factor driving diatom assemblage shifts in Arctic lakes, we predict the greatest ecological changes to occur in the lake with the longest open water period.
Site Description
The two study lakes, Skeleton Lake and EP2 (unofficial name), are small (maximum depths ∼4 and ∼3 m, respectively), relatively simple basin, High Arctic lakes (81°50′N, 71°28′W), located on the north side of Lake Hazen, Ellesmere Island, Nunavut, Canada. Skeleton Lake drains into EP2 (less than 20 m to the east) via Skeleton Creek, which in turn flows through a third pond (EP3) and then into Lake Hazen (). Skeleton Lake has repeatedly been shown to become ice free later than EP2 (; CitationOliver and Corbet, 1966; National Air Photo Archives; and field observations, this study, 2003). This is likely due to the greater shading and protection from winds on Skeleton Lake by Blister Hill, located to the south (; CitationOliver and Corbet, 1966).
FIGURE 3 Ice-off dates for Skeleton Lake (solid bars) and Lake EP2 (hatched bars). The data from 1963 and 1964 are from CitationOliver and Corbett (1966). The data from 2003 is approximated from our own field observations based on two site visits that occurred seven days apart; during the first visit, EP2 was already ice-free while Skeleton Lake was ∼80% ice-covered. Seven days later, after very warm weather, Skeleton Lake was ice-free.
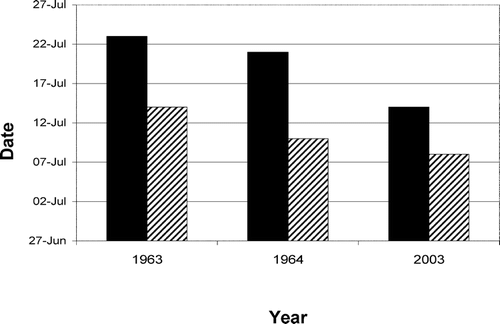
For its latitude, the Hazen Basin region experiences anomalously warm summer conditions due to its continental location and its placement on the leeward side of the Grant Land Mountains (CitationThompson, 1994). In July 2003, for example, mean maximum daily temperatures at Eureka and Alert were 10.9 and 4.3 °C, respectively (CitationMeteorological Service of Canada, 2006), while the mean maximum daily temperature at Lake Hazen camp was 16 °C. The area receives very little precipitation (∼95 mm annually; CitationThompson, 1994). The frost-free period at Lake Hazen () lasts 8–10 weeks and, as such, supports a greater abundance and diversity of vegetation than the surrounding areas (CitationSoper and Powell, 1985). Skeleton Lake, EP2, and EP3 are surrounded by wet meadows which are predominantly covered with Carex aquatilis var. stans and Eriophorum spp., as well as Saxifraga, Lychnis, Salix, Stellaria, Arctagrostis, Polygonum, Equisetum, and many types of mosses (CitationSoper and Powell, 1985). Bedrock consists of Jurassic and Cretaceous sandstone and shale; this is overlain by glacial till, sand, gravel, talus, and soils of Pleistocene and more recent origin (CitationChristie, 1964).
Methods
Water chemistry measurements and water samples were taken from Skeleton Lake, EP2, and EP3 within approximately one hour of each other on 7 July 2003. Temperature, pH, and specific conductivity were measured in the field with a handheld thermometer, a handheld Hanna pHEP pH meter and aYSI model 33 conductivity meter, respectively. Water samples were also taken for total filtered phosphorus (TPf), total unfiltered phosphorus (TPu), nitrate-nitrite (NO3-NO2), ammonia (NH3), total Kjeldahl nitrogen (TKN), total dissolved nitrogen (TdN), particulate nitrogen (PON), dissolved organic carbon (DOC), dissolved inorganic carbon (DIC), particulate carbon (POC), dissolved silica (SiO2), chlorophyll a (Chl a, uncorrected for phaeophytin), calcium (Ca), magnesium (Mg), sodium (Na), potassium (K), sulfate (SO4), and chloride (Cl). Laboratory analyses were performed at the National Laboratory for Environmental Testing (NLET), Environment Canada, Burlington, Ontario, Canada, using methods outlined in CitationEnvironment Canada (1994).
Sediment cores were obtained using a modified CitationGlew (1989) corer (3.75 cm inner diameter) from the deepest part of both Skeleton Lake (33 cm long) and EP2 (17 cm long) on 14 July 2003. Cores were sectioned on-site at 0.5 cm intervals using a CitationGlew (1988) extruder. The uppermost (∼2 cm) sediments from the adjacent pond, EP3, were sampled by hand to allow for a comparison of the recently deposited diatom assemblages between the three sites.
Subsamples from each 0.5-cm-thick interval of both cores were analyzed for 210Pb using alpha spectrometry at MyCore Scientific, Deep River, Ontario, Canada. The 210Pb dates for Skeleton Lake were calculated using the constant rate of supply (CRS) method (CitationBinford, 1990). In the sediments from EP2, the 210Pb activity levels were too low to provide a reliable age model, so trace elements were used to identify anthropogenic pollution horizons as an approximate marker for the increase in industrial emissions at a.d. ca. 1850. Trace element analyses were carried out by Activation Laboratories Inc., in Ancaster, Ontario, Canada. A suite of metals (specifically total Pb) were measured using a Perkin Elmer SCIEZ ELAN 6100 Inductively Coupled Plasma Mass Spectrometer (ICP/MS). International certified reference materials USGS GXR-1, GXR-2, GXR-4, and GXR-6 were analyzed at the beginning and end of each batch of samples. Internal control standards were analyzed every 10 samples and a duplicate was run for every 10 samples. Total Hg was measured on a Perkin Elmer FIMS 100 cold vapor Hg analyzer. Organic matter was estimated by loss on ignition (LOI) at 550 °C for 2 hours (CitationHeiri et al., 2001) at PEARL in Kingston, Ontario, Canada.
Microwave digestion techniques were used to clean diatom frustules from the sediment with concentrated HNO3 (CitationParr et al., 2004). Diatom slurries were rinsed with deionized water until they reached a neutral pH, dried on pre-cleaned glass cover slips, and mounted on slides with Naphrax® (high refractive index mounting medium). A minimum of 500 diatom valves was identified and enumerated from each sample using a Leica DMRB microscope. Taxonomy primarily followed CitationKrammer and Lange-Bertalot (1986–1991), CitationKrammer (2002), and CitationAntoniades et al. (2008). In order to examine whether rare species were tracking environmental changes in these lakes, we used all species in a principal components analysis (PCA). We also performed a detrended correspondence analysis (DCA) to examine species turnover in standard deviation (SD) units (CitationLepš and Šmilauer, 2003). This ecologically relevant interpretation provides an objective comparison of species compositional change between the two sites.
Results
Modern Limnology
The physical and chemical variables measured for Skeleton Lake, EP2, and EP3 are shown in . The variables of greatest limnological interest that influence diatom assemblages, including pH, specific conductivity, and dissolved nutrients, are nearly identical between the three lakes; notable exceptions to this include temperature and the particulate fractions of carbon (POC) and nitrogen (PON), and NH3. However, when examined in the context of 55 lakes and ponds from northern Ellesmere Island, a principal components analysis (PCA), based on water chemistry variables that are expected to influence diatom assemblages, indicates that they are more similar to each other than to 52 other sites from northern Ellesmere Island ().
TABLE 1 Selected limnological characteristics for the three study lakes.
FIGURE 4 Principal components analysis (PCA) biplot based on selected measured water chemistry variables that are considered to influence diatom assemblages (pH, specific conductivity, dissolved nutrients, and related variables). The arrows represent the measured environmental variables, while the open circles represent 52 lakes and ponds across northern Ellesmere Island. Skeleton Lake is represented by the solid square, EP2 is represented by the open square, and EP3 is represented by the solid circle. The proximity of these three sites represents their highly similar water chemistry.
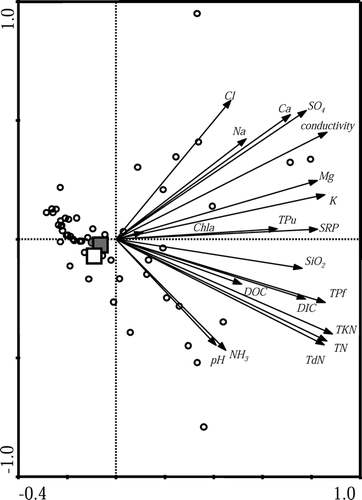
Diatom assemblages identified from the recently deposited sediments of the three lakes are listed in . All three lakes are characterized by small, benthic diatoms and share high abundances of some common taxa (e.g. small, benthic Fragilaria spp. sensu lato). The diatom assemblage in the surface sediments from EP3 was the most diverse of the three lakes, and consisted of small Achnanthes spp. (A. petersenii, A. minutissima), Brachysira zellensis, Cymbella spp., Denticula spp., and small Nitzschia species (). Similar to EP2, the most prevalent diatom in EP3 was Fragilaria brevistriata suggesting that some as-of-yet unidentified characteristic about these water bodies currently favors the growth of F. brevistriata.
TABLE 2 Diatom taxa (>3% relative abundance) from the top 2.5 cm intervals from the sediment cores of Skeleton Lake and EP2 and the surface sediment (∼2 cm) diatoms from EP3.
Paleolimnology
Unsupported 210Pb concentrations in the Skeleton Lake sediment core displayed a largely exponential decline until reaching background levels at 4.75 cm depth, corresponding to an age of a.d. ca. 1877 (). Since the 210Pb concentrations were too low in the EP2 sediment core to obtain reliable dates, we examined total Pb and Hg concentrations to provide an approximate time horizon indicating the onset of airborne anthropogenic pollution. Sedimentary concentrations of Pb and Hg in ponds from nearby Greenland have been shown to increase starting in the early 19th and 20th centuries (CitationBindler et al., 2001a, Citation2001b). Thus, a rise in the Hg and Pb concentrations at 9 cm depth is interpreted as corresponding to ∼150 to 200 years BP.
FIGURE 5 (A) Age-depth model for Skeleton Lake based on CRS model (CitationBinford, 1990). (B) Total 210Pb activities. The dashed line indicates estimated supported 210Pb.
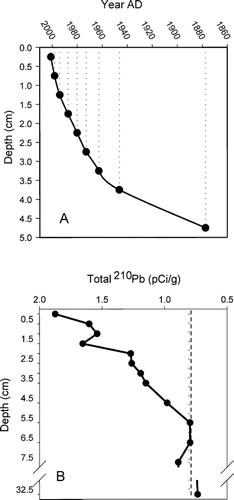
A list of common diatom taxa and the DCA axis 1 gradient lengths (an estimate of change in species composition) of the sediment cores of Skeleton Lake and EP2 can be found in . There is little change in the 33 cm Skeleton Lake core (); this is reflected in the short gradient length of DCA axis 1 (1.06 SD units; ). Diatom assemblages are composed of small, benthic taxa, dominated by Fragilaria construens var. venter (∼80–90%). Throughout much of this core, F. construens var. venter co-occurs with F. pinnata (up to ∼20%). In the top 2 cm (corresponding to approximately the last ∼30 years), there is a small increase in F. brevistriata (up to its maximum relative abundance of ∼10%), as well as the near complete disappearance of F. pinnata.
TABLE 3 Common diatom taxa (>3% relative abundance) found in the sediment cores from Skeleton Lake and EP2. Numbers in parentheses after the lake name represent the lengths of the DCA axis 1 gradients, in standard deviation units; N is the number of occurrences, N2 is the effective number of occurrences, calculated according to Hill's N2 (CitationHill, 1973); Max % is the maximum relative abundance. Synonyms for taxa with revised names are listed in the far right column.
FIGURE 6 (A) Diatom profile of Skeleton Lake showing taxa present in at least one interval with a relative abundance of >3%. See for synonyms for some of the common taxa. Percent loss-on-ignition (%LOI; an estimate of organic matter) and PCA axis 2 sample scores (PCA2; a summary of change occurring in rare taxa) are presented at the right side of the profile. (B) Photographs of ice cover on Skeleton Lake and Lake EP2, indicating the physical proximity of the two lakes, Blister Hill, and a nearby pingo for reference (photographs taken 7 July 2003). (C) Diatom profile of EP2 showing only species that are present in at least 3% relative abundance in at least one interval. See for synonyms for some of the common taxa. Percent loss-on-ignition (% LOI), total Pb, and total Hg (both expressed per gram organic carbon), and PCA axis two sample scores (PCA2) are presented at the right side of the profile. The rise in total Pb and Hg are interpreted to mark the onset of anthropogenic pollution (mid-19th to early 20th century).
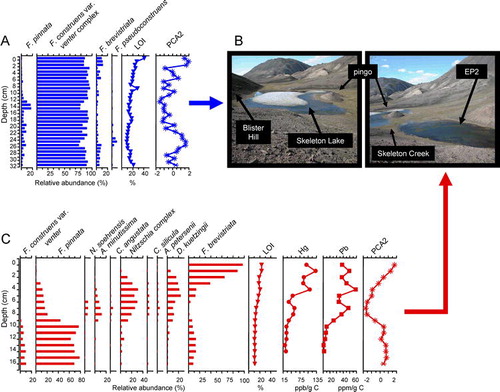
In nearby Lake EP2, which is less shaded, warmer, and experiences less prolonged ice cover than Skeleton Lake (), three periods of diatom change occur within the 17 cm sediment core (). These patterns in the dominant species are also reflected when all species are incorporated in the DCA, which indicates a species turnover of 2.00 SD units (). Below 10 cm, F. pinnata dominated the record (up to ∼80% relative abundance), and was accompanied by other small benthic diatoms such as F. construens var. venter and F. brevistriata (). At ∼9.25 cm, estimated to be ∼100–150 years BP based on our geochemical proxies, diatom assemblages diversified to include taxa such as Denticula kuetzingii, small Nitzschia species (N. frustulum, N. inconspicua, and N. perminuta), and Cymbella spp. In the uppermost sediments, between 0 and 2 cm, a small benthic diatom, F. brevistriata, becomes the dominant taxon.
Discussion
Modern Limnology
The similarity of the modern limnological variables among the three lakes is a function of their similar bedrock, regional climate, and connectivity via Skeleton Creek. The notable exceptions to these similarities are temperature and the particulate fractions of carbon (POC) and nitrogen (PON), which are all higher in EP2 and EP3 than Skeleton Lake, and NH3, which is highest in EP2 (). The lower temperature of Skeleton Lake can be attributed to the persistent ice cover that was present at the time of sampling; this ice cover is in turn related to the local shading of Skeleton Lake. Both POC and PON are measures of particulate matter in the water column, and not directly indicative of nutrients that are available to photosynthetic organisms. Higher particulate concentrations in EP2 and EP3 suggest that they have higher concentrations of algal and detrital matter, which are indicative of higher production and/or inflow energy in these systems, as would be expected with decreased ice cover (CitationSmol, 1988). Moreover, as water flows from Skeleton Lake through EP2 and then EP3, it is reasonable to expect higher particulate matter downstream. Although NH3 concentrations are twice as high in EP2 as Skeleton Lake, both values are low compared to the range of NH3 values for 24 ponds and lakes located within ∼10 km radius of our study site (range NH3 = 0.003 to 0.145 mg L−1; CitationKeatley et al., 2007). In any case, as all three lakes are P limited, not N limited (), slight differences in these nutrients are not likely to be biologically significant.
Modern Diatom Assemblages
Although there are some specific taxonomic differences between the diatom assemblages present in the recently deposited sediments of Skeleton Lake, EP2, and EP3, they also exhibit some important similarities (). For example, small benthic Fragilaria sensu lato taxa are the most abundant diatoms in each of the three sites. Small Fragilaria taxa, especially F. pinnata, are considered to be pioneering diatoms that can commonly exploit harsh conditions and have been well documented as an indicator of cool conditions with short growing seasons in both the Arctic (CitationDouglas et al., 1994; CitationMichelutti et al., 2003a), and in alpine regions (CitationLotter and Bigler, 2000), and are frequently the dominant taxa found in pre-industrial polar lake sediments (CitationSmol et al., 2005). Ecological preferences that can distinguish these small Fragilaria taxa are difficult to define; for example, CitationKarst-Riddoch (2004) suggested that the distribution of small benthic Fragilaria taxa (F. pinnata, F. construens var. venter, F. brevistriata) in many lakes across Iceland was not related to measured environmental variables.
The differences among the three lakes can best be appreciated with respect to the higher diversity of taxa representing different growth forms and life strategies. In Lake EP3, which experiences the least shading and is the shallowest lake, the diatom assemblage consists of many species known to have secondary growth characteristics (e.g. Cymbella taxa often have mucilaginous stalks). Such diverse assemblages have been linked to regions with longer growing seasons, as these more complex life forms would take longer to develop (CitationDouglas and Smol, 1999).
Paleolimnology
Skeleton Lake and EP2 have very similar morphologies, water chemistry, are located within ∼20 m of each other, and are connected by Skeleton Creek, yet the historical composition of diatom species assemblages is strikingly different between the two lakes. Although both lakes must have experienced warming to some degree, Skeleton Lake has had more persistent ice cover than EP2. Thus, it was expected that diatom assemblage shifts consistent with climate warming would be recorded in EP2, but that only a muted diatom response to the same environmental drivers would be apparent in Skeleton Lake due to shading that leads to a cooler microclimate and extended ice cover (CitationSmol, 1988).
In Skeleton Lake, the consistent domination of the diatom assemblages by small, benthic Fragilaria taxa is likely indicative of cool conditions characterized by extensive ice cover (e.g. CitationSmol, 1988; CitationDouglas and Smol, 1999; CitationLotter and Bigler, 2000). In the most recent sediments, the subtle increase in F. brevistriata is similar to that found in the very recent sediments, representing the warmest years on record, of Char Lake, a large, mostly ice-covered High Arctic lake on Cornwallis Island (CitationMichelutti et al., 2003a). Thus, Skeleton Lake sediments indicate a relatively muted response to recent regional warming, consistent with its more extended ice cover.
In Lake EP2, on the other hand, shifts in diatom assemblages from those indicative of cool, harsh conditions towards a diversification of taxa indicative of more diverse substrate availability (e.g. moss epiphytes such as Denticula kuetzingii; CitationLim et al., 2001; CitationMichelutti et al., 2003b), and larger, more complex frustules (e.g. Cymbella taxa) are consistent with climate warming and reduced ice cover. Such cover duration would have led to a longer growing season for aquatic macrophytes and algae, as well as enhanced nutrient export from the catchment (CitationSmol, 1988; CitationDouglas and Smol, 1999). These types of diatom species changes are consistent with many other diatom changes seen in lakes and ponds throughout the Arctic (e.g. CitationDouglas et al., 1994; CitationMichelutti et al., 2003a; CitationAntoniades et al., 2005; CitationSmol et al., 2005).
Alternative Hypotheses
Other factors, such as acid precipitation, atmospheric deposition of anthropogenic nitrogen or other nutrients or pollutants, and/or some other unmeasured phenomenon, may potentially be proposed as alternate drivers explaining the diatom assemblage changes in Skeleton Lake and EP2. A comparison of the diatom profiles between the two lakes addresses these other factors.
The remote location of our study sites, far from point sources of pollution of any type, identifies atmospheric deposition as the major pathway of pollutant transport to these sites. Due to the proximity of our sites, both lakes are subjected to identical types and quantities of atmospheric deposition. Nonetheless, if some type of atmospheric deposition was affecting the lakes differentially, we should see differences in our water chemistry data, especially with respect to pH, dissolved nitrogen, and sulfate. However, both Skeleton Lake and EP2 are presently nearly identical with respect to these variables (), with the exceptions of POC, PON, and NH3, which have been discussed above. Furthermore, historical water chemistry measurements indicate that Skeleton Lake and EP2 have remained circumneutral to alkaline and, if anything, have slightly increased in pH since 1964 (CitationOliver and Corbet, 1966). These historical data also confirm that the water chemistry of Skeleton Lake and EP2 were very similar in 1964 (CitationOliver and Corbet, 1966), just as it was during our field work in 2003.
Enrichment from atmospheric nitrogen has been linked to diatom community shifts in lakes in the Rocky Mountains, U.S.A. (e.g. CitationWolfe et al., 2001; CitationSaros et al., 2003). In Arctic lakes on Baffin Island, nitrogen deposition may have acted in concert with climate changes to cause diatom shifts after 1950, although diatom community change commenced in the mid-19th century (CitationWolfe et al., 2006). Although our extremely remote study sites must have received comparatively less atmospheric N input than those in the continental U.S.A., persistent ice on Skeleton Lake could lead to differences in the timing of N delivery to the two lakes, thereby eliciting unique ecological responses. However, the diatom changes in the very remote Lake EP2 began over the last ∼100–150 years, based on our approximate chronological estimate, while atmospheric N deposition in comparatively impacted lakes in the continental U.S.A. only become an ecologically important source of N after the mid- to late 20th century (e.g. CitationWolfe et al., 2001; CitationSaros et al., 2003). Thus, even if differences in timing of delivery of N deposition were affecting diatom communities, this could only explain much more recent changes, and in any case, would ultimately still be traced back to differences in ice cover regime.
With respect to other types of pollution, previous paleolimnological studies have shown that no changes in diatom assemblages have been recorded in lakes that have experienced point-source pollution from PCBs (CitationPaterson et al., 2003). Thus, differences in acidification, amount of nitrogen deposition, and pollution cannot explain the types of diatom responses recorded in the Skeleton Lake and EP2 cores.
The results from the sediment cores from Skeleton Lake and EP2, as well as the surface sediment diatoms from EP3, all suggest that ice cover plays an important role in dampening diatom community shifts to environmental change. This is likely due to the role of ice cover in restricting the growing season for both algae and their potential substrates (e.g. mosses and grasses). However, due to microclimatic differences, the ice cover continues to persist longer at Skeleton Lake than at EP2. Thus, the changes recorded in the diatom records may reflect the crossing of some type of ice cover threshold in EP2 that has not yet been reached in Skeleton Lake, as the climatic changes have not yet been great enough to reduce ice cover to the point at which dramatic diatom shifts are occurring (). Furthermore, these results underscore the importance of careful site selection and contextual interpretation in paleolimnological studies, as local conditions may greatly influence ecological responses to regional environmental change (CitationSmol et al., 2005).
FIGURE 7 Schematic diagrams illustrating possible diatom responses to changing ice cover conditions in the two study lakes. Although the length of the ice-free season has likely increased in both lakes, it has yet to reach a critical threshold in Skeleton Lake, resulting in a muted diatom response. In Lake EP2, reduction in ice cover has crossed this critical threshold and resulted in marked diatom changes.
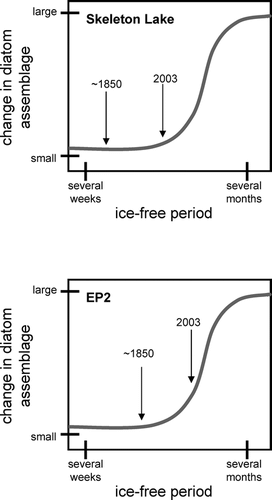
Conclusions
Although Skeleton Lake and EP2 are nearly identical with respect to water chemistry, morphology, and are located within ∼20 m of each other, the two lakes have recorded strikingly different diatom histories. The major difference between the two sites is the longer length of ice cover on Skeleton Lake relative to EP2. Both the muted diatom assemblage change in Skeleton Lake and the marked changes in EP2 suggest that ice cover plays an important role in influencing biological assemblages in polar lakes.
Acknowledgments
Funding for this project came from Natural Sciences and Engineering Research Council of Canada (NSERC) grants to Keatley, Douglas, and Smol, and from a Northern Scientific Training Program (NSTP) grant to Keatley. We would also like to thank the Polar Continental Shelf Project and Parks Canada for logistical support. We are grateful to the National Laboratory for Environmental Testing (NLET) at Environmental Canada for water chemistry analysis, S. Arnott and J. Glew for assistance in the field, and J. R. Glew for drafting the detailed inset map. Many thanks to D. Antoniades, N. Michelutti, and the anonymous journal reviewers for constructive comments on the manuscript, and to one of the journal reviewers for suggesting . This is PCSP contribution number 010-07.
References Cited
- ACIA 2004. Impacts of a warming Arctic. Arctic Climate Impact Assessment Cambridge Cambridge University Press. 140 pp.
- Antoniades, D. , M. S. V. Douglas , and J. P. Smol . 2005. Quantitative estimates of recent environmental changes in the Canadian High Arctic inferred from diatoms in lake and pond sediments. Journal of Paleolimnology 33:349–360.
- Antoniades, D. , P. B. Hamilton , M. S. V. Douglas , and J. P. Smol . 2008. Diatoms of North America: the freshwater floras of Prince Patrick, Ellef Ringnes and Northern Ellesmere Islands from the Canadian Arctic Archipelago. Iconographica Diatomologica 17:1–649.
- Bindler, R. , I. Renberg , N. J. Anderson , P. G. Appleby , O. Emteryd , and J. Boyle . 2001a. Pb isotope ratios of lake sediments in West Greenland: inferences on pollution sources. Atmospheric Environment 35:4675–4685.
- Bindler, R. , I. Renberg , P. G. Appleby , N. J. Anderson , and N. L. Rose . 2001b. Mercury accumulation rates and spatial patterns in lake sediments from West Greenland: A coast to ice margin transect. Environmental Science & Technology 35:1736–1741.
- Binford, M. W. 1990. Calculation and uncertainty analysis of 210Pb dates for PIRLA project lake sediment cores. Journal of Paleolimnology 3:253–267.
- Braun, C. , D. R. Hardy , and R. S. Bradley . 2004. Mass balance and area changes of four High Arctic plateau ice caps, 1959–2002. Geografiska Annaler Series A–Physical Geography 86A:43–52.
- Christie, R. L. 1964. Geological reconnaissance of northeastern Ellesmere Island, District of Franklin Ottawa: Geological Survey of Canada Memoir 331:. 79 pp.
- Doubleday, N. C. , M. S. V. Douglas , and J. P. Smol . 1995. Paleoenvironmental studies of black carbon deposition in the High Arctic—A case-study from northern Ellesmere Island. Science of the Total Environment 161:661–668.
- Douglas, M. S. V. and J. P. Smol . 1999. Freshwater diatoms as indicators of environmental change in the High Arctic. In Stoermer, E. F. and J. P. Smol , editors. The Diatoms: Applications for the Environmental and Earth Sciences Cambridge Cambridge University Press. 227–244.
- Douglas, M. S. V. , J. P. Smol , and W. Blake Jr . 1994. Marked post-18th century environmental change in high-arctic ecosystems. Science 266:416–419.
- Douglas, M. S. V. , P. B. Hamilton , R. Pienitz , and J. P. Smol . 2004. Algal indicators of environmental change in Arctic and Antarctic lakes and ponds. In Pienitz, R. , M. S. V. Douglas , and J. P. Smol , editors. Long-Term Environmental Change in Arctic and Antarctic Lakes Dordrecht Springer. 117–158.
- Environment Canada 1994. Manual of Analytical Methods Burlington, Canada National Laboratory for Environmental Testing, Canadian Centre for Inland Waters. 2 vols. 1097 pp.
- Glew, J. R. 1988. A portable extruding device for close interval sectioning of unconsolidated core samples. Journal of Paleolimnology 2:241–243.
- Glew, J. R. 1989. A new trigger mechanism for sediment samplers. Journal of Paleolimnology 2:241–243.
- Heiri, O. , A. F. Lotter , and G. Lemcke . 2001. Loss on ignition as a method for estimating organic and carbonate content in sediments: reproducibility and comparability of results. Journal of Paleolimnology 25:101–110.
- Hill, M. O. 1973. Diversity and evenness: a unifying notation and its consequences. Ecology 54:427–432.
- Karst-Riddoch, T. L. 2004. Sedimentary diatoms from sensitive alpine (northwest Canada) and subarctic (Iceland) lakes as indicators of environmental and climatic conditions Ph.D. thesis. Kingston, Canada Department of Biology, Queen's University. 194 pp.
- Keatley, B. E. , M. S. V. Douglas , and J. P. Smol . 2007. Limnological characteristics of a high Arctic oasis and comparisons across northern Ellesmere Island. Arctic 60:294–308.
- Koerner, R. M. and D. A. Fisher . 1990. A record of Holocene summer climate from a Canadian High-Arctic ice core. Nature 343:630–631.
- Krammer, K. 2002. Diatoms of Europe: Cymbella Ruggell A.R.G. Gantner Verlag. 584 pp.
- Krammer, K. and H. Lange-Bertalot . 1986–1991. Bacillariophyceae Stuttgart Gustav Fisher Verlag. 2485 pp.
- Lepš, J. and P. Šmilauer . 2003. Multivariate Analysis of Ecological Data using CANOCO Cambridge Cambridge University Press. 269 pp.
- Lim, D. S. S. , C. Kwan , and M. S. V. Douglas . 2001. Periphytic diatom assemblages from Bathurst Island, Nunavut, Canadian High Arctic: An examination of community relationships and habitat preferences. Journal of Phycology 37:379–392.
- Lotter, A. F. and C. Bigler . 2000. Do diatoms in the Swiss Alps reflect the length of ice-cover. Aquatic Sciences 62:125–141.
- Meteorological Service of Canada 2006. Monthly Climate Data Environment Canada (http://www.climate.weatheroffice.ec.gc.ca/climateData/monthlydata_e.html). Accessed July 2006.
- Michelutti, N. , M. S. V. Douglas , and J. P. Smol . 2003a. Diatom response to recent climatic change in a High Arctic lake (Char Lake, Cornwallis Island, Nunavut). Global and Planetary Change 38:257–271.
- Michelutti, N. , M. S. V. Douglas , and J. P. Smol . 2003b. Periphytic diatom assemblages from ultra-oligotrophic and UV transparent lakes and ponds on Victoria Island and comparisons with other diatom surveys in the Canadian Arctic. Journal of Phycology 39:465–480.
- Oliver, D. R. and P. S. Corbet . 1966. Operation Hazen: Aquatic Habitats in a High Arctic Locality: the Hazen Camp Study Area, Ellesmere Island, N.W.T Ottawa Department of National Defence. 126 pp.
- Parr, J. F. , K. H. Taffs , and C. M. Lane . 2004. A microwave digestion technique for the extraction of fossil diatoms from coastal lake and swamp sediments. Journal of Paleolimnology 31:383–390.
- Paterson, A. M. , A. A. Betts-Piper , J. P. Smol , and B. A. Zeeb . 2003. Diatom and chrysophyte algal response to long-term PCB contamination from a point-source in northern Labrador, Canada. Water Air and Soil Pollution 145:377–393.
- Perren, B. B. , R. S. Bradley , and P. Francus . 2003. Rapid lacustrine response to recent High Arctic warming: a diatom record from Sawtooth Lake, Ellesmere Island, Nunavut. Arctic, Antarctic, and Alpine Research 35:271–278.
- Pienitz, R. , M. S. V. Douglas , and J. P. Smol . 2004. Long-Term Environmental Change in Arctic and Antarctic Lakes Dordrecht Springer. 550 pp.
- Rühland, K. , A. Priesnitz , and J. P. Smol . 2003. Paleolimnological evidence from diatoms for recent environmental changes in 50 lakes across Canadian Arctic treeline. Arctic, Antarctic, and Alpine Research 35:110–123.
- Saros, J. E. , S. J. Interlandi , A. P. Wolfe , and D. R. Engstrom . 2003. Recent changes in the diatom community structure of lakes in the Beartooth Mountain Range, USA. Arctic, Antarctic, and Alpine Research 35:18–23.
- Smith, I. R. 2002. Diatom-based Holocene paleoenvironmental records from continental sites on northeastern Ellesmere Island, High Arctic, Canada. Journal of Paleolimnology 27:9–28.
- Smith Jr, S. V. , R. S. Bradley , and M. B. Abbott . 2004. A 300 year record of environmental change from Lake Tuborg, Ellesmere Island, Nunavut, Canada. Journal of Paleolimnology 32:137–148.
- Smol, J. P. 1983. Paleophycology of a High Arctic lake Cape Herschel, Ellesmere Island. Canadian Journal of Botany 61:2195–2204.
- Smol, J. P. 1988. Paleoclimate proxy data from freshwater Arctic diatoms. Verhandlungen Internationale Vereinigung für theoretische und angewandte Limnologie 23:837–844.
- Smol, J. P. 2008. Pollution of Lakes and Rivers: a Paleoenvironmental Perspective Second edition. Oxford Blackwell Publishing. 383 pp.
- Smol, J. P. , A. P. Wolfe , H. J. B. Birks , M. S. V. Douglas , V. J. Jones , A. Korhola , R. Pienitz , K. Ruhland , S. Sorvari , D. Antoniades , S. J. Brooks , M. A. Fallu , M. Hughes , B. E. Keatley , T. E. Laing , N. Michelutti , L. Nazarova , M. Nyman , A. M. Paterson , B. Perren , R. Quinlan , M. Rautio , E. Saulnier-Talbot , S. Siitoneni , N. Solovieva , and J. Weckstrom . 2005. Climate-driven regime shifts in the biological communities of arctic lakes. Proceedings of the National Academy of Sciences of the United States of America 102:4397–4402.
- Solovieva, N. , V. J. Jones , L. Nazarova , S. J. Brooks , H. J. B. Birks , J. A. Grytnes , P. G. Appleby , T. Kauppila , B. Kondratenok , I. Renberg , and V. Ponomarev . 2005. Palaeolimnological evidence for recent climatic change in lakes from the northern Urals, Arctic Russia. Journal of Paleolimnology 33:463–482.
- Soper, J. H. and J. M. Powell . 1985. Botanical Studies in the Lake Hazen Region, Northern Ellesmere Island, Northwest Territories, Canada Ottawa Publications in Natural Sciences, National Museums of Canada. 67 pp.
- Sorvari, S. , A. Korhola , and R. Thompson . 2002. Lake diatom response to recent Arctic warming in Finnish Lapland. Global Change Biology 8:171–181.
- Stoermer, E. F. and J. P. Smol . 1999. The Diatoms: Applications for the Environmental and Earth Sciences Cambridge Cambridge University Press. 469 pp.
- Thompson, R. , D. Price , N. Cameron , V. Jones , C. Bigler , P. Rosen , R. I. Hall , J. Catalan , J. Garcia , J. Weckstrom , and A. Korhola . 2005. Quantitative calibration of remote mountain-lake sediments as climatic recorders of air temperature and ice-cover duration. Arctic, Antarctic, and Alpine Research 37:626–635.
- Thompson, W. 1994. Climate. In . Resource Description and Analysis: Ellesmere Island, National Park Reserve Winnipeg National Resource Conservation Section, Prairie and Northern Region, Parks Canada, Department of Canadian Heritage. 78 pp.
- Wolfe, A. P. 2000. A 6500-year record from southwestern Fosheim Peninsula, Ellesmere Island, Nunavut. In Garneau, M. and B. T. Alt , editors. Environmental Response to Climate Change in the Canadian High Arctic Ottawa: Geological Survey of Canada, Bulletin 529,. 249–256.
- Wolfe, A. P. , J. S. Baron , and R. J. Cornett . 2001. Anthropogenic nitrogen deposition induces rapid ecological changes in alpine lakes of the Colorado Front Range (USA). Journal of Paleolimnology 25:1–7.
- Wolfe, A. P. , C. A. Cooke , and W. O. Hobbs . 2006. Are current rates of atmospheric nitrogen deposition influencing lakes in the eastern Canadian Arctic. Arctic, Antarctic, and Alpine Research 38:465–476.