Abstract
We used integrated multiproxy analysis of a lake sediment core and glacial geomorphology to reconstruct the late Pleistocene and Holocene climate and geomorphic evolution of the Burstall Pass area, Kananaskis Country, Alberta, Canada. Analysis of macrofossils, pollen, sedimentology, and sediment geochemistry from a lake sediment core and geomorphology and tephrochronology of glacial moraines provide evidence for multiple modes of climate during the last ca. 11,000 yr. An advance of the Robertson Glacier prior to ca. 9200 14C BP is correlated to the Crowfoot advance and was the largest of the postglacial period. Immediately following this event, increased lake productivity and the deposition of marl as well as increased arboreal/nonarboreal pollen (AP/NAP) ratios suggest that the climate warmed, possibly accompanied by increased aridity. Decreased turbidity and clastic sediment flux in Lower Burstall Lake during the early Holocene suggest reduced glacial runoff and may indicate the complete ablation of the Robertson Glacier shortly after 10,000 14C BP. Clastic sediment flux to Lower Burstall Lake remained minimal until after ca. 3500 14C BP, when decreasing LOI (loss-on-ignition) organic carbon levels in lake sediments signaled the return of glacial runoff to the lake system. The largest Neoglacial advance in the Burstall Pass area appears to have been the most recent and was followed by rapid recession during the 20th century.
Introduction
Previous paleoclimatic studies examining the late Pleistocene and Holocene glacial history of the front and middle ranges of the Southern Canadian Rocky Mountains have identified three major periods of increased glacial activity since the late Wisconsinan. On the basis of stratigraphic relationships between moraines and tephrochronology, the earliest of these advances, the Crowfoot, was initially thought to be of early Holocene or late Wisconsinan age (CitationLuckman and Osborn, 1979). More recent analysis of lake sediment cores and moraine stratigraphy has suggested that this advance occurred during the Younger Dryas (Osborn and CitationLuckman, 1988; CitationReasoner et al., 1994). Neoglacial activity in the Canadian Rockies is thought to have begun with the Peyto advance at ca. 3000 14C BP (CitationLuckman et al., 1993; CitationLeonard and Reasoner, 1999), which was followed by early and later Little Ice Age advances beginning at ca. 800 14C BP and lasting until the beginning of the 20th century (CitationLuckman, 2000). Pollen and diatom studies from lakes in the same area suggest that treeline elevations were higher during the early Holocene and have decreased through the past 4000 yr, with cooler, wetter conditions becoming predominant (CitationMacDonald, 1982; CitationKearney and Luckman, 1983; CitationLuckman and Kearney, 1986; CitationHickman and Reasoner, 1998; CitationReasoner and Huber, 1999).
The paleoenvironmental chronology presented in this study combines sedimentological and palynological analysis of a lake sediment core with geomorphic reconstructions of past glacial advances. This approach provides a direct link between vegetation, sedimentary, and glacial responses to past climatic changes, allowing more detailed reconstruction. This work also provides an additional minimum age for the Crowfoot advance, strengthening previous evidence suggesting that the type section Crowfoot moraine is of late Pleistocene age and possibly correlative to the Younger Dryas (CitationReasoner et al., 1994). Paleo&ndash=uilibrium-line altitude (ELA) reconstructions provide an estimate for the magnitude of climatic change at the termination of the Crowfoot advance.
Regional Setting
The Burstall Valley is a tributary to the Spray River valley in Kananaskis Country, Alberta, approximately 100 km west of Calgary (). The hydrology of the valley is dominated by meltwater from the Robertson Glacier, which lies between 2500 and 2750 m a.s.l., on the north side of a col at the head of a deeply incised glacial valley southwest of Burstall Pass (). The ELA of the Robertson Glacier was estimated at 2600 m a.s.l., based on aerial photographs taken 3 October 1993. Downvalley 1.5 km from the modern glacier terminus, a pair of moraines record two post–Late Wisconsinan advances of the Robertson Glacier ( and ).
Lower Burstall Lake is fed by meltwater from the Robertson Glacier via two more proximal basins (upper and middle Burstall Lakes: ). Because the upper two lakes act as sediment traps, both the total influx and particle size of sediment entering Lower Burstall Lake are restricted, resulting in a relatively shallow sedimentary fill that is uninterrupted by density flows or other sedimentary events associated with environments having high sedimentation rates. Meltwater from the Robertson Glacier (via the upper two lakes) is the only major inflow to Lower Burstall Lake (). Lower Burstall Lake (1970 m a.s.l.) is currently below treeline (2450 m a.s.l.) and is surrounded by a mixed Picea englemanii and Abies lasiocarpa forest.
Methods
We took sediment cores from Lower Burstall Lake with a percussion coring system (CitationReasoner, 1993) in 8-m water depth, using the frozen lake surface as a platform. The core LBSL1 penetrated 290 cm into the lake bed before being stopped by dense, clay-rich sediment. The cores were frozen and cut in half longitudinally with a diamond rock saw. Moraines and drainage were mapped, both in the field and by analysis of aerial photographs.
Analysis of the lake sediment cores included total organic carbon (TOC) and total inorganic carbon (TIC) by loss-on-ignition (CitationBengtsson and Enull, 1986) as well as lithostratigraphic, macrofossil, and pollen analyses. Pollen samples were taken at 10-cm intervals, prepared using standard treatment (CitationFaegri and Iversen, 1989), and spiked with lycopodium spores, with an average of 300 grains counted per sample. Macrofossils and sand grains (>175 μm) were counted at 10-cm intervals by wet sieving 1-cm-thick slices of core (∼45 cm3). Raw counts were then normalized to 1 cm3. An accelerator mass spectrometer (AMS) radiocarbon date was obtained from a single conifer needle (Picea sp.) sieved from the center of the core at 242-cm depth. The Mazama (ca. 6730 14C BP) and Bridge River (ca. 2400 14C BP) tephras provided additional chronological control (CitationClague et al., 1995; CitationHallett et al., 1997), and allowed the creation of an age-depth model and calculation of sedimentation rates by linear interpolation (). Tephras in both the lake core and soil pit were identified on the basis of petrographic morphology according to the criteria suggested by CitationReasoner and Healy (1986), stratigraphic position where appropriate, and laboratory comparison to known reference samples of Mazama, Bridge River, and St. Helen's Y tephras. All dates are quoted in radiocarbon years BP (14C BP), and the thickness of the Mazama tephra was removed from the age-depth model (). Core zonation was done primarily on the basis of visual lithostratigraphy as well as the pollen, TOC, and TIC data ( and ).
Results
GEOMORPHOLOGY
The outermost of the two moraines downvalley from the Robertson Glacier (Moraine 1) is rounded in profile and well vegetated (), with both arboreal and nonarboreal species established on the crest. Mazama tephra was found near the base of a 20-cm soil profile () atop the crest of Moraine 1. The location of the tephra on the apex of the moraine precludes secondary deposition, thereby providing a minimum age for Moraine 1 of ca. 6730 14C BP. Although Mazama is the only tephra plume mapped as crossing this area, Bridge River tephra and St. Helen's Y tephra were identified in a section 15 km northeast of the Burstall Pass Area (CitationLimbird, 1984). Despite the presence of Bridge River tephra in the core from Lower Burstall Lake, neither it nor St. Helen's Y tephra were found on either moraine.
The proximal lateral moraine (Moraine 2) is sharp-crested and pushed into the flank of Moraine 1. Soil is mostly absent from the surface, with vegetation limited to scattered willows, and subalpine fir seedlings growing in small pockets of fine-grained sediment (). The flanks of Moraine 2 are oversteepened and incised with small rills and gullies. Small recessional ridges within the perimeter of Moraine 2, in the glacier forefield, are rounded, less than 2 m high in profile, and mostly devoid of vegetation. Because there are no other terminal moraines located inside these recessional features, we assumed that the Moraine 2 represents the most recent Holocene advance of the Robertson Glacier. Following this advance, the Robertson Glacier retreated approximately 1.5 km to its current position ().
LAKE CORE
The 290-cm-long sediment core obtained from Lower Burstall Lake contained three prominent lithostratigraphic zones, consisting of a basal inorganic silt and clay unit (Zone 1), an organic-rich marl and silt unit (Zone 2), and a transitional organic-rich to inorganic silt unit (Zones 3a and b, respectively) (). Analysis of LOI organic and inorganic carbon content, pollen, and macrofossils ( and ) indicate that zonation based on visual sedimentology matches well with geochemical and biological changes in the sediments.
Zone 1: 290–246 cm
Sedimentation in Zone 1 was dominated by massive to laminated sand, silt, and clay. Sieving Zone 1 revealed small concentrations of coarse sand in several intervals. TOC in Zone 1 was consistently below 5% (), and aquatic or terrestrial macrofossils were absent. Pollen was very sparse in Zone 1 sediments as compared to Zone 2, requiring as many as five slides (compared to less than one for Zone 2) to count ∼300 pollen grains. The pollen assemblage in Zone 1 was dominated by xeric herbaceous and shrub taxa, with very little arboreal representation ().
Zone 2: 246–160 cm
An abrupt, conformably bedded change to organic-rich marl at 246 cm marked the boundary between Zones 1 and 2, suggesting a rapid change in sedimentary regime. The marl, 4 cm thick, ended in a sharp transition to organic-rich silt. TOC in Zone 2 increased to 10% and remained above 5% throughout the zone (). Molluscs of the genus Pisidium were found in concentrations of 55 valves/100 cc within the marl but declined rapidly to 7 valves/100 cc within the first few centimeters of the organic-rich silt before disappearing altogether by the 225-cm interval. Also present within the marl were very high concentrations of charophyte oogonia. The AP/NAP ratio increased from less than 1 in Zone 1 to 3 at the base of Zone 2, with Pinus increasing to more than 60% relative abundance (). Alnus pollen decreased sharply across this boundary, as did herbaceous pollen and pollen from several other shrubs. A brief decrease in the AP/NAP ratio and a concentration of sub millimeter charcoal macrofossils at 230 cm corresponded to the sedimentary change from marl to organic-rich silt ( and ). An AMS radiocarbon date of 9180 ± 60 14C BP (CAMS-20358) was obtained from a Picea needle fragment found 4 cm above the Zone 1–2 boundary. The Mazama tephra (ca. 6730 14C BP: CitationHallett et al., 1997), between 210 and 218 cm, also provided chronological control for this zone (). The average sedimentation rate between the radiocarbon date and Mazama tephra was the lowest in the core at 0.9 mm yr–1.
Zone 3: 160–0 cm
The transition from Zone 2 to Zone 3 was gradational and characterized by decreased TOC and a sharp decline in the AP/NAP ratio. Sedimentation in Zone 3 was dominated by carbonate silt and clay, becoming less organic and more carbonate rich up core. Zone 3 contained no macrofossils and averaged less than 5% TOC throughout (). On the basis of TOC and AP/NAP ratios, Zone 3 was divided into two subzones, Zones 3a (160–90 cm) and 3b (90–0 cm) ( and ). The Bridge River tephra, dated at ca. 2400 14C BP, was found at the 146-cm interval of Zone 3a. Sedimentation rates in Zone 3 were 1.7 mm yr–1 below the Bridge River tephra and 6 mm yr–1 above it. The abrupt nature and precise timing of this change in sedimentation rate are a function of the location of the dating control point.
Zone 3a: 160–90 cm
Beginning at about 160 cm, Pinus pollen declined abruptly and was replaced by increasing amounts of Alnus, Salix, Shepherdia, and several herbaceous pollen taxa. These changes resulted in decreased AP/NAP ratios, with levels similar to those in Zone 1 at 120 cm (). LOI organic carbon declined steadily from the base of Zone 3a, stabilizing at ∼5% above the 120-cm interval. Carbonate values increased from the base of Zone 3a until 130 cm, reaching ∼20% at the transition to Zone 3b.
Zone 3b: 90–0 cm
At the base of Zone 3b, the AP/NAP ratio increased as Pinus pollen became more dominant and herb and shrub pollen, which dominated Zone 3a, declined significantly (). Following a peak at 80 cm, the AP/NAP ratio declined steadily with herb and shrub pollen becoming more dominant and Pinus pollen decreasing by 20%. Herbaceous pollen remained at increased levels until the 20 cm interval of the core, where the AP/NAP ratio increased, reflecting decreased importance of Alnus and herbaceous pollen taxa (). LOI organic carbon continued to decrease above 90 cm, reaching the lowest values of the Holocene in the upper 10 cm of the core. Carbonate increased steadily after 90 cm, reaching a Holocene maximum value of ∼25% in the topmost sample of the core.
Discussion
LATE PLEISTOCENE (>10,000 14C BP)
The presence of the Mazama tephra, which is found within the soil profile on the surface of Moraine 1, shows that Moraine 1 is older than ca. 6730 14C BP (). This date indicates that it is likely of similar age to a pre-Mazama aged moraine at the Crowfoot Glacier, identified by CitationLuckman and Osborn (1979) and named the Crowfoot advance. Because the only period of clastic sedimentation stratigraphically below the Mazama tephra in the core from Lower Burstall Lake occurs in Zone 1, below 246-cm depth, the radiocarbon date at 242-cm depth provides a minimum age for the advance of 9180 ± 60 14C BP (Zone 1: ). Because this date comes from 4 cm above the onset of organic sedimentation, it underestimates the age of the basal clastic sediments, likely by several hundred years. On this basis, it is probable that the transition from clastic to organic sediments in Lower Burstall Lake is correlative to similar transitions in numerous lakes in the Southern Canadian Rocky Mountains that occurred at ca. 10,000 14C BP (e.g., CitationReasoner and Hickman, 1989; CitationBeierle, 1997; CitationBeierle and Smith, 1998; CitationReasoner and Huber, 1999).
The age of this basal inorganic sediment package is also similar to basal inorganic sediments from Crowfoot Lake that were deposited between 10,100 14C BP and 11,300 14C BP and have been correlated to the Younger Dryas cold period (CitationReasoner et al., 1994). The dominantly herbaceous pollen assemblage in Zone 1 supports this inference and suggests that cold, dry conditions prevailed during this interval. Although recent work has cast some doubt on the validity of the correlation between the Crowfoot type section moraine and the basal sediments from Crowfoot Lake (CitationLeonard, 1998), the apparent synchrony of the Crowfoot event in the Rocky Mountains (this paper; CitationReasoner et al., 1994; CitationReasoner and Jodry, 2000) and the Younger Dryas in the Greenland ice cores (CitationAlley, 2000) indicates that the two may represent the same event. Evidence for a significant Younger Dryas climatic event in western North America is also supported by recent work from both New Mexico (CitationArmour et al., 2002) and British Columbia (CitationKovanen and Easterbrook, 2002) which also suggest the occurrence of major glacial advances during the late Pleistocene.
EARLY TO MIDDLE HOLOCENE (CA. 10,000 14C BP–3500 14C BP)
Clastic sediment flux decreased abruptly in Lower Burstall Lake at ca. 10,000 14C BP and remained low until ca. 3500 14C BP (Zone 2: ). Clastic sediment was replaced by organic-rich silt and marl during this interval, suggesting a major change in depositional environment. Marl deposition in this and other glacier-fed lakes in the southern Canadian Rocky Mountains between ca. 10,000 and 9000 14C BP (e.g., CitationReasoner et al., 1994; CitationBeierle, 1997) suggests that lake water was warmer and may have been thermally unstratified, because marl accumulated on the floor of the lake, rather than redissolving at depth due to increased acidity often associated with anoxic bottom conditions and stratified lake waters. Higher rates of algal photosynthesis inferred by increased LOI organic carbon levels suggest decreased water turbidity during the early Holocene, as does the presence of gill-breathing benthic molluscs (). Gill-breathing benthic fauna also show that oxic conditions likely prevailed in bottom waters during this interval. Marl deposition terminated abruptly at ca. 9000 14C BP, after which molluscs also disappeared from the sedimentary record. This change may indicate seasonal stratification of lake waters, with anoxic conditions below the thermocline resulting in redissolution of carbonate solids and the extinction of some benthic fauna. Continued organic sedimentation above the marl suggests that turbidity and clastic sediment influx remained low until ca. 3500 14C BP. Increased arboreal pollen percentages, particularly Pinus (), may indicate that treeline was higher during the early Holocene, probably as a result of a warmer climate. Many other records from this region also indicate that treeline was higher than at present during the early Holocene (e.g., CitationKearney and Luckman, 1983; CitationLuckman and Kearney, 1986; CitationReasoner and Hickman, 1989), although this record is unique in that there are several dramatic drops in the AP/NAP ratio in Zone 2, which may indicate the establishment of more open valley-bottom forests as treeline moved to even higher elevations. This interpretation is corroborated by increased abundances of xerophytic pollen taxa during these intervals at ca. 8000 14C BP and between 5000 and 6000 14C BP.
Increased water temperature and decreased water column turbidity suggest that glacial influence in Lower Burstall Lake was substantially decreased during the early Holocene. Similar early Holocene records from Crowfoot Lake, Upper Kananaskis Lake, Lake Louise, Lake O'Hara, and Bow Lake (CitationReasoner and Hickman, 1989; CitationReasoner et al., 1994; CitationBeierle, 1997; CitationBeierle and Smith, 1998; CitationLeonard and Reasoner, 1999) also contain evidence for significantly decreased glacial sediment input, with the presence of organic sediments, marl, and molluscs suggesting that glacial ice may have completely disappeared in some locations. Sediments from Hector Lake also suggest decreased glacial activity but indicate that the Waputik Ice Field, although reduced in area, continued to exist through the early Holocene (CitationLeonard and Reasoner, 1999). This may have been the case because the Waputik Ice Field is one of the highest glaciers in this area of the Canadian Rockies, at 3240 m a.s.l. The regional picture of decreased glacierization is also supported by detrital wood dating to the early Holocene discovered at the terminus of the Athabasca and Dome Glaciers (CitationLuckman, 1988; CitationLuckman et al., 1993), indicating that that these glaciers were substantially less extensive during the early Holocene.
LATE HOLOCENE (CA. 3500 14C BP TO PRESENT)
Whereas the Zone 1–2 boundary is sharp, the sedimentological change from Zone 2 to Zone 3 is gradual (). LOI organic carbon values began to decline at ca. 3500 14C BP and decreased steadily through the late Holocene. AP/NAP values dropped rapidly just prior to ca. 3500 14C BP and continued to decline through ca. 2000 14C BP, remaining at almost pre-Holocene levels until ca. 1300 14C BP (). Zone 3a likely represents the onset of the Neoglacial, with decreased AP/NAP values suggesting either reduced treeline elevations or disruption of local forest communities by reactivation of the Robertson Glacier outwash plain, which occupies much of the valley bottom area upstream of Lower Burstall Lake (). Either of these hypotheses is consistent with decreased LOI organic carbon, increased LOI carbonate values, and increased sedimentation rates, which suggest renewed glacial activity in the catchment ().
AP/NAP values remained low until ca. 1300 14C BP, when increased arboreal pollen percentages suggest that spruce and pine increased in abundance (Zone 3b: ). This interval had the highest arboreal pollen content of the core, suggesting improved growing conditions over those in Zone 3a (). After this initial increase, arboreal pollen again declined in importance, although more slowly this time, reaching a minimum at ca. 400 14C BP (). After ca. 400 14C BP, the AP/NAP ratio increased until ca. 200 14C BP before declining into modern times, likely in response to renewed glacial activity during the Little Ice Age (). Although it is uncertain whether these changes in AP/NAP ratio indicate movement of treeline or changes in local vegetation dynamics, periods with decreased arboreal pollen abundances during the last 1300 yr may correspond with Neoglacial and Little Ice Age advances proposed by CitationLuckman et al. (1993), CitationSmith et al. (1995), and CitationLuckman (2000), although the chronology through this portion of the core lacks sufficient resolution for these correlations to be more than speculative.
Because LOI carbon and carbonate remained stable through the late Holocene, we suggest that the lacustrine sedimentary record in Lower Burstall Lake is not as sensitive to small-scale fluctuations in climate as the pollen record ( and ). Increased clastic flux to the basin after ca. 3500 14C BP is likely indicative of renewed glacierization in a general sense, as opposed to recording specific glacial events. Thus, the multiple advances thought to have occurred during the Neoglacial were likely blended together within the clastic sedimentary record.
Despite the minimal resolution in the lacustrine sedimentary record during the late Holocene, the lack of smaller terminal moraines within Moraine 2 at the Robertson Glacier suggest that it was most likely produced during the Little Ice Age. Although this moraine is undated, its morphology and size are consistent with known Little Ice Age moraines in the area. This moraine is sharp-crested, with oversteepened flanks, similar to the Little Ice Age moraine described by CitationSmith et al. (1995) at the adjacent French Glacier. In addition, the lack of turf or large trees makes it likely that Moraine 2 is relatively recent compared to older Neoglacial moraines at the nearby French Glacier that have been dated to ca. 500 14C BP (CitationSmith et al., 1995) and at other locations in the Southern Canadian Rockies (CitationLuckman and Osborn, 1979). Because the small ridges in the forefield of the Robertson Glacier are poorly formed and lack the characteristic sharp crest common in terminal moraines, they are interpreted as recessional features deposited during ice recession following the advance responsible for depositing the inner moraine (Moraine 2). CitationMcCarthy and Smith (1994) used historical photographs to document the retreat of many glaciers in the Southern Canadian Rocky Mountains, including the Robertson, and showed that it has retreated more than 1.2 km since 1916, losing 35% of its area.
Conclusion
We suggest that Holocene climatic and landscape evolution in the Burstall Pass area occurred in three stages, beginning with an advance of the Robertson Glacier prior to ca. 10,000 14C BP, which is correlated to the Crowfoot Advance and may represent cooling during the Younger Dryas. This advance deposited lateral and end moraines about 1.5 km downvalley from the modern glacier terminus correlating to an ELA depression of ∼300 m relative to the current ELA. The magnitude of the advances depositing Moraines 1 and 2 was almost equal, suggesting either that the ELA depression for both the Younger Dryas and Little Ice Age may have been similar in this region or that the glacier may not have had time to equilibrate to changes in ELA during one or both advances.
Following this advance, the termination of clastic sediment flux to Lower Burstall Lake suggests that abrupt climatic warming at ca. 10,000 14C BP resulted in rapid retreat of the Robertson Glacier, and possibly its complete ablation. Treeline may also have been higher after ca. 10,000 14C BP, and reconstructions of paleo-ELA using the 70% area method (CitationBradley, 1999) indicate that the ELA of the Robertson Glacier may have risen by as much as 440 m at ca. 10,000 14C BP.
This period of limited glacial activity lasted until ca. 3500 14C BP, when arboreal pollen levels suggest that treeline elevation began to decrease and declining LOI organic carbon values suggest declining lake productivity and increased glacial sediment flux. Palynological analysis indicates that the period between 3500 and 1300 14C BP was colder, with renewed glacial activity resulting in increased clastic sediment flux to the lake. Although the clastic sediment record from Lower Burstall Lake cannot resolve multiple Neoglacial advances, fluctuations in arboreal pollen levels through the last 2000 radiocarbon yr may correlate to Late Holocene glacial events noted by CitationLuckman and Osborn (1979) and CitationSmith et al. (1995). Warmer climate after ca. 1300 14C BP is suggested by increased representation of arboreal pollen, although the almost steady decline in LOI organic carbon and steady increase in LOI carbonate after ca. 3500 14C BP suggest that climatic fluctuations after this time were also too subtle to be reflected in the bulk physical properties of the sediment. The younger moraine at the Robertson Glacier is most likely associated with the last advance of the Little Ice Age during the mid- to late 19th century, but it remains undated.
FIGURE 1. Location of Burstall Pass/Lower Burstall Lake area (1.) and locations of other lakes mentioned in text (2. Upper Kananaskis Lake, 3. Lake O'Hara, 4. Lake Louise, 5. Hector Lake, 6. Crowfoot Lake, 7. Bow Lake)
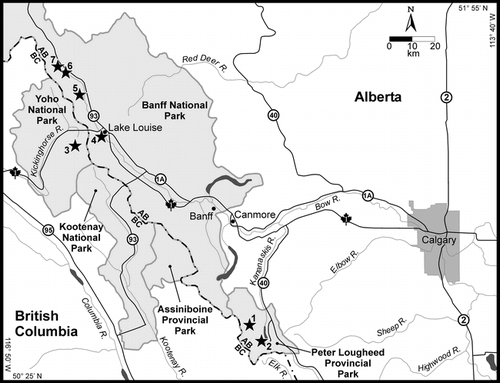
FIGURE 2. Contour map of the Burstall Pass area showing the Robertson Glacier, lateral and terminal moraines, and the locations of the soil pit in Moraine 1 and the coring site in Lower Burstall Lake
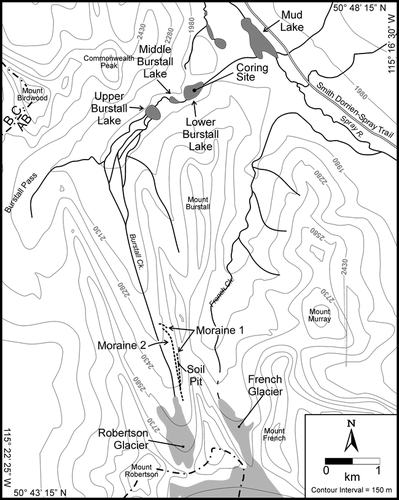
FIGURE 3. (a) The paired lateral moraines downvalley from Robertson Glacier showing the difference in vegetation cover between the older Moraine 1 (left) and younger Moraine 2. (b) Soil pit in older (left) moraine
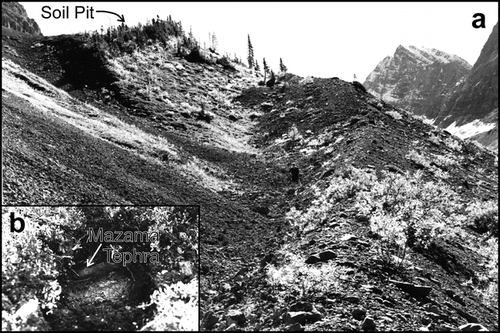
FIGURE 4. Age depth curve based on linear interpolation between points of known age in the core LBSL2 from Lower Burstall Lake. Note that the thickness of the Mazama tephra was removed from the calculations
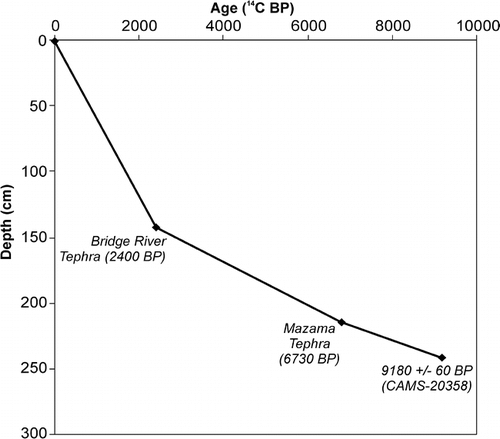
Acknowledgments
The authors would like to thank David Mazzucchi and Natalie Veldhoen for their assistance in the field. Mel Reasoner is also thanked for his field assistance and for providing the radiocarbon date. Laboratory facilities were provided by the Departments of Geography, The University of Calgary, and Queen's University. Julian Szeicz provided laboratory space, equipment, and assistance with the pollen analysis. Markus Heinrichs, Robert Gilbert, Scott Lamoureux, Eric Leonard, Mel Reasoner, and an anonymous reviewer provided valuable critical reviews of this manuscript.
References Cited
- Alley, R. B. 2000. The Younger Dryas cold interval as viewed from central Greenland: past global changes and their significance for the future. Quaternary Science Reviews 19:213–226.
- Armour, J. , P. J. Fawcett , and J. W. Geissman . 2002. 15 k.y. paleoclimatic and glacial record from northern New Mexico. Geology 30:723–726.
- Beierle, B. D. 1997. Early Holocene climate of southwestern Alberta, Canada, reconstructed from lake sediment cores. Master's thesis, Department of Geography, The University of Calgary, Calgary, Alberta, Canada. 115 pp.
- Beierle, B. and D. G. Smith . 1998. Severe drought in the early Holocene (10,000–6800 BP) interpreted from lake sediment cores, southwestern Alberta, Canada. Palaeogeography, Palaeoclimatology, Palaeoecology 140:75–83.
- Bengtsson, L. and M. Enull . 1986. Chemical Analysis. In Berglund, B. E. (ed.), Handbook of Holocene Palaeoecology and Palaeohydrology. Chichester: John Wiley and Sons Ltd., 423–451.
- Bradley, R. S. 1999. Paleoclimatology, 2nd ed. San Diego: Academic Press. 613 pp.
- Clague, J. J. , S. G. Evans , V. N. Rampton , and G. J. Woodsworth . 1995. Improved age estimates for the White River and Bridge River tephras, western Canada. Canadian Journal of Earth Sciences 32:1172–1179.
- Faegri, K. and J. Iversen . 1989. Textbook of Pollen Analysis, 4th ed. Chichester: John Wiley and Sons. 328 pp.
- Hallett, D. J. , L. V. Hills , and J. J. Clague . 1997. New accelerator mass spectrometry radiocarbon ages for the Mazama tephra layer from Kootenay National Park, British Columbia, Canada. Canadian Journal of Earth Sciences 34:1202–1209.
- Hickman, M. and M. A. Reasoner . 1998. Late Quaternary diatom response to vegetation and climate change in a subalpine lake in Banff National Park, Alberta. Journal of Paleolimnology 20:253–265.
- Kearney, M. S. and B. H. Luckman . 1983. Postglacial vegetational history of Tonquin Pass, British Columbia. Canadian Journal of Earth Sciences 20:776–786.
- Kovanen, D. J. and D. J. Easterbrook . 2002. Timing and extent of Allerød and Younger Dryas Age (ca. 12,500–10,000 14C YR B.P.) Oscillations of the cordilleran Ice Sheet in the Fraser Lowland, western North America. Quaternary Research: 57:208–224.
- Leonard, E. M. 1998. Is the type-Crowfoot Moraine, Alberta, really a Younger Dryas equivalent?. Geological Society of America, 1998 Annual Meeting 30:66.
- Leonard, E. M. and M. A. Reasoner . 1999. A continuous Holocene glacial record inferred from proglacial lake sediments in Banff National Park, Alberta, Canada. Quaternary Research 51:1–13.
- Limbird, A. 1984. Genesis of soils affected by discrete volcanic ash inclusions, Alberta, Canada. Catena, Selected Papers of the Congresso Internacional de Seulos Volcanicos: 119–130.
- Luckman, B. H. 1988. 8000 year old wood from the Athabasca Glacier, Alberta. Canadian Journal of Earth Sciences 25:148–151.
- Luckman, B. H. 2000. The Little Ice Age in the Canadian Rockies. Geomorphology 32:357–384.
- Luckman, B. H. and M. S. Kearney . 1986. Reconstruction of Holocene changes in alpine vegetation and climate in the Maligne Range, Jasper National Park, Alberta. Quaternary Research 26:244–261.
- Luckman, B. H. and G. D. Osborn . 1979. Holocene glacier fluctuations in the middle Canadian Rocky Mountains. Quaternary Research 11:52–77.
- Luckman, B. H. , G. Holdsworth , and G. D. Osborn . 1993. Neoglacial glacier fluctuations in the Canadian Rockies. Quaternary Research 39:144–153.
- MacDonald, G. M. 1982. Late Quaternary paleoenvironments of the Morley Flats and Kananaskis Valley of southwestern Alberta. Canadian Journal of Earth Sciences 19:23–25.
- McCarthy, D. P. and D. J. Smith . 1994. Historical glacier activity in the vicinity of Peter Lougheed Provincial Park, Canadian Rocky Mountains. Western Geography 4:94–109.
- Osborn, G. D. and B. H. Luckman . 1988. Holocene glacier fluctuations in the Canadian Cordillera (Alberta and British Columbia). Quaternary Science Reviews 7:115–128.
- Reasoner, M. A. and R. E. Healy . 1986. Identification and significance of tephras encountered in a core from Mary Lake, Yoho National Park, British Columbia. Canadian Journal of Earth Sciences 23:1991–1999.
- Reasoner, M. A. 1993. Equipment and procedure improvements for a lightweight, inexpensive, percussion core sampling system. Journal of Paleolimnology 8:273–281.
- Reasoner, M. A. and M. Hickman . 1989. Late Quaternary environmental change in the Lake O'Hara region, Yoho National Park, British Columbia. Palaeogeography, Palaeoclimatology, Palaeoecology 72:291–316.
- Reasoner, M. A. and U. M. Huber . 1999. Postglacial palaeoenvironments of the upper Bow Valley, Banff National Park, Alberta, Canada. Quaternary Science Reviews 18:475–492.
- Reasoner, M. A. and M. A. Jodry . 2000. Rapid response of alpine timberline vegetation to the Younger Dryas climate oscillation in the Colorado Rocky Mountains, U.S.A. Geology 28:51–54.
- Reasoner, M. A. , G. D. Osborn , and N. W. Rutter . 1994. Age of the Crowfoot advance in the Canadian Rocky Mountains: a glacial event coeval with the Younger Dryas oscillation. Geology 22:439–442.
- Smith, D. J. , D. P. McCarthy , and M. E. Colenutt . 1995. Little Ice Age glacial activity in Peter Lougheed and Elk Lakes provincial parks, Canadian Rocky Mountains. Canadian Journal of Earth Sciences 32:579–589.