Abstract
Monitoring studies show that many mountain glaciers worldwide are decreasing in mass. An important component of the process of ice mass loss is the effect of dust on albedo and its effect on glacier mass balance. The characteristics of surface dust were investigated in August 2006 on the Ürümqi Glacier No. 1 in the Tien Shan Mountains, China. The bare ice surface of the glacier was mostly covered by brown dust. The amounts of surface dust on the ice surface (dry weight) ranged from 86 to 1113 g m−2 (mean: 335 g m−2, standard deviation: = 211), which is within the normal range for Asian glaciers, but significantly greater than those on glaciers in other regions such as Alaska, Patagonia, and the Canadian Arctic. An analysis of organic matter and microscopy of the surface dust revealed that the dust contained high levels of organic matter, including living cyanobacteria. This suggests that it is comprised not only of deposits of wind-blown desert dust, but is also a product of microbial activity on the glacier itself. Spectral albedo of the glacial surface showed spectrum curves typical of those of snow and ice contaminated with dust. The integrated surface albedo ranged from 0.09 to 0.24 (mean: 0.14) in the ice area, from 0.50 to 0.64 (mean: 0.56) in the snow area. The lower albedo on the glacial surface compared with that of clean bare ice or snow surface suggests that the albedo was significantly reduced by the surface dust on this glacier. Results suggest that the mineral and organic dust on the glacial surface substantially accounts for the recent shrinkage of the glacier.
Introduction
Surface dust on glaciers can significantly affect albedo on the glacial surface (e.g. CitationBrock et al., 2000; CitationCutler and Munro, 1996). Surface materials usually consist of wind-blown dust particles, rock from the surrounding valley walls, glacial till, and/or organic matter produced by glacial organisms. These contaminants in snow and ice can reduce surface albedo and accelerate the melting of snow and ice. For example, the drastic decrease from 0.8 to 0.5 in albedo due to a strong dust storm was observed on the surface of a Tibetan glacier (CitationFujita, 2007). Its impact on glacial runoff has been estimated as a 29% increase over the control runoff without the dust deposition (CitationFujita, 2007). Recent observational records have shown a substantial thinning and terminus retreat of glaciers in many parts of the world (e.g. CitationMeier et al, 2007; CitationOerlemans, 2005). Glacial shrinkage is generally considered to result from climate change such as global warming, but also possibly from the variations in surface dust. Therefore, the characteristics and quantity of surface dust constitute one of the most important parameters needed to determine the mass balance of glaciers.
On Asian glaciers, wind-blown desert dust is one of major components of surface contamination. Significant amounts of wind-blown desert dust are deposited on glaciers in western China because the glaciers are surrounded by arid areas encompassing vast deserts such as the Taklimakan, Junggar, and Gobi (e.g. CitationWake et al., 1994). In this region, dust storms usually occur in spring and deposit enormous amounts of fine desert particles on the glacial surface. Such annual deposits form dust layers in the accumulation area, and can be visibly observed in ice cores or snow pits (e.g. CitationHan et al., 2006; CitationLi et al., 2006; CitationWake et al., 1994).
Recent studies have revealed that surface dust is derived not only from wind-blown desert dust, but also from biological activity on the glacial surface, where communities of snow algae, microfauna, insects, and bacteria thrive. These organisms are specialized taxa that have adapted to extremely cold environments (e.g. CitationHoham and Duval, 2001; CitationKohshima, 1987). Organic matter derived from such organisms forms small spherical granules on the glacial ice (e.g. CitationTakeuchi et al., 2001a). Such biogenic surface dust is known as cryoconite, and exerts a significant impact on the surface albedo of some glaciers (e.g. CitationTakeuchi et al., 2005; CitationTakeuchi et al., 2001a; CitationKohshima et al., 1993). However, the biological element of surface dust has not been thoroughly studied.
Ürümqi Glacier No. 1, located in the Tien Shan Mountains in northwest China, is a glacier in Asia that has been monitored for more than 40 years and is among those glaciers listed as actively receding by the World Glacier Monitoring Service (e.g. CitationYe et al., 2005). The reports have shown that this glacier is currently receding rapidly, so it is important to understand the process of glacial shrinkage. Although much research, including studies of mass balance, ice cores, and snow chemistry, has been carried out on the glacier (e.g. CitationLi et al., 2006; CitationLee et al., 2003; CitationWake et al., 1992), the characteristics of surface dust and effects of surface dust on the mass balance of this glacier have not yet been studied.
This paper aims to describe the quantity and characteristics of surface dust on the Ürümqi Glacier No. 1. Dust collected from various parts of the glacier in August 2006 was analyzed, and its characteristics were compared with those from other glaciers across the world. The spatial variations in surface albedo were measured on the glacier, and the effects of surface dust on the surface albedo are also discussed.
Study Site and Methods
The Tien Shan Mountains are one of the major mountain systems in central Asia with peaks rising about 4000–6000 m a.s.l. The Ürümqi Glacier No. 1 (43°06′N, 86°48′E) is located on the eastern side of the Tien Shan Mountains in the Xinjyang Uygur autonomous region of China. The total area of the glacier is approximately 1.73 km2. It lies between 3740 and 4486 m a.s.l., faces northwest, and consists of two branches (the east and west branches), which became separated in 1994 due to glacial shrinkage ( and ). Mass balance of the glacier has been monitored since 1959, and the resulting records have been published in annual reports of the Tianshan Glacier Station, as well as compiled by the World Glacier Monitoring Service of the International Commission on Snow and Ice. According to those records, the glacier has retreated continuously from 1962 to 2003, with the overall decrease during that period amounting to 20% of the glacier volume (CitationYe et al., 2005). The recent mean equilibrium line altitude was measured as 4110 m a.s.l. (1997–2003; CitationYe et al., 2005). Because the glacier is located in the headwaters of the Ürümqi River that flows into Ürümqi, which is the largest city in this region with a population of approximately 1.6 million, the glacial shrinkage is causing great concern over its impact on water resources (CitationYe et al., 2005).
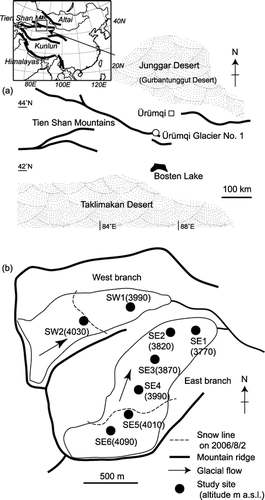
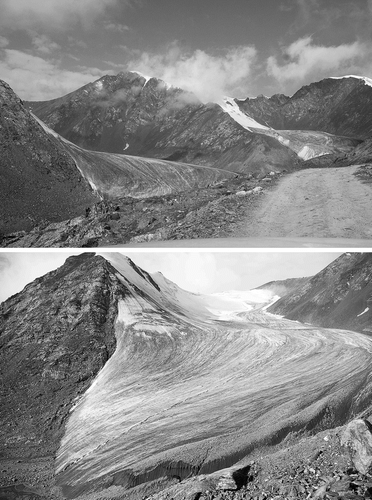
Field work was carried out from 2 to 5 August 2006. Sample collections and spectral albedo measurements were done at 6 sites on the east branch (SE1–SE6) and at 2 on the west branch (SW1 and SW2) (). The sites chosen were visibly representative of the surface conditions around each site in terms of their surface roughness and the amounts of rock debris. Moreover, these sites were selected because of their safety and easy accessibility. The snow line in our study period (early August) was approximately 4000 m a.s.l., and was located between sites SE4 and SE5 on the east branch, and SW1 and SW2 on the west branch ().
In order to quantify the amount of dust on the glacial surface and its organic-matter content, ice and snow on the surface layers were collected with a stainless-steel scoop (approximately 15 cm × 15 cm in area and 1–3 cm in depth) from 5 sites in the bare ice area (SE1–SE4, and SW1) and 3 in the snow area (SE5, SE6, and SW2). Five samples were collected from randomly selected surfaces at each study site. Collection areas on the surface were measured to calculate the amount of dust per unit area. To fix biological activity, the collected samples were melted and preserved as a 3% formalin solution in clean 30-mL polyethylene bottles. All samples were transported for analysis to a laboratory of Chiba University, Japan. The samples were dried (60°C, 24 hours) in pre-weighed crucibles. The amount of dust per unit area of the glacier was obtained based on measurements of the dry weight and the sampling area. The dried samples were then combusted for 3 hours at 500°C in an electric furnace, and weighed again. The amount of organic matter was calculated from the difference in weight between the dried and combusted samples. This method is slightly modified from CitationDean (1974). After combustion, only mineral particles remained. In order to investigate the composition of the surface dust, other samples of surface ice/snow were collected and examined with optical microscopes (Leica MZ125, and Nikon E600).
Spectral reflectances in the visible to near-infrared wavelength range (350–1050 nm) were measured on the glacial surface with a portable spectroradiometer (MS-720, Eiko Seiki, Japan). Measurements were taken using the sensor at a height of approximately 20 cm above the surface of the ice or snow with the instrument in the nadir-viewing position. Measurements made at this height provided a spot 8.9 cm in diameter on the snow or ice surface. The reflectances were obtained by dividing the surface radiances by the radiance acquired from a white reference panel that is nearly 100% reflective and diffuse. Measurements of the white panel were made immediately before and after each surface measurement. Spectral reflectances were measured at five surfaces randomly selected at each site. The mean of the five surface measurements at a given site constitutes the reflectance at that site.
Results
Amounts of Dust on the Glacier Surface
Most of the glacial surface of the ice area was covered with brown dust (). The amounts of dust on the bare ice surface ranged from 86 to 1113 g m−2 (mean: 335 g m−2, standard deviation [SD] = 211) in dry weight. The altitudinal profile of such amounts showed that those at the higher elevation sites (SE4 and SW1) were greater than those at the other sites except for the snow area (431–480 versus 238–267 g m−2; ); however, the difference was not statistically significant (one-way ANOVA test, F = 1.37, P = 0.288). Dust was also deposited at the bottom of cryoconite holes, relatively higher numbers of which were observed at site S3, in contrast to only a few at the other sites. Dust amounts in the snow area were much smaller than in the ice area, ranging from 4.0 to 9.8 g m−2 (mean: 6.2 g m−2, SD = 1.5).
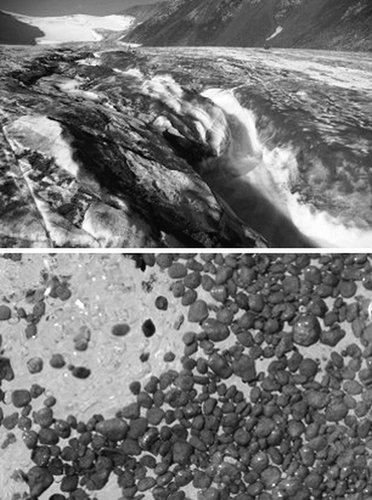
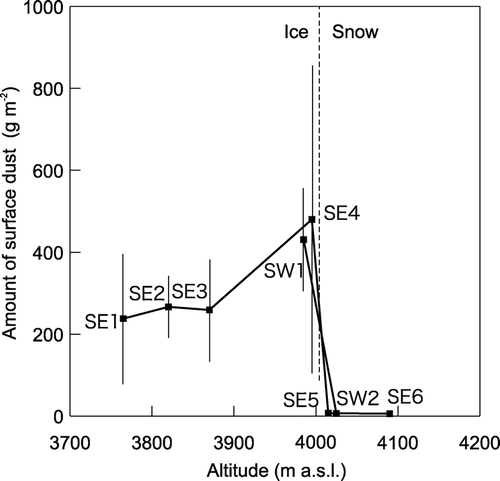
The surface dust in the ice area contained levels of organic matter ranging from 7.3 to 11.9% (mean: 9.4%, SD = 1.6) in dry weight (). The percentage of organic matter for specific sites was highest at site SE2 (11.5 ± 0.5%, mean ± SD), and gradually diminished down to site SE4 (8.0 ± 0.6%) as the elevation increased. The organic-matter content in the snow-surface dust was lower than that on the ice surface, ranging from 3.5 to 5.9% (mean: 5.1%, SD = 0.59).
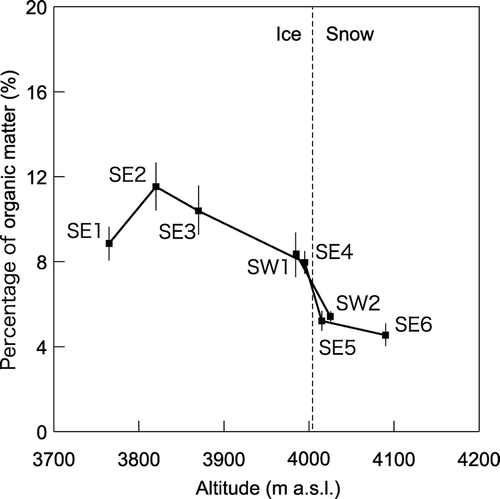
The amount of organic matter per unit area on the glacial surface ranged from 7.4 to 82.3 g m−2 (mean: 30.2 g m−2, SD = 15.6) on the ice surface, and from 0.18 to 0.42 (mean: 0.31 g m−2, SD = 0.07) on the snow surface (). The amount on the ice surface was relatively lower at the lowest site (20.5 ± 12.6 g m−2, SE1) and higher at sites SE4 and SW1 (37.8 ± 27.2 and 35.0 ± 8.1 g m−2, respectively).
Composition of Surface Dust
Microscopic study of the surface dust revealed mineral particles, amorphous organic matter, and living cyanobacteria. The proportion of the components, when observed by microscopy, clearly differed between the dust in snow and ice areas (). Brown organic granules were the main component in dust from the ice area (SE1–SE4, SW1), whereas mineral particles predominated in the snow area (SE5, SE6, and SW2) (). The brown granules are spherical and contain an abundance of filamentous cyanobacteria and mineral particles. The size of the organic granules ranged from 0.48 to 4.1 mm (mean: 1.4 mm, SD = 0.47). Observation with a fluorescence microscope revealed at least three taxa of filamentous cyanobacteria with autofluorescence densely covering the surface of the granules. The morphological characteristics of the three taxa were: (1) 2.9 ± 0.27 µm (mean ± SD) in cell diameter with a sheath, (2) 1.0 ± 0.12 µm in cell diameter with a sheath, and (3) 1.0 ± 0.11 µm in cell diameter without a sheath. The mineral particles in the dust were brown, white, or transparent, and were microscopically observed to range from 1.3 to 98 µm (mean: 15.2 µm, SD = 8.6) in diameter. The minerals appear to be quartz, feldspar, mica, calcite, and clay minerals, although more detailed studies are required. The dust on the snow surface mainly consisted of mineral particles, but also contained spherical unicellular green algae (Chlorophyta). The algal cells were red or green in color, and ranged 8.8 ± 3.5 µm (mean ± SD) in size. The algae were relatively more abundant at site SE5 than at site SE6.
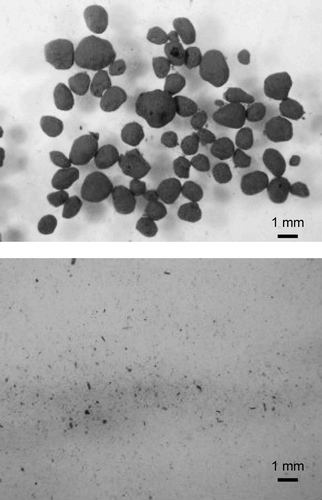
Spectral Reflectance of the Glacier Surface
Spectral reflectance on the glacial surface varied among the study sites (). The reflectance in the measured wavelength range (350–1050 nm) varied from 0.076 to 0.221 on the ice surface (SE1–SE4), and was significantly lower than on the snow surface, which varied from 0.341 to 0.661. In the ice surface spectra, the reflectance in the range between 550 and 850 nm was relatively higher than in other ranges. A small absorption (depression in the spectrum) was apparent at 680 nm in the ice-surface spectra. The snow-surface spectra showed that albedo increased as wavelength increased from 350 to 600 nm, held steady between 0.6 and 0.7 from 600 to 850 nm, and then decreased from 850 to 1050 nm.
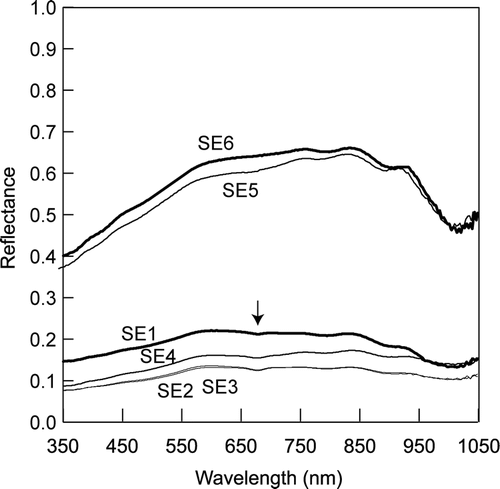
The integrated albedos in the measured wavelength range varied from 0.093 to 0.236 (mean: 0.143) in the ice area, and from 0.496 to 0.638 (mean: 0.560) in the snow area (). The albedo at the lowest site (SE1) was the highest in the ice area, while that at the middle site (SE3) was the lowest. In the snow area, the albedo at the lower site was lower than that at the higher site (0.531 at SE5 versus 0.588 at SE6).
Discussion
Our results indicate that surface dust significantly reduced the surface albedo of Ürümqi Glacier No. 1. In the ice area, the spectral reflectances measured on the surface showed that the reflectances in the visible wavelength range (350–800 nm) were generally low, but slightly higher in the range between 550 and 850 nm. They exhibit the typical spectral curves of a dirty glacial ice surface (e.g. CitationZeng et al., 1984). The higher reflectance between 550 and 850 nm corresponded to the optical characteristics of glacial surface dust on Chinese glaciers (CitationTakeuchi, 2002b). The mean integrated albedo of the ice surface on the glacier was 0.143, which is lower than that of a clean, bare ice surface, which is generally 0.34–0.51 (CitationPaterson, 1994). In western China, it has been reported to be 0.38 (0.34 to 0.42) on glaciers (CitationBai and Yu, 1985). The difference between the albedo of bare ice and the measured glacial albedo is 0.24, which equals the approximate effect of surface dust on this glacier.
The reduction in albedo by surface dust was also significant in the snow area. The spectral reflectance of clean snow or firn surface in the visible wavelength usually ranges from 0.60 to 0.95 and continuously diminishes as wavelength increases (e.g. CitationZeng et al., 1984). On the Ürümqi Glacier No. 1, the spectral albedos on two sites (SE5 and SE6) in the snow area showed that the albedo was generally lower than that of clean snow, and was particularly lower in the range between 350 and 550 nm. This curve indicates that the snow albedo was reduced by dust on the surface.
The composition of surface dust suggests that dust in the snow area mainly consisted of deposits of wind-blown mineral desert dust, while that in the ice area was mostly composed of cryoconite granules formed by microbial activity on the glacier. As previous studies have shown, significant amounts of desert dust can be deposited on the northern slopes of the Tien Shan Mountains from surrounding deserts such as the Junggar (CitationSun, 2002). Since microscopy confirmed that dust on the snow surface consisted mainly of fine mineral particles (), it appeared to be mostly wind-blown desert dust. On other glaciers, for example, some in Alaska and Altai, red colored snow algae account for the reduction in surface albedo in the snow area (e.g. Takeuchi, 2001d; CitationTakeuchi et al., 2006a, Citation2006b). Microscopy revealed red algal cells in the dust on the snow of Ürümqi Glacier No. 1. These are probably Chloromonas sp., which is a common genus of snow algae (Chlorophyta) with red pigments. However, since the amount of algae appeared to be much less than that of mineral particles, their effect on surface albedo is likely negligible. Were the algae to affect the surface albedo significantly, the absorption feature of chlorophyll a would be apparent at a wavelength of 680 nm (e.g. CitationTakeuchi et al., 2006a). However, there was no such absorption at 680 nm in the snow surface spectra, confirming the insignificant contribution of the algal cells to surface albedo reduction.
In contrast to the snow area, surface dust in the ice area contained high levels of organic matter and cyanobacteria. The mass fraction of organic matter in dust on the ice surface (9.4%) was considerably higher than that on the snow surface (5.1%). Microscopy showed that the dust consisted mainly of small brown granules that were not observed on the snow surface (). In addition, their size, composition, and structure resemble those of cryoconite granules, already reported on a Himalayan glacier, growing as an algal mat on the glacial surface (CitationTakeuchi et al., 2001a). Thus, these granules are likely to be the products of biological activity on the glacial surface. Although the mass fraction of organic matter in the dust was only 9.4% in dry weight, microscopy shows that the organic matter was comparable to the mineral components in terms of particle volume. The smaller mass fraction of organic matter is likely due to its lower density compared with that of mineral particles. In the ice-surface spectra, an absorption of around 680 nm was apparent, and was presumably due to the chlorophyll a of snow algae and cyanobacteria on the glacial surface (). This indicates that organic components also exert a major influence on the surface albedo. Although the quantitative contribution of each component to albedo reduction is uncertain, both organic and inorganic components are likely to be effective in reducing the surface albedo on this glacier.
The amount of total (organic plus inorganic) surface dust on the ice surface of the Ürümqi Glacier No. 1 is roughly equivalent to those on other glaciers in China and the Himalayas, but is markedly greater than on glaciers in other parts of the world. According to previous studies, the average amount on the July 1st Glacier in the Qilian Mountains, China, was 292 g m−2 (CitationTakeuchi et al., 2005). The amount of dust recorded on the Yala Glacier in the Himalayas was 225 g m−2 (CitationTakeuchi et al., 2000). These amounts are roughly comparable to that on the Ürümqi Glacier No. 1. In contrast, the amounts of surface dust are reported to be less than 100 g m−2 on glaciers in the Canadian Arctic, Alaska, and Patagonia (CitationTakeuchi, 2002a; CitationTakeuchi et al., 2001b, Citation2001c; ). Thus, higher amounts of surface dust may be a common characteristic of Asian glaciers.
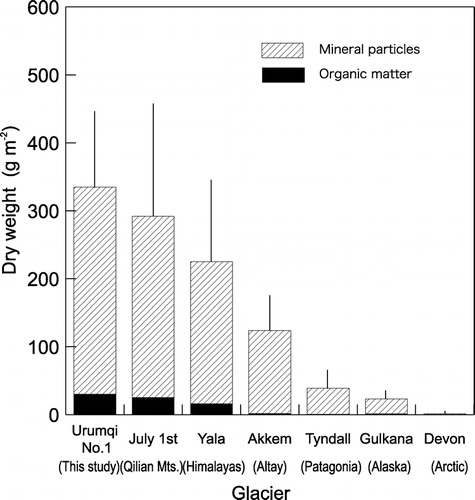
Comparisons of dust components among a number of glaciers showed that both organic and inorganic components were higher on the Ürümqi Glacier No. 1 compared with those on the other glaciers (). The amount of mineral dust on the Ürümqi Glacier No. 1 was approximately 15 times higher than the mean of that on the Patagonian, Alaskan, and Canadian Arctic glaciers (297 versus 20.3 g m−2), while the amount of organic matter was approximately 45 times higher (30 versus 0.67 g m−2). The amounts of both mineral dust and organic matter on Ürümqi Glacier No. 1 are also higher when compared with other Asian glaciers (). The higher levels of inorganic components on the Ürümqi Glacier No. 1 are probably due to its exposure to abundant wind-blown deposits of desert dust, while those of organic components may be the result of intensive biological productivity on the glacial surface.
Long-term mass balance measurements of the Ürümqi Glacier No. 1 have shown a negative mass balance from 1958 to 2003 (−10,032 mm), which is equivalent to a glacial thinning of 11.1 m (CitationYe et al., 2005). In particular, mass balance change has been continuous and highly negative in a recent 5-year period (1997–2002). Analysis of the mass balance and climate data suggests that the negative mass balance of the glacier is due to an increase in summer temperatures (CitationYe et al., 2005). Warmer summer temperatures could increase the frequency of rain instead of snowfall. Snowfall could cover the ablation ice surface, raise its albedo, and thus decrease its melting. However, increase of the frequency of rain could allow the dusty ablation surface to be exposed for a longer time (CitationFujita and Ageta, 2000). Therefore, the effect of surface dust on glacial melting could drastically increase when summer temperature increases. Thus, an enormous accumulation of surface dust probably accounts for the recent accelerated shrinkage of the glacier.
Our results show that organic matter contributes significantly to the reduction in surface albedo and may affect ablation of the glacial surface. Previous studies have shown that anthropogenic pollution was a significant factor in altering the chemical conditions of snow and ice on this glacier (e.g., CitationLee et al., 2003). Such chemical alterations of snow and ice may affect the biological community, changing the amount of organic dust, and then possibly altering the glacial surface albedo. Further study is necessary to improve our understanding of what accounts for variations in a biological community and to evaluate better the effect of microbial activity on glacial surface albedo.
Acknowledgments
We wish to thank Wan Feiteng, Zhou Ping, and other staff and students of the Cold and Arid Regions Environment and Engineering Research Institute of the Chinese Academy of Science in Lanzhou, China, for their valuable logistical support of the field work. This study was financially supported by a Grant-in-Aid for Young Scientists (A, No. 18681005) and a Grant-in-Aid for Scientific Research (B, No. 19310020) of the Japan Society for the Promotion of Science (JSPS) and also partly by the Ili Project funded by the Research Institute for Humanity and Nature, Japan. We also thank two reviewers (Todd Hinkley and anonymous) and an associate editor, Dan Muhs, for helpful suggestions that very much improved the manuscript.
References Cited
- Bai, Z. and X. Yu . 1985. Energy exchange and its influence factors on mountain glaciers in west China. Annals of Glaciology 6:154–157.
- Brock, B. W. , I. C. Willis , and M. J. Sharp . 2000. Measurement and parameterization of albedo variations at Haut Glacier d'Arolla, Switzerland. Journal of Glaciology 46:155 675–688.
- Cutler, P. M. and D. S. Munro . 1996. Visible and near-infrared reflectivity during the ablation period on Peyto Glacier, Alberta, Canada. Journal of Glaciology 42:141 333–340.
- Dean, W. E. 1974. Determination of carbonate and organic matter in calcareous sediments and sedimentary rocks by loss on ignition; comparison with other methods. Journal of Sedimentary Research 44:242–248.
- Fujita, K. 2007. Effect of dust event timing on glacier runoff; sensitivity analysis for a Tibetan glacier. Hydrological Processes 21:2892–2896.
- Fujita, K. and Y. Ageta . 2000. Effect of summer accumulation on glacier mass balance on the Tibetan Plateau revealed by mass-balance model. Journal of Glaciology 46:153 244–252.
- Han, J. , M. Nakawo , K. Goto-Azuma , and C. Lu . 2006. Impact of fine-dust air burden on the mass balance of a high mountain glacier: a case study of the Chongce ice cap, west Kunlun Shan, China. Annals of Glaciology 43:23–28.
- Hoham, R. W. and B. Duval . 2001. Microbial ecology of snow and freshwater ice. In Jones, H. G. , J. W. Pomeroy , D. A. Walker , and R. W. Hoham , editors. Snow Ecology Cambridge Cambridge University Press. 168–228.
- Kohshima, S. 1987. Glacial biology and biotic communities. In Kawano, S. , J. H. Connell , and T. Hidaka , editors. Evolution and Coadaptation in Biotic Communities Kyoto Faculty of Science, Kyoto University. 77–92.
- Kohshima, S. , K. Seko , and Y. Yoshimura . 1993. Biotic acceleration of glacier melting in Yala Glacier, Langtang region, Nepal Himalaya. Snow and Glacier Hydrology (Proceeding of the Kathmandu Symposium, November 1992), IAHS Publication 218:309–316.
- Lee, X. , D. Qin , G. Jiang , K. Duan , and H. Zhou . 2003. Atmospheric pollution of a remote area of Tianshan Mountain: ice core record. Journal of Geophysical Research 108:D144406. , doi:10.1029/2002JD002181.
- Li, Z. , R. Edwards , E. Mosley-Thompson , F. Wang , Z. Dong , X. You , H. Li , C. Li , and Y. Zhu . 2006. Seasonal variability of ionic concentrations in surface snow and elution processes in snow-firn packs at the PGPI site on Ürümqi Glacier No. 1, eastern Tien Shan, China. Annals of Glaciology 43:250–256.
- Meier, M. F. , M. B. Dyurgerov , K. R. Ursula , S. O'Neel , W. T. Pfeffer , R. S. Anderson , S. P. Anderson , and A. F. Glazovsky . 2007. Glaciers dominate eustatic sea-level rise in the 21st century. Science 317:1064–1067.
- Oerlemans, J. 2005. Extracting a climate signal from 169 glacier records. Science 308:675–677.
- Paterson, W. S. B. 1994. The Physics of Glaciers. 3rd edition Oxford Elsevier. 480 pp.
- Sun, J. 2002. Source regions and formation of the loess sediments on the high mountain regions of northwestern China. Quaternary Research 58:341–351.
- Takeuchi, N. 2001. The altitudinal distribution of snow algae on an Alaska glacier (Gulkana Glacier in the Alaska Range). Hydrological Processes 15:3447–3459.
- Takeuchi, N. 2002a. Surface albedo and characteristics of cryoconite (biogenic surface dust) on an Alaska glacier, Gulkana Glacier in the Alaska Range. Bulletin of Glaciological Research 19:63–70.
- Takeuchi, N. 2002b. Optical characteristics of cryoconite (surface dust) on glaciers: the relationship between light absorbency and the property of organic matter contained in the cryoconite. Annals of Glaciology 34:409–414.
- Takeuchi, N. , S. Kohshima , Y. Yoshimura , K. Seko , and K. Fujita . 2000. Characteristics of cryoconite holes on a Himalayan glacier, Yala Glacier central Nepal. Bulletin of Glaciological Research 17:51–59.
- Takeuchi, N. , S. Kohshima , and K. Seko . 2001a. Structure, formation, darkening process of albedo reducing material (cryoconite) on a Himalayan glacier: a granular algal mat growing on the glacier. Arctic, Antarctic, and Alpine Research 33:115–122.
- Takeuchi, N. , S. Kohshima , T. Shiraiwa , and K. Kubota . 2001b. Characteristics of cryoconite (surface dust on glaciers) and surface albedo of a Patagonian glacier, Tyndall Glacier, Southern Patagonia Icefield. Bulletin of Glaciological Research 18:65–69.
- Takeuchi, N. , S. Kohshima , K. Goto-Azuma , and R. M. Koerner . 2001c. Biological characteristics of dark colored material (cryoconite) on Canadian Arctic glaciers (Devon and Penny ice cap). Proceeding of the Memoirs of National Institute of Polar Research Special Issue, 54. 495–505.
- Takeuchi, N. , Y. Matsuda , A. Sakai , and K. Fujita . 2005. A large amount of biogenic surface dust (cryoconite) on a glacier in the Qilian Mountains, China. Bulletin of Glaciological Research 22:1–8.
- Takeuchi, N. , R. Dial , S. Kohshima , T. Segawa , and J. Uetake . 2006a. Spatial distribution and abundance of red snow algae on the Harding Icefield, Alaska derived from a satellite image. Geophysical Research Letters 33:L21502. , doi:10.1029/2006GL027819.
- Takeuchi, N. , J. Uetake , K. Fujita , V. Aizen , and S. Nikitin . 2006b. A snow algal community on Akkem Glacier in the Russian Altai Mountains. Annals of Glaciology 43:378–384.
- Wake, C. P. , P. A. Mayewski , P. Wang , Q. Yang , J. Han , and Z. Xie . 1992. Anthropogenic sulfate and Asian dust signals in snow from Tien Shan, northwest China. Annals of Glaciology 16:45–52.
- Wake, C. P. , P. A. Mayewski , Z. Li , J. Han , and D. Qin . 1994. Modern eolian dust deposition in central Asia. Tellus 46b:220–233.
- Ye, B. , D. Yang , K. Jiao , T. Han , Z. Jin , H. Yang , and Z. Li . 2005. The Ürümqi River source Glacier No. 1, Tianshan, China: changes over the past 45 years. Geophysical Research Letters 32:L21504. , doi:10.1029/2005GL024178.
- Zeng, Q. , M. Cao , X. Feng , F. Liang , X. Chen , and W. Sheng . 1984. Study on spectral reflection characteristics of snow, ice and water of northwest China. Scientia Sinica, B 27:6 647–656.