Abstract
Data for Rhizocarpon agg. thalli at the Illecillewaet Glacier in British Columbia were used to see whether a single growth curve could provide accurate age estimates on either side of the Continental Divide, to determine whether modern and historical growth rates are similar, and to test a published model that estimates lichen age from short-term radial growth rates. A lichen growth curve was developed for Rhizocarpon agg. using thallus-size data from 14 tree-ring and historically dated substrates. Comparison of this curve with one developed on similar materials 250 km north of this location found similar growth rates for the first 150 yr but slightly faster growth over the next 150 yr at the Illecillewaet site. Radial growth was also measured annually at an average of five points at 105 Rhizocarpon agg. thalli to see if direct-measurement data could be used to reliably estimate lichenometric ages. Radial growth from 1996–2000 ranged between 0.262 and 0.412 mm yr−1and showed large variation within and between thalli. Mathematical analysis found that radial growth was not a positive function of the radius, and linear regression incorrectly predicted that growth rates increase with thallus size. Ages estimated by linear extrapolation of the 4-yr mean growth rate were ca. 10 yr less than those estimated by the indirectly calibrated growth curve on surfaces <200 yr old. Progressively less accurate minimum estimates were obtained using linear extrapolation of radial growth rates for old surfaces and thalli >60-mm diameter. These findings lend support to the assumption that modern growth rates of Rhizocarpon lichens averaged over several years can potentially provide close estimates of lichenometric age.
Introduction
Lichenologists and users of lichenometry tend to use different approaches to estimate the radial growth rates of crustose lichens. Typically, lichenologists use calipers or photogrammetry to measure radial growth rates (e.g., CitationHooker and Brown, 1977; CitationBenedict, 1990), while users of lichenometry develop lichen growth curves by assuming that thalli on surfaces of known age are representative of radial growth over the long term (e.g., CitationMcCarthy and Smith, 1995). Since these two approaches are seldom used together and do not generate growth rates that cover the same time interval, it is unclear whether modern and historical growth rates are significantly different. This uncertainty is of particular concern in situations where archival or tree-ring dating controls are unavailable and linear extrapolation of short-term growth rates is by default used to estimate lichenometric ages (e.g., in arctic tundra: CitationHaworth et al., 1986; CitationMiller, 1973). Because traditional lichenometric approaches depict the long-term growth trends of crustose lichens by a curved line, there is reason to question whether linear extrapolation of short-term growth rates can indeed provide close estimates of substrate age.
Presumably, if direct measurements of lichen growth were undertaken at a site where lichen growth curves have already been calibrated by indirect means, it might be possible to test whether modern and historical growth rates are similar. However, in the western Canadian cordillera, forefields of Holocene mountain glaciers where growth curves have been calibrated are now either severely disturbed by human activity (e.g., Mt. Edith Cavell: CitationLuckman, 1977) or are too remote to be visited annually (e.g., Bennington Glacier: CitationMcCarthy, 1985). Accordingly, this paper describes work done at an accessible yet protected site where no previous lichenometric work had occurred.
This study has three components. The first is the development of a growth curve that estimates long-term growth from lichen sizes on historical and tree-ring dated surfaces formed by Holocene glaciation. The second component is an attempt to use direct measurements to determine the annual radial growth rate of lichens. The final section of the paper examines the accuracy of methods that can be used to estimate lichenometric ages from annual growth-rate data.
Study Site
The Illecillewaet Glacier (51°14′N, 117°26′W) is located in the Columbia Mountains in Glacier National Park, approximately 75 km west of Golden, British Columbia (). Although the glacier terminus has long been a popular destination for hikers, the forefield has been undisturbed by human activity and has an exceptionally good photographic and documentary record dating from 1887 (e.g., CitationCavell, 1983; CitationPutnam, 1982; CitationChampoux and Ommanney, 1986). Some of the historical data can be used to establish precise deglaciation dates for the conifer, shrub, and lichen communities that have developed on the quartzite bedrock exposed in the forefield. While terminal moraines that were formed here prior to the late 1800s have either been destroyed or reworked by streams and snow avalanches, most of the 18 morainic ridge segments found on the crest and sides of the lateral moraines are well preserved and support both trees and lichens. Accordingly, fieldwork was undertaken during the summers of 1986, 1996, and 2000 to map and morphologically correlate the morainic ridge segments () and to collect the thallus-size and tree-age data needed to calibrate a lichen growth curve.
CORRELATION OF MORAINIC RIDGES
The forefield is bordered by a pair of large lateral moraines (ca. 30 m tall), and several minor ridges (0.5 to 3 m high) are superimposed on the flanks of these laterals. Each of the morainic ridges was mapped and correlated on the basis of morphology and position relative to the outermost ridge on each side of the forefield.
On the eastern side of the valley the outer ridge (Moraine 1 East) was a 2-m-high mound of moss-, shrub-, and sapling-covered boulders that was traced for about 75 m downvalley from the distal crest of the main lateral moraine (). It had few mature trees, and crustose lichens were scarce in the small clearings (covering ca. 100 m2) on the ridge crest. In contrast, Moraine 1 West was much larger (ca. 5–8 m high by 1.5 km long), was completely shrub and tree covered, and had no lichens. Though Moraines 1 and 2 were easily traced for several hundred meters outside the area shown in , those areas have been severely reworked by streams, snow avalanches, and rockslides and did not support useful lichens or trees. However, fragments of younger moraines and lines of boulders that survived stream and avalanche activity were followed across the center of the valley and showed direct correlation of Moraines 6 and 7. Though tree and shrub cover was dense on the valley floor, large sections of Moraines 2 to 8 had no trees and were ideal for lichenometry.
Method One: Calibration of a Lichen Growth Curve by Indirect Measurement
TREE-RING DATING OF MORAINES
Tree-ring dating in the forefield involved collecting increment cores from the base of about 300 living trees, including all mature trees found on or between the morainic ridge segments. In each case the tree species, location, sampling height, and site conditions were noted. When coring, the first core was usually taken at about a 15-cm height on the main stem so as to estimate pith location before collecting a better-aimed sample lower on the tree. Inspection of the cores generally showed there was <1-yr difference between cores taken <10 cm apart. However, in a few cases 1 to 3 yr were added to an earliest ring date to compensate for a missed pith or for years lost owing to sampling above 15 cm on the main stem. This approach recognizes that age errors owing to missed piths can be considerable, but such errors are often minor and can often be accurately estimated by studying ring curvature in several cores from young, rapidly growing, undeformed stems. Repeat sampling at various heights in several trees in this study suggest that apical growth at this site is similar to that reported by CitationMcCarthy et al. (1991) at other forefields in the region.
Tree ages on surfaces deglaciated at known times were used to estimate tree ecesis. The results () show that the shortest ecesis was <5 yr for a spruce (Picea engelmannii Parry ex. Engelm.) growing on bedrock at the 1931 ice-front position, 26 yr for alpine fir (Abies lasiocarpa [Hook.] Nutt.) on a bouldery moraine, and 32 yr for both spruce and fir on bedrock. While the data illustrate that ecesis can be quite short and is inherently difficult to accurately estimate, the mean ecesis of the five oldest trees on each control site () suggests that yr is common. Accordingly, an ecesis of 35 yr will be added to the germination ages of the oldest trees on each of the morphologically correlated ridges () to more closely estimate the timing of moraine stabilization.
There is little evidence of substrate disturbance at these sites, but two of the units (Moraine 1 East and Moraine 4 West) support living trees that are tilted or have lost branches due to snow avalanches. In addition, a small snag (sample L5-S1), measuring about 2 m tall by 6 cm diameter at its base, was found rooted on the crest of Moraine 2 West. A disc was cut from the base of the snag, and its 88 growth rings were measured and compared with a 307-yr ring-width chronology for mountain hemlock (Tsuga mertensiana [Bong.] Carr.) that was developed at a site <1 km from this location (CitationMcCarthy and Taylor, 2001). Cross-dating using the program COFECHA indicates that the snag germinated in 1728 and died in 1816. With an adjustment of 35 yr for ecesis, this indicates that Moraine 2 West had formed by 1693.
LICHEN SIZES ON THE MORAINES
The lichenometric method used to calibrate the historical growth rate (growth curve) is comparable with that of CitationLuckman (1977). This method involved using a flexible plastic ruler to measure the maximum diameter of the largest thalli on quartzite boulders on each deposit (). Measurements are accurate to the nearest millimeter, and only yellow-green Rhizocarpon agg. thalli that had oval to circular outlines were considered. None of the lichens measured was near a stream or on a mossy or deeply shaded site. Though many thalli were measured, maximum diameters of only 5–10 of the largest thalli on each unit were recorded.
Comparison of tree ages and thallus sizes on individual ridges () shows that most of the largest thalli were found on the shaded western side of the forefield, while many of the landform ages were developed using trees on the sunnier eastern moraine complex. This difference suggests that lichens and trees responded differently to local environmental conditions and shows that correlation of moraines would have been difficult if it were based solely on tree age or lichen size.
GROWTH CURVE CONSTRUCTION AND EVALUATION
Dating controls have been established for 14 locations (). With the exception of control point 4 (a single boulder measuring ∼5 m3), each control surface was >50 m2 and included a wide range of thallus sizes and growing environments. These details are important because the likelihood of finding a larger thallus tends to rise as search-area size and the range of microenvironments sampled increases.
shows a curved line that was drawn to link two historically dated surfaces (i.e., points 1 and 4) and connect points 6, 8, and 14. Point 5 is below the line, indicating that tree ecesis was <35 yrs at that site, while points that are above the line (7, 9, 10, 11, 12, and 13) show that tree establishment took from 50 to 135 yr (points 7 and 12) at those sites. Ecesis variability is not uncommon in glacier forefields (e.g., CitationMcCarthy and Luckman, 1993: ) and reflects the fact that conditions on the moraines were suitable for lichen establishment and growth but unsuitable for tree establishment and survival.
Comparison with the growth curve developed by CitationLuckman (1977) at a site that has similar substrate lithology () shows that similar growth rates exist for the first century (i.e., the great growth period). However, slightly faster growth occurred over the next 200 yr at the Illecillewaet, possibly caused in part by different moisture regimes. Precipitation normals for meteorological stations nearest these sites indicate that the Illecillewaet site (Golden, B.C.) receives about 950 mm of precipitation, while Mt. Edith Cavell (Jasper, Alberta) receives about 394 mm each year (http://www.msc-smc.ec.gc.ca/climate/climate_normals/index_e.cfm). The precipitation differences are clearly reflected in the forest cover, with cedar and hemlock forests near the Illecillewaet Glacier and spruce and pine forests at Mt. Edith Cavell.
Method Two: Direct Measurement of Radial Growth
A 5-m-wide by 25-m-long section of the crest of Moraine 6 West () (at 1468 m elevation, 51°15.114′ N, 117°26.228′ W) was selected as a direct-measurement study site. This area is free of human disturbance and has no mosses or standing water but has several of the largest thalli on the moraine and few trees or higher plants. Yellow-green Rhizocarpon thalli cover about 10% of exposed surfaces on this moraine.
Direct measurement of growth was done using digital calipers. Consequently, only thalli that were located where calipers could be easily positioned were considered as candidates for measurement. Initially, 112 thalli (619 fixed measurement points) were selected so as to include approximately 10 thalli in each of 12 size classes from the 0- to 4.99-mm size class and rising in increments of 5 to the 45- to 49.99-mm size class. Each thallus had distinct margins and clear peripheral zones and was on a rock that was embedded in the moraine. No attempt was made to restrict sampling to one particular aspect or slope on the rock, but all thalli share the same general microclimate and are on the same quartzite lithology. Consequently, the measured growth rates are assumed to represent radial growth under microenvironmental conditions that are typical of exposed moraines in this and nearby valleys.
The ground-level microclimate can be characterized using data collected by Stow-Away® data loggers that were placed in a wire cage at this site in 1996–1997 (). The data show that the summer and fall microenvironment has large diurnal shifts in temperature and humidity. However, in the winter and spring a snowpack up to 3 m thick kept the lichens at or very near 0°C and 100% relative humidity. Nonetheless, a late-December thaw showed that even in midwinter there can be both snow-free opportunities for growth and the risk of premature death if thalli are sealed under refrozen meltwater (e.g., CitationBenedict, 1990).
In July of 1996, reference points were placed along the upper and downslope edges of thalli. Dots (ca. 5-mm diameter) were first painted on the rock about 1 cm from the thallus using white, oil-based typewriter correction fluid. Each thallus and its fixed points were numbered, reference crosses were marked using waterproof India ink and a 0000 Rapidograph pen, and the markings were sealed with varnish (). Some of the growth-rate measurements were done to points arbitrarily selected along the thallus margin, but most were done to hyphae that protruded from the general thallus outline. These prominences and points along the thallus margin will, for convenience, be referred to as “lobes”—even though they are not “lobes” in the sense in which the term is normally applied to lichens (S. Clayden, pers. comm. 1999).
Measurements were made annually from the nearest edge of the reference crosses to the edges of dry thalli. Digital calipers, a 4× head-mounted magnifier, and a voice-activated tape recorder were used to collect the measurement data. All measurements were done by the author. Errors in measurement are expected to be close to the manufacturer's estimates of instrumental error (0.02 mm, Starrett Manufacturing Co.). An estimated maximum error (error in reproducibility) was obtained by comparing 70 repeat measurements (not at the same time) done on 40 randomly selected thalli (). Actual errors in measurement are expected to be smaller because in practice, each measurement was cross-checked in the field to ensure that it was within acceptable tolerances (±0.025 mm). In a few cases measurements were difficult to reproduce because the caliper blades damaged the prothallus or could not be rested on the crosshairs (e.g., because of minor irregularities on the rock surface). In addition, some of the measurements were rejected because I had to assume an awkward position to reach the thallus or repeat measures could not be made to some damaged reference points or to fragile hyphae. Over the 4-yr period these factors reduced the total number of thalli being monitored from 112 to 105.
In 1997 the lichenologist K. Glew identified all thalli to section and in some cases species level. Reagents were used sparingly near the downslope margin of the thallus. Excess chemicals were quickly blotted from the thallus, and, when necessary, apothecia were removed to allow microscopic examination of spores. Measurable regrowth is occurring in most of the excised patches (see CitationArmstrong. and Smith, 1987, Citation1996), and the disturbance is expected to have low or no impact on the radial growth rates. All yellow-green-black Rhizocarpon agg. thalli were identified as Rhizocarpon section Rhizocarpon (most were Rhizocarpon lecanorinum Anders), and a thorough search of adjacent moraines failed to find thalli from the Rhizocarpon section Alpicola group. This finding is consistent with reports from Europe (e.g., CitationInnes, 1988:75) that describe some members of the section Alpicola as being late-successional species.
RESULTS
Summary data for “lobe” growth (, ) show that mean growth rates ranged from 0.26 to 0.41 mm yr−1. However, a large range (2.07 to 3.12) and standard deviation (0.28 to 0.34) shows that there was considerable scatter around the mean growth rate, and the mode (0.08 to 0.40) reveals that many locations along the thallus margins did not grow radially every year. Scatterplots and linear regression of maximum thallus diameter and the mean annual growth rates () show that thallus diameter is a poor predictor of mean annual radial growth rate and in 3 of 4 yr, regression lines with a positive slope incorrectly predict that growth rates increase with thallus diameter. Consequently, linear regression of class means should not be used to identify growth trends or estimate lichenometric ages.
Method Three: Estimation of Long-Term Growth from Short-Term Data
It is unclear how best to use direct-measurement data as a basis for estimating lichenometric age. Some studies have assumed that a growth curve will have a linear form (e.g., CitationMiller, 1973; CitationHaworth et al., 1986), while others have attempted to develop and apply models that are consistent with the known growth characteristics of lichens (e.g., CitationAplin and Hill, 1979; CitationProctor, 1983). These two approaches will be attempted in the following sections.
LINEAR EXTRAPOLATION OF SHORT-TERM GROWTH RATES
The dotted lines seen in highlight age predictions derived by using the growth curve () and ages estimated by linear extrapolation of the annual means and the 4-yr mean growth rate (). Comparison of the two graphs ( & B) shows that for the first 200 yr, both the mean growth measured in 1997–1998 and the 4-yr mean growth rate predict substrate ages that were within ca. 10 yr of those estimated by the indirectly calibrated growth curve (). Larger underestimates of age were generated for thallus diameters >60 mm and surfaces >200 yr old, suggesting that the directly measured growth rates poorly represent growth in thalli that were larger than those being monitored. The findings demonstrate that linear extrapolation of longer-term mean growth rates has the potential to provide conservative estimates of substrate age. Nonetheless, final judgment on the suitability of using linear extrapolation to estimate substrate age must await the findings of more uniformly controlled studies.
APLIN AND HILL ANALYSIS
The lichen growth model developed by CitationAplin and Hill (1979) is based on biological principles and has provided a good fit when used with growth data for several lichen species (e.g., CitationProctor, 1983). However, CitationArmstrong (1983) used the Aplin and Hill model with growth data from 39 Rhizocarpon thalli and found that the model may be appropriate only for a small portion of the Rhizocarpon growth cycle (e.g., between ca. 7 and 16 mm diameter). In contrast, CitationProctor (1983) had some success in using the Aplin and Hill approach to construct Rhizocarpon growth curves for lichenometric dating. These curves provided an estimate of growth rates starting at a fixed time (i.e., radial growth after an estimated ecesis), and CitationProctor (1983: 258) reported that they gave “an acceptable account of the field observations on thalli of R. geographicum in the Valsorey up to ages of 100 years or so.”
The CitationAplin and Hill (1979) approach first involves calculation of the radial growth rate (Δr) and the increase in the natural logarithm of the radius (Δlnr). In this study the thallus radius (r) will be the mean of the longest and shortest axis measured at time zero (r0), and Δr is represented by the mean growth of all measured “lobes” of that thallus. Individual years will be treated as separate data sets, and the value used for radius at the end of year one (r1) will be adjusted for growth that occurred in the previous year [i.e., (Δr0)]. The second step in the Aplin and Hill approach involves the production of a graph that plots radial growth rate (Δr) against change in the natural logarithm of the radial change
that occurred during the measurement period (Δt). The graph should reveal a linear relationship (CitationHill, 1981: 276), and a line fitted to these data can then be used to obtain the starting constants α (dimensions : time−1) and s (distance) that are needed to develop the growth curve.
Unfortunately, analysis using the Illecillewaet data found that the radial growth rates are positively correlated with the natural logarithm of the change in radius. This finding indicates that radial growth is not a positive function of the radius and that the Aplin and Hill approach is inappropriate for these data. The same finding was reported by CitationArmstrong (1983:620).
Discussion and Conclusion
Data presented in this paper confirm the lichen growth trends indicated by the first century of growth at Mt. Edith Cavell (CitationLuckman, 1977) and suggest that after ca. 150 yr slightly faster growth occurs at the wetter Illecillewaet study site. The direct-measurement data show that considerable variability in radial growth occurs both within and between thalli and that linear regression or linear extrapolation of mean growth rates can lead to less accurate estimates than can be developed using traditional approaches to lichen growth-curve calibration. Linear extrapolation of the 4-yr mean provided close minimum estimates of substrate age for the first two centuries of growth. This demonstrates that linear extrapolation has considerable potential as a lichenometric technique. My finding that the CitationAplin and Hill (1979) model was inappropriate for these data once again calls attention to the need for models that can be used to accurately calibrate lichen growth and estimate lichenometric ages. The fact that such a fundamental need remains unfilled nearly 50 yr after lichenometry was pioneered by Beschel (e.g., CitationBeschel, 1957), suggests that the topic either is exceptionally challenging or has yet to attract sufficient interest.
Good agreement between the new and existing growth curves for Rhizocarpon agg. suggest that there is no need to use complex and tedious search and measurement strategies (e.g., use calipers, quadrats, and statistical models) in order to develop accurate lichenometric ages in this region. Nonetheless, users of lichenometry on acid rocks in the Canadian cordillera should either develop their own curve or use the Rhizocarpon agg. growth curve that most closely matches the moisture regime at their study site. In the absence of meteorological data, forest cover type or position east or west of the Continental Divide can be used to assist in selecting the most appropriate growth curve. In addition, surveys of lichen thalli at this study site indicated that thalli in the Rhizocarpon section Alpicola were either absent from or very difficult to find in the Illecillewaet forefield. Our inability to find Rhizocarpon section Alpicola thalli in this forefield lends support to anecdotal reports that species in this group tend to arrive late in the lichen successional sequence (e.g., CitationBickerton and Matthews, 1992; CitationInnes, 1982, Citation1983) and suggests that concern about age errors owing to the possible inclusion of Rhizocarpon section Alpicola thalli in existing growth curves (e.g., CitationLuckman and Osborn, 1979) may be unfounded.
This is the first time that lichenometry has been used in the Columbia Mountains. Its successful use shows that lichenometry has considerable utility even at subalpine sites. While greater opportunities for lichenometric dating occur above timberline in this area, this work has shown that lags in tree ecesis can make it difficult to use tree-ring dating to calibrate lichen growth curves. Despite this, the study showed that even in the rainforests of British Columbia, small snags can remain intact and useful as dating controls for at least two centuries. Thus, there is hope that dendrochronology can be used to establish even older control points for lichen growth curves and develop closer ages for early Little Ice Age deposits in this region.
FIGURE 2. Sketch maps of the Illecillewaet Glacier forefield. Dated ice-front positions and historical details are based on maps and photographs by the Vaux family (CitationVaux and Vaux, 1907 and photo NG4-894—Archives of the Canadian Rockies, Banff). Dashed lines show ridges that are hidden from view by dense shrub cover or have been removed by erosion since the late 1880s. Morainic ridge segments have been correlated based on their size and position (A). The germination dates of the oldest trees on each ridge segment are also shown (B)
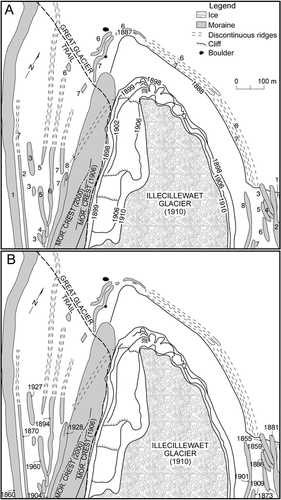
FIGURE 3. Scatterplot showing the control points and growth curve developed in this study. Open circles show the age of each control-point surface and the maximum diameter of the largest Rhizocarpon agg. thallus on each surface. Error bars show the adjustments made for tree ecesis. Dating controls are summarized in
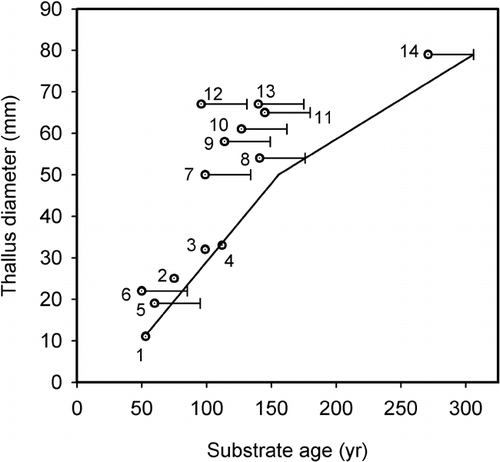
FIGURE 4. Comparison of the lichen growth curve developed in this study (solid line) and a growth curve developed on similar substrates at Mount Edith Cavell (CitationLuckman, 1977). Open circles (this study) and triangles (CitationLuckman, 1977) show the age of each control point surface and the maximum diameter of the largest Rhizocarpon agg. thallus on each surface. Error bars show the adjustments made for tree ecesis. Dating controls are summarized in and in CitationLuckman (1977)
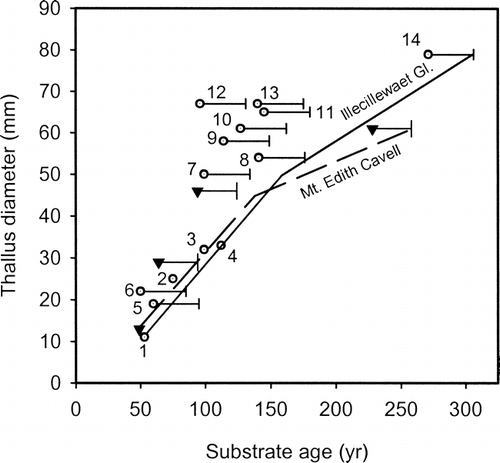
FIGURE 5. Graph of air temperature (A) and relative humidity (B) collected in 1996. The data summarize hourly readings by sensors that were placed on a rock in the middle of the study site. The humidity record is incomplete owing to sensor failure linked to the melting of a 2–3-m-deep snowpack
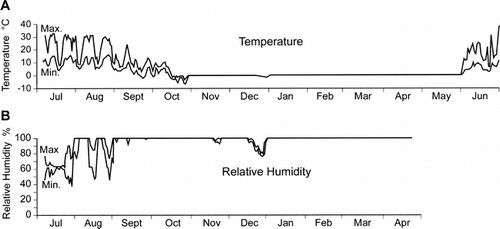
FIGURE 6. Photograph of a Rhizocarpon lecanorinum thallus with fixed points. This photo shows the thallus in July 2000. A white line has been drawn on the image to mark the approximate edge of the thallus in July 1996. Since radial growth tends to be uneven, measurements were often made to five or more fixed points at each thallus. The circles and arrows drawn on this image highlight two of the small bulges (“lobes”) where growth was measured on this thallus. The scale bar measures 10 mm
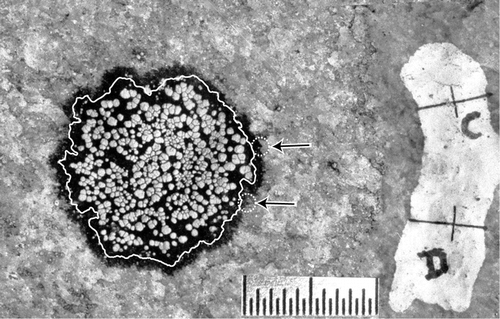
FIGURE 7. Scatterplots (A, B, C, D) summarize the annual radial growth rate measured in 105 thalli. Each dot represents the mean growth rate in a 5-mm size class. The dotted line in each graph represents the mean growth rate measured in that year. The histogram (E) shows the number of thalli measured in each 5-mm size class
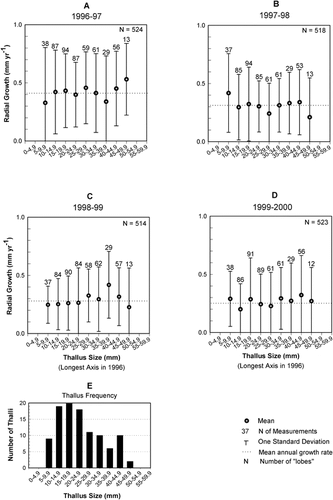
FIGURE 8. Scatterplot showing linear regression of thallus size against mean radial growth rates for each thallus size class in all years. Weak correlation coefficients and linear regression lines with a positive slope (years 1996–1997, 1997–1998, and 1998–1999) hint that radial growth may increase slightly as thallus size increases
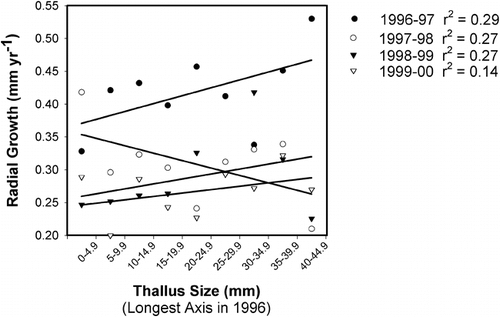
FIGURE 9. Scatterplots comparing ages estimated by the Illecillewaet growth curve (A) and the means of directly measured radial growth rates (B). Dotted lines represent thallus size–substrate age relationships of 20-, 40-, 60-, and 70-mm thalli. Comparison of the two approaches shows that in the first two centuries of growth (up to ca. 60-mm thallus diameter), ages estimated using the 4-yr mean growth rate (1996–2000) are 10 yr younger than those estimated by the indirectly calibrated growth curve (graph A). Larger underestimates of age are predicted for surfaces >200 yr old and thalli >60 mm diameter
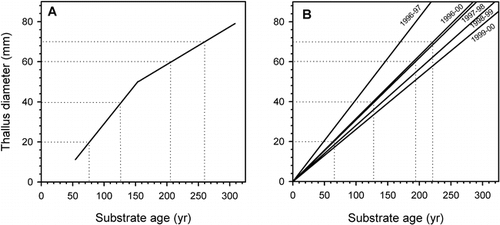
TABLE 1 Tree ecesis data from the Illecilewaet Glacier forefield
TABLE 2 Summary tree-age and lichen-size data for the Illecillewaet Glacier forefield. Thalli first measured in 2000 are indicated by *. All other thalli were measured in 1996
TABLE 3 Historical and dendrochronologic dating controls and Rhizocarpon agg, size (control points) from the Illecillewaet Glacier forefield. Unless otherwise noted, photographs are referred to by call numbers assigned by the Archives of the Canadian Rockies, Banff, Alberta
TABLE 4 Estimated maximum error (error in reproducibility). Measurements were taken on different days from 70 randomly chosen points to estimate the reproducibility of the data. The differences between the two measurements are reported here as the error in reproducibility
TABLE 5 Summary statistics for the annual radial growth rate measurements
Acknowledgments
I wish to thank the many people and agencies who supported this work. Funding was provided by grants from the Royal Canadian Geographical Society (1986), Brock University (1996), and the Natural Sciences and Engineering Research Council of Canada (1997–2000). The staff of Glacier National Park allowed me to work at this site, trusted me not to cut down trees or paint too many rocks, and gave me a dry place to stay. Dave Seaborn cored trees and helped to map the moraines in the wet summer of 1986. Eva Sadowski, Craig Barr, and Andy Brown served as field assistants in 1998. Mike Lozon of Brock University produced the figures, and John R. Taylor developed a tree-ring chronology, cross-dated the critical snag, and generated graphs. Dr. Katherine Glew identified the lichens in the field and gave me a “crash” course on Rhizocarpons. Parker Calkin and an anonymous reviewer provided helpful comments on an earlier version of this paper.
References Cited
- Aplin, P. S. and D. J. Hill . 1979. Growth analysis of circular lichen thalli. Journal of Theoretical Biology 78:347–363.
- Armstrong, R. A. 1983. Growth curve of the lichen Rhizocarpon geographicum . New Phytologist 94:619–622.
- Armstrong, R. A. and S. N. Smith . 1987. Development and growth of the lichen Rhizocarpon geographicum . Symbiosis 3:287–300.
- Armstrong, R. A. and S. N. Smith . 1996. Experimental studies of hypothallus growth in the lichen Rhizocarpon geographicum . New Phytologist 132:123–126.
- Benedict, J. B. 1990. Experiments on lichen growth. 1. Seasonal patterns and environmental controls. Arctic and Alpine Research 22:244–254.
- Beschel, R. 1957. A project to use lichens as indicators of climate and time. Arctic 10:60.
- Bickerton, R. W. and J. A. Matthews . 1992. On the accuracy of lichenometric dates: an assessment based on the ‘Little Ice Age’ moraine sequence of Nigardsbreen, southern Norway. Holocene 2:227–237.
- Cavell, E. 1983. Legacy in Ice: The Vaux Family and the Canadian Alps. Banff: Altitude Publishing. 98 pp.
- Champoux, A. and C. S L. Ommanney . 1986. Evolution of the Illecillewaet Glacier, Glacier National Park, B.C., using historical data, aerial photography and satellite image analysis. Annals of Glaciology 8:31–33.
- Collier, E. P. 1958. Glacier variation and trends in run-off in the Canadian Cordillera. International Association of Scientific Hydrology, Publication No. 46, General Assembly of Toronto, International Union of Geodesy and Geophysics, 344–357.
- Haworth, L. A. , P. E. Calkin , and J. M. Ellis . 1986. Direct measurement of lichen growth in the central Brooks Range, Alaska, U. S. A., and its application to lichenometric dating. Arctic and Alpine Research 18:289–296.
- Hill, D. J. 1981. The growth of lichens with special reference to the modelling of circular thalli. Lichenologist 13:265–287.
- Hooker, T. N. and D. H. Brown . 1977. A photographic method for accurately measuring the growth of crustose and foliose saxicolous lichens. Lichenologist 9:65–75.
- Innes, J. L. 1982. Lichenometric use of an aggregated Rhizocarpon “species.”. Boreas 11:53–57.
- Innes, J. L. 1983. Use of an aggregated Rhizocarpon “species” in lichenometry: an evaluation. Boreas 12:183–190.
- Innes, J. L. 1988. The use of lichens in dating. In Galun, M. (ed.), Handbook of Lichenology. Boca Raton: C. R. C. Press, Inc., 75–92.
- Luckman, B. H. 1977. Lichenometric dating of Holocene moraines at Mount Edith Cavell Jasper, Alberta. Canadian Journal of Earth Sciences 14:1809–1822.
- Luckman, B. H. and G. D. Osborn . 1979. Holocene glacier fluctuations in the Middle Canadian Rocky Mountains. Quaternary Research 11:52–57.
- McCarthy, D. P. 1985. Dating Holocene geomorphic activity of selected landforms in the Geikie Creek Valley, Mount Robson Provincial Park, British Columbia. M. Sc. Thesis, Department of Geography, University of Western Ontario. 304 pp.
- McCarthy, D. P. and B. H. Luckman . 1993. Estimating ecesis for tree-ring dating of moraines: a comparative study from the Canadian cordillera. Arctic and Alpine Research 25:63–68.
- McCarthy, D. P. and D. J. Smith . 1995. Growth curves for calcium-tolerant lichens in the Canadian Rocky Mountains. Arctic and Alpine Research 27:290–297.
- McCarthy, D. P. and J. R. Taylor . 2001. The development of lichen growth curves, tree-ring chronologies and a field guide to the Great Glacier Trail in Glacier National Park. Report to Glacier National Park, July 2001. 75pp.
- McCarthy, D. P. , B. H. Luckman , and P. E. Kelly . 1991. Sampling height-age correction for spruce seedlings in glacial forefields, southern Canadian cordillera. Arctic and Alpine Research 23:451–455.
- Miller, G. 1973. Variations in lichen growth from direct measurements: preliminary curves for Alectoria miniscula from Eastern Baffin Island, N.W.T., Canada. Arctic and Alpine Research 5:333–340.
- Proctor, M. C F. 1983. Sizes and growth-rates of thalli of the lichen Rhizocarpon geographicum on the moraines of the Glacier de Valsorey, Valais, Switzerland. Lichenologist 15:249–261.
- Putnam, W. L. 1982. The Great Glacier and Its House. New York: American Alpine Club. 223 pp.
- Vaux, G. and W. S. Vaux . 1899. Additional observations on glaciers in British Columbia. Proceedings of the Academy of Natural Sciences of Philadelphia, December, 501–511.
- Vaux Jr., G. and W. S. Vaux . 1907. Glacier observations. Canadian Alpine Journal 1:138–148.
- Webb, C. E. 1948. Investigations of glaciers in British Columbia. Canadian Alpine Journal 31:207–217.
- Wheeler, A. O. 1932. Retreat of ice in the Canadian cordillera, the 1931 expedition. Canadian Alpine Journal 20:120–137.