Abstract
Glacial deposits are identified from within and near Sinks Canyon, southwest of Lander, Wyoming, representing 6 first-order Pleistocene glaciations. Relative-age analyses of the deposits (including moraine morphology and soil development characteristics) indicate that they correspond to 4 glaciations previously identified from the Wind River Range (Pinedale, Early Wisconsin, Bull Lake, and Sacagawea Ridge) and to 2 older glacial events (Younger and Older Pre–Sacagawea Ridge). 10Be and 26Al exposure ages associated with recessional Pinedale deposits are similar to those associated with recessional Pinedale deposits elsewhere in the range. 10Be and 26Al exposure ages also support the identification of Early Wisconsin deposits here. The Early Wisconsin deposits represent the second locality where O-isotope stage 4 glacial deposits are described from the Wind River Range. Preliminary analysis of 10Be exposure data from the Older Pre–Sacagawea Ridge deposit suggests that a glacial advance occurred here before O-isotope stage 18 (>800 ka). If true, then Sinks Canyon contains the most complete record to date of glaciation in the Wind River Range and the only reported record of Pleistocene glaciation prior to O-isotope stage 18 in the Rocky Mountains.
Introduction
No single locality contains a complete record of the Pleistocene glacial history of the Rocky Mountain region of the United States. Until recently, however, it was thought that 5 first-order Pleistocene glaciations were represented by surface moraines, buried moraines, and lake deposits at the Cedar Ridge section above the north shore of Bull Lake on the northeastern flank of the Wind River Range of Wyoming and that these deposits represented most of Pleistocene time (CitationRichmond, 1964, Citation1986a). The deposits at Cedar Ridge purportedly represented (from youngest to oldest) the Pinedale, Bull Lake, Sacagawea Ridge, Cedar Ridge, and Washakie Point glaciations (CitationBlackwelder, 1915; CitationRichmond 1964, Citation1965, Citation1983, Citation1986a, Citation1986b; CitationRichmond and Murphy, 1989; CitationHall and Shroba, 1995). Recent work by CitationHall and Jaworowski (1999) suggests that the Cedar Ridge and Washakie Point deposits were misidentified at Bull Lake and that deposits here represent glacial activity that is no older than Sacagawea Ridge (∼600–770 ka). Thus, only Pinedale, Early Wisconsin, Bull Lake, and Sacagawea Ridge deposits are presently identified from the Wind River Range.
This study reports evidence of as many as 6 glaciations in a canyon ∼55 km south of Bull Lake on the northeastern flank of the Wind River Range. The study describes a series of inner canyon-to-piedmont glacial deposits identified from Sinks Canyon, southwest of Lander, Wyoming (). The main stratigraphic issues addressed by this study include (1) the number of glaciations documented by deposits here, (2) correlation with previously identified glaciations in the Wind River Range, and (3) identification of glacial deposits in or near Sinks Canyon that are related to older glacial events than those presently identified in the range.
Relative-age data (RA) indicate that 6 morphostratigraphic units are present in the canyon; each of these units are interpreted to represent a Pleistocene glacial advance. A limited amount of numeric-age data supports the presence of Pinedale, Early Wisconsin, Bull Lake, Sacagawea Ridge, and Pre–Sacagawea Ridge glacial deposits. Based on the data presented here, Sinks Canyon is the second locality in the Wind River Range from which Early Wisconsin deposits are identified. The oldest 2 surficial units described here are Pre–Sacagawea Ridge deposits previously not described in the Wind River Range. These deposits may are the only reported surficial record of Pleistocene glaciation prior to O-isotope stage 18 reported in the U.S. Rocky Mountains.
Study Area and Methods
Sinks Canyon is a 19-km2 area 15 km southwest of Lander, Wyoming, near the southern flank of the Wind River Range (). The canyon is the most southerly of 4 canyons along the northeastern slope of the Wind River Range where piedmont glacial deposits have been described (CitationMurphy and Richmond, 1965; CitationRichmond and Murphy, 1965, Citation1989; CitationRichmond, 1986a; CitationShroba, 1989; CitationChadwick et al., 1997; CitationPhillips et al., 1997; CitationApplegarth and Dahms, 2001). Sinks Canyon was the single outlet for glaciers that accumulated in the Middle Popo Agie (Po-po′-zhuh) basin in the southeastern Wind River Range. Sinks Canyon is the only one of the four of which the glacial sequence consists predominantly of inner-canyon deposits.
Sinks Canyon is carved into a nearly complete section of the Paleozoic limestones, dolomites, and sandstones described for this region of Wyoming (CitationLove et al., 1992). The upper one-fourth of the canyon is in Precambrian granite of the Louis Lake Formation (CitationFrost et al., 2000), whereas the walls of the lower canyon are Paleozoic limestone, dolomite, and sandstone. The glacial deposits described here (, ) generally are within the canyon walls. Only the oldest 2 deposits are identified on or beyond the canyon rims. The lithology of most of the Sinks Canyon deposits differs from that previously reported for northeastern flank localities because the tills above the canyon's mouth contain no primary carbonate.
Elevation of the Middle Popo Agie River ranges from 2500 m (8200 ft) a.s.l. near the western (upstream) edge of the study area to 1824 m (5980 ft) at the mouth of Sinks Canyon where the Middle Popo Agie river enters the Lander Valley (, ). The highest and lowest elevations for units mapped in this study are 2800 m (9184 ft) and 1751 m (5760 ft), respectively. Mean January and July temperatures at Lander (1636 m a.s.l.) are −7° and 22°C, respectively. Mean annual precipitation (MAP) at Lander is 30.7 cm; most of this is spring snowmelt and early-summer thunderstorms. In general, temperatures are lower and precipitation is higher with increasing elevation in Sinks Canyon due to orographic effects. MAP is estimated to vary within the canyon from ∼38 cm at the mouth to ∼64 cm near the west edge of the study area at Popo Agie Falls (R.W. Scott, personal communication, 2002).
The map units described here were identified from a combination of walking unit boundaries and mapping each unit's geomorphic characteristics and position relative to adjacent units. Unit contacts were placed on topographic maps. Boulder weathering data and moraine morphology were described at locations that were representative of the overall morphology of each map unit. Soil pits then were excavated at locations where there was good likelihood that intact, representative profiles might be exposed.
RELATIVE-AGE METHODS
Relative-age (RA) methods historically are the primary data used to differentiate glacial deposits in the Wind River Range (e.g., CitationBlackwelder, 1915; CitationRichmond, 1986a). Few numeric ages were available prior to 1995 for the Late Pleistocene deposits of the Wind River Range. The most critical of these numeric ages are summarized elsewhere (CitationGosse et al., 1995a, Citation1995b; CitationChadwick et al., 1997; CitationPhillips et al., 1997; CitationHall and Jaworowski, 1999; CitationDahms, 2004). I relied on the RA characteristics used by previous studies in the Wind River Range, as few numeric ages were available to this study (e.g., CitationRichmond 1965, Citation1976, Citation1986a, Citation1987; CitationShroba and Birkeland, 1983; CitationDahms, 1991, Citation1994; CitationHall and Shroba, 1995; CitationHall, 1999; CitationApplegarth and Dahms, 2001).
Moraine Morphology and Boulder Weathering Characteristics
Previous descriptions of Pleistocene glacial deposits have shown that surface expression(s) of moraines change and boulders weather in a more or less predictable way with time (e.g., CitationBlackwelder, 1915; CitationRichmond, 1973, Citation1986a, Citation1987). Boulder weathering varies widely because of fire-induced spalling and variations in granular disintegration and erosion here. Boulders also become partly buried by loess in places (CitationRichmond, 1987; CitationShroba and Birkeland, 1983). Boulder weathering characteristics used in this study include () amount of polish and preservation of striae, maximum weathering pit depths (>100 boulders of >1-m diameter), % split boulders, and the estimated surface boulder frequency (boulders per unit area).
Soil Development
Soils are established tools for estimating the relative ages of Quaternary deposits and their associated landforms (e.g., CitationBirkeland, 1999; CitationBirkeland et al., 1991). Soils data generally are difficult to use without other RA data because soil characteristics commonly vary widely across the landscape. Thus, it is often difficult to use soil development data alone to constrain the ages of the morphostratigraphic units in this area because soils are often under both forest and sagebrush/grass cover and tills often contain material derived from bedrock of different lithology (CitationHall, 1983). Soils data generally must be combined with other independent information (such as the moraine morphology and boulder weathering data) to create a more useful (although generalized) representation of local or regional age relations.
Soils were described and sampled from hand-dug pits using standard methods and horizon nomenclature (CitationBirkeland et al., 1991; Soil CitationSurvey Staff, 1994, Citation1999; CitationBirkeland, 1999). Bw and Cox horizons were distinguished either by texture (usually sandy loam versus loamy sand) and/or differences in Munsell color; Bw horizons have a 10YR hue and Cox horizons have a 2.5Y hue. The designation Btj (CitationNational Soil Survey Committee of Canada, 1974) is used here for horizons that contain translocated clay in insufficient amounts to meet minimal requirements for argillic (Soil CitationSurvey Staff, 1999).
Laboratory analyses used standard techniques modified from CitationJackson (1969), CitationKlute (1986), and CitationSinger and Janitzky (1986). Particle-size distribution was determined by the sieve and pipette method with prior oxidation of organic matter by 30% H2O2. Pedogenic carbonate and exchangeable divalent cations were removed by dissolution with 0.5N HCL on heat (∼80°C). Particle-size distribution is reported as weight percent (USDA grain-size scale). Bulk density was determined by the paraffin-clod method for gravel-free peds using an estimated gravel density of 2.6 g/cm−3. Bulk density values were obtained from the average of at least 2 peds where the soil matrix was sufficiently cohesive. When cohesive peds were not available, bulk density estimates were obtained from the mean values of similar soil horizons in Sinks Canyon or from other Wind River Range locales (CitationDahms, 1991, Citation1993, Citation1994, 2004; CitationApplegarth and Dahms, 2001).
Simple calculations of soil characteristics were used to portray the range of soil development on the glacial deposits in Sinks Canyon. Soil characteristics used for RA correlations and age assignments include horizonation, solum thickness, depth to Cox/C boundary, clay percentage data (accumulation index [AI], maximum clay in B horizon, profile weighted mean clay [PWM]), pedogenic clay, rubification and texture indices, and profile development index (PDI) ( and ).
Solum thickness and the depth to the Cox/C boundary measure the progressive weathering of the deposits as soils develop over time. Solum thickness measures the thickness of the A + B horizons, whereas depth to the Cox/C boundary includes the oxidized portion of the upper C horizon in many soils of the region (CitationShroba and Birkeland, 1983; CitationBirkeland, 1999), so this number is usually the larger of the two.
Clay accumulation data calculated for Sinks Canyon soils include the maximum percent clay in the B horizons, accumulation index (AI), the profile weighted mean (PWM), and the total accumulation of clay (here called pedogenic clay). The AI and the PWM were calculated using the methods described by CitationHall and Shroba (1995). Pedogenic clay is the sum of clay (both translocated and authigenic) that has accumulated in all horizons of each soil (profile g cm−2). The calculation follows the method described in CitationMcCalpin and Berry (1996):
where h is horizon value, BD is gravel-free bulk density, T is horizon thickness, pm is parent material value, and G is gravel (Taylor, 1986); vol%Gh = (wt.%Gh/δG)/[(wt.%Gh/δGh) + {(1 − wt.%Gh)/BDh}], where δ is density (CitationSoil Conservation Service, 1972). I attribute much of the clay in the soils of Pinedale and Bull Lake deposits and in A horizons of soils in all deposits to additions of aeolian dust (CitationBirkeland et al., 1991; CitationDahms, 1993; CitationDahms and Rawlins, 1996), so that relatively little clay in late Pleistocene profiles is due to in situ weathering. I consider clay derived from aeolian dust to be pedogenic, since dust-derived fines generally are regarded to have a considerable influence on soil genesis (e.g., CitationMuhs, 1983; CitationMcFadden and Weldon, 1987; CitationMcFadden, 1988; CitationReheis and Kihl, 1995; CitationReheis et al., 1995; CitationHarden et al., 1991; CitationMcCalpin and Berry, 1996). Clay contents of C horizons were used to estimate parent material values used to calculate values for AI, PWM, and pedogenic clay.
Rubification and texture indices are quantitative measurements of redness and textural changes in a soil profile relative to its C horizon. Procedures here follow those of CitationHall and Shroba (1993, Citation1995) and CitationHall (1999). The profile development index (PDI) converts soil field properties into numerical values by which soil development might be compared among stratigraphic units (CitationHarden, 1982; CitationBirkeland et al., 1991). Properties used here to calculate the PDI include moist and dry rubification, moist and dry melanization, total texture, wet/moist/dry consistence, structure, and clay films. Procedures for calculating Accumulation Index, PWM clay, and rubification and texture indices follow those of CitationHall (1999) and CitationHall and Shroba (1995). Index calculations for PDI followed those of CitationHarden (1982) and CitationBirkeland et al. (1991).
Glaciation of Sinks Canyon
The glacial succession described in Sinks Canyon generally corresponds to that previously described in the Wind River Range (CitationBlackwelder, 1915; CitationRichmond, 1976, Citation1986a, CitationHall and Jaworowski, 1999; CitationDahms, 2004). Additionally, I describe deposits of 2 older glacial events that currently have no recognized correlatives in the Rocky Mountain region. The field data are summarized in . From youngest to oldest, I identify these deposits as Morphostratigraphic units 1–6.
STRATIGRAPHY OF THE MAP UNITS
Morphostratigraphic Unit 1
Characteristics of unit 1 deposits correspond to those of deposits assigned to the Pinedale glaciation elsewhere in the Wind River Range. A complex of 5 terminal-recessional-lateral moraines lies along the upper 9 km of Sinks Canyon (). This valley-floor complex extends from a terminal moraine at 2037 m (6700 ft) directly upvalley from the Sinks to recessional moraine unit P5 at 2462 m (8100 ft), about 1 km upvalley from Popo Agie Falls. End moraines here are steep and sharp-crested. Kettles are not common on unit 1 moraines in Sinks Canyon, probably because of the limited ice volume and limited till thickness.
Lateral moraines of unit 1 are steeper and have sharper crests than the end moraines. Because the lateral moraines were deposited against steep valley walls, they have undergone more intense erosion and mass wasting than the end moraines. The lateral moraines of unit 1 extend along the valley walls from the Pinedale 1 terminal/end moraines at ca. 2037 m (6700 ft) near the Sinks to 2736 m (9000 ft), northwest of Popo Agie Falls (). They are closely inset within (and in places overlap) older deposits mapped here as morphostratigraphic units 2 and 3.
Boulders on moraines of unit 1 are relatively unweathered and unpitted except where boulders from older till have been incorporated locally into the till of unit 1. Granite boulders show no evidence of weathering to grus. Boulders often exhibit polished and faceted surfaces and little burial by loess. Boulders exhibit only slight pitting, surface boulder frequencies are high, and the number of split boulders is low ().
Morphostratigraphic Unit 2
Characteristics of unit 2 deposits correspond to those assigned to Early Wisconsin deposits in the Rocky Mountains (CitationColman and Pierce, 1986; CitationHall and Shroba, 1995). Moraines assigned to unit 2 are identified at 3 locations in Sinks Canyon. Two lateral moraines are located on the north and south walls of the canyon above the Missouri geology camp (). The crests of both moraines have elevations of approximately 2220 m (7300 ft). A third Early Wisconsin deposit is identified adjacent to and downvalley from the Pinedale terminal moraine (P1) on the north side of the Middle Popo Agie River.
The Early Wisconsin moraines exhibit RA characteristics similar to the Pinedale moraines. Boulders on Early Wisconsin moraines are nearly as unweathered and unpitted as those on the Pinedale moraines. Boulders on Early Wisconsin moraines show little postdepositional burial by loess. Boulders exhibit only slight pitting, surface boulder frequencies are relatively high, and the number of split boulders is low (). Buried small-diameter granite boulders show only slight weathering to grus.
Morphostratigraphic Unit 3
Unit 3 deposits in Sinks Canyon are correlated with deposits identified as Bull Lake elsewhere in the Wind River Range (CitationRichmond and Murphy, 1965). Bull Lake moraines in Sinks Canyon exhibit more subdued morainal topography than the Early Wisconsin and Pinedale moraines. Bull Lake deposits are above (on the valley wall) or downvalley from the Pinedale and Early Wisconsin moraines (). The highest Bull Lake deposits are identified on the north and south canyon walls at 2797 m (9200 ft) and 2368 m (7790 ft), respectively. Two adjacent lateral moraines are mapped at each of these localities. The innermost Bull Lake lateral moraines locally are partially buried by the Early Wisconsin and Pinedale units. In this area of the canyon, the Early Wisconsin and Pinedale deposits may have incorporated Bull Lake–age material, because Early Wisconsin and Pinedale soils have slightly redder hues and contain slightly more clay than elsewhere. A Bull Lake deposit is identified further downvalley on the south canyon wall where it is preserved between several talus deposits. Bull Lake deposits are not exposed on the north wall of the canyon downvalley from the Missouri geology camp.
Deposits that represent the downvalley limit of Bull Lake ice are mapped just beyond the mouth of Sinks Canyon. Bull Lake ice apparently advanced only slightly beyond the mouth of the canyon where it stagnated and deposited a mixture of till and outwash. This material is mapped from 1885 m (6200 ft) to 1763 m (5800 ft) on both sides of the Middle Popo Agie River. These deposits exhibit subdued knob-and-kettle topography, but the kettle sides have been breached (or the kettles filled) and drainages between depressions are integrated, so the original topography is now obscured. These stagnant-ice deposits are inset into similar material deposited by the Sacagawea Ridge ice advance.
Boulders are more weathered on Bull Lake deposits than on the Early Wisconsin and Pinedale deposits. Boulders are often imbedded in a 50-cm-thick deposit of loess and in till that is characteristic of Bull Lake moraines in this region (CitationRichmond, 1986a; CitationShroba and Birkeland, 1983). Surface boulder frequencies are lower and split boulders are more common than on Pinedale and Early Wisconsin moraines (). Boulders on lateral moraines along the south canyon wall are not as deeply buried because erosion maintains very steep slopes inside the canyon. Pits are deeper on granite surface boulders and where buried approximately 50% of boulders are weathered to grus.
Morphostratigraphic Unit 4
RA characteristics described for unit 4 generally correspond to those deposits previously assigned to the Sacagawea Ridge glaciation in the Wind River Range (CitationRichmond, 1986a; CitationHall, 1999; CitationHall and Jaworowski, 1999). Mapped Sacagawea Ridge deposits are the oldest deposited by ice that was entirely within Sinks Canyon. Sacagawea Ridge deposits are identified only on the north and south walls of the upper canyon above the Missouri geology camp and beyond the canyon mouth ().
Sacagawea Ridge units are identified as separate, indistinct deposits that exhibit only subdued morainal topography. In the upper canyon, Sacagawea Ridge deposits are identified as lateral moraines that are indistinct farther downvalley because they are buried by till of the Bull Lake glaciation and talus. The Sacagawea Ridge unit beyond the canyon mouth also is an indistinct diamicton but consists of stagnant-ice deposits (both till and outwash) that lie outside and above adjacent Bull Lake deposits.
Boulder weathering is more pronounced and pit depths are much deeper on Sacagawea Ridge deposits than on the younger tills. Surface boulder frequency is very low, and most buried granitic boulders are completely disintegrated in these deposits (). All surface boulders, when present, are split and spalled. Weathering pit depth is not a reliable measure of boulder weathering on the older deposits, because weathering rinds tend to weaken the rock between adjacent pits and cause rinds to spall off the boulders.
Morphostratigraphic Units 5 and 6
Deposits corresponding to morphostratigraphic units 5 and 6 are not identified within Sinks Canyon; these deposits are identified only on or near ridge crests beyond the canyon rims. These units are identified only from scattered and isolated diamictons that are gently rounded and have indistinct morainal topography. These deposits would have been deposited by ice that not only filled Sinks Canyon but also flowed above and beyond it.
Morphostratigraphic units 5 and 6 are identified at 6 locations. Only preliminary cosmogenic dates are available that might constrain the ages of these units. Based on the stratigraphic relations to the other glacial deposits in Sinks Canyon and the preliminary 10Be ages discussed below, I tentatively identify these units as Younger Pre–Sacagawea Ridge and Older Pre–Sacagawea Ridge.
Younger Pre–Sacagawea Ridge
A remnant of a lateral moraine (or kame terrace) extends from 2280 to 2310 m (7500 to 7600 ft) along the north canyon rim northeast of the Missouri geology camp.
An extensive remnant of till is located from 2341 to 2250 m (7700 to 7400 ft) on a wide upland area on the south rim of Sinks Canyon between Sinks and Sawmill canyons. Remnants of at least 3 moraine crests trend roughly southwest-northeast from the center of the unit to its eastern and northeastern boundaries. The northwest portion of the unit consists of slope deposits that extend about 120 m (400 ft) down along the inner wall of Sinks Canyon. Near the southwestern extent of the unit, a smaller portion is separated from the main unit by a small stream valley. No obvious areas of outwash were found here. This unit is thought to be the remains of recessional features that formed when the ice body noted in (4) below retreated back across the mesa now occupied by Sawmill Canyon from its terminal position below Table Mountain.
Two remnants of moraines lie on a narrow ridge that separates Sawmill Canyon from Sinks Canyon just above their confluence. These remnants are thought to be associated with ice that remained within the main canyon during the time that ice flowed over the south rim, as noted above (2) and below (4).
Two remnants of moraines are located from 2158 to 1825 m (7100 to 6000 ft) and from 1945 to 1825 m (6400 to 6000 ft) below the southwest end of Table Mountain. These units are thought to be the remains of lateral moraines formed by ice that advanced across the area just south of the present confluence of Sawmill and Sinks canyons during the time that ice occupied the area discussed above in (2).
A remnant of a lateral moraine is mapped from about 2067 to 1976 m (6800 to 6500 ft) west of and immediately above the mouth of Sinks Canyon. The largest remnant lies at the same elevation as the largest moraine remnant below Table Mountain noted above in (4).
Extensive areas that contain scattered and isolated granitic boulders are associated with the Younger Pre–Sacagawea Ridge units discussed in (4) and (5) above (). These scatters of erratic boulders are devoid of surrounding till; the boulders sit directly on bedrock of the Park City Formation (Permian).
Older Pre–Sacagawea Ridge Unit
Two small (25–50 m across) remnants of till overlie the Bighorn Dolomite just northeast of the feature named “George” on the rim of the south canyon wall.
A southwest-northeast-trending remnant of till along the north edge of Table Mountain consists of boulders as large as 4 m in diameter in a coarse, sandy matrix. Except for the intense weathering this till is indistinguishable from younger tills in Sinks Canyon. The unit locally has subdued morainal topography.
The origin of the older Pre–Sacagawea Ridge unit on the north rim of Table Mountain is equivocal. Similar diamictons in the Rocky Mountains that consist of boulders in a poorly sorted, sandy matrix on ridge crests have been interpreted as landslide, alluvial-colluvial, periglacial, or glacial deposits (CitationMadole, 1982). The deposits covering Table Mountain have been interpreted as remnants of a boulder conglomerate facies of the Eocene Wind River Formation (CitationKeefer, 1965; CitationLove et al., 1979; CitationLove and Christiansen, 1985). Although the Older Pre–Sacagawea Ridge diamicton may be nonglacial in origin, I interpret this deposit to be till that was deposited by ice that advanced beyond the walls of Sinks Canyon for the following reasons.
The deposit exhibits subdued morainal topography muted by erosion.
The nearest source of bouldery granitic material is Late Archean granite (CitationLove et al., 1979; CitationFrost et al., 2000), which is exposed in outcrops ≥18 km southwest of Table Mountain near Frye Lake (). Many of the boulders on Table Mountain are granodiorite clasts 1–4 m in diameter that were transported ∼25 km northeastward from the core of the Wind River Range. The size and distance of transport of these boulders suggest transport by glacial ice rather than by fluvial or mass movement processes.
Boulders associated with the Pre–Sacagawea Ridge units commonly are split into several pieces and are deeply pitted (). Most boulders barely protrude above the ground surface due to a combination of granular disintegration and erosion of these boulders. Most boulders that have tops that are level with the present ground surface characteristically have red to orange-yellow Munsell colors (10R 5-6/8 and 7.5YR 5-6/8). Larger (whaleback) boulders commonly are weathered into mushroom-like shapes that are extensively undercut to ∼1 m above ground level. This characteristic emphasizes the importance of the deflation and abrasion of the Pre–Sacagawea Ridge moraine surfaces here. The intensity of boulder weathering and soil truncation indicates that erosion has removed at least 1–2 m and possibly as much as 4 m of material from the Pre–Sacagawea Ridge deposits.
SOIL DEVELOPMENT CHARACTERISTICS
Soil profiles on Pinedale moraines have A-Bw-Cox-C horizons. Pinedale soils have the thinnest sola (), the least amount of illuvial clay, and generally are the least developed in the Sinks Canyon chronosequence (, , ). Colors of B horizons usually have 10YR hues, although 7.5YR hues are locally present where till of the Pinedale glaciation has incorporated Early Wisconsin or Bull Lake materials. Pinedale soils have the lowest values for all measures of clay accumulation and for all indices of profile development (; , ).
All soil profiles on Early Wisconsin moraines have A-Bt-Cox-C horizons (, ). Soils on Early Wisconsin deposits are slightly thicker () and B horizons are redder than those of Pinedale soils. Early Wisconsin and Pinedale soils have similar B horizon textures. Early Wisconsin soils have higher values for all measures of clay accumulation and for all indices of profile development than Pinedale soils (; , ).
Soil profiles described on Bull Lake moraines in Sinks Canyon have A-Bt1-Bt2-Cox-C horizons. Soils on Bull Lake moraines are thicker, have thicker sola, and thicker B-horizons that are redder (7.5YR hues) and have finer textures than soils on Pinedale and Early Wisconsin moraines (, , ; ). Soils on Bull Lake deposits have larger values for all measures of clay accumulation compared to Pinedale soils. Values for Accumulation Indices and pedogenic clay also are greater for Bull Lake soils than Early Wisconsin soils, but clay maxima and PWM values for Bull Lake and Early Wisconsin soils are similar (). All indices of profile development are greater for Bull Lake soils than for the soils in Pinedale and Early Wisconsin deposits (; , ).
Soils developed in Sacagawea Ridge deposits have at least A-Bt1-Bt2-Cox horizons. Soils on these deposits generally are severely eroded and often have relict B horizons that are welded to modern surface horizons (A, AB, BA). Where uneroded, soils are much deeper than those on younger deposits (, ). B horizons are much redder (as red as 5YR hues) and their textures are finer than those of younger soils. Soils in Sacagawea Ridge deposits exhibit multiple stacked Bt horizons that surround large stones that are weathered to grus. The B horizons are clay rich and generally have distinct or prominent clay films. Nearly all of the subsurface boulders below a depth of 70–80 cm are disintegrated and weathered to grus, even those with little or no biotite. I attempted to use hornblende weathering values as a quantitative measure of weathering in this study but found no hornblende in soils on Sacagawea Ridge and Pre–Sacagawea Ridge deposits. The hornblendes, evidently, have been completely weathered from these soils, because hornblende was present in soils on all younger glacial deposits.
Soils in Sacagawea Ridge units commonly are more than 2 m thick, although it is difficult to determine soil depths due to the presence of extensively weathered boulders. At soil site G-2, for example, no solid granitic boulders were present in the profile to a depth of 4.5 m. Such intense weathering in the Sacagawea Ridge soils make it difficult to distinguish illuvial from authigenic clay. Profiles FC-3 and DS-1 show evidence of erosion and subsequent addition of eolian material. Evidence for these processes is indicated by abrupt changes in color, texture, or structure between adjacent upper soil horizons ().
Values for the soil development indices (rubification, texture, and PDI) are much greater for Sacagawea Ridge soils than for soils in the younger deposits (; ). Values for the measures of clay accumulation are more equivocal (). The values for pedogenic clay and the AI are more than twice those of younger soils, while the values of clay maxima and PWM are similar to those for soils on Bull Lake moraines. The similarities in clay maxima and PWM values may be due to the greater erosion described for Sacagawea Ridge soils because of the age of the Sacagawea Ridge deposits and of their positions along the valley walls that make them vulnerable to high rates of erosion. Note that the values for clay maxima and PWM clay are calculated simply as measures of clay percents in the A, B, BC, and CB horizons whereas calculations of the pedogenic clay and AI values compare the quantities of clay in the above soil horizons to that in the C horizons. Thus, values for clay maxima in B horizons and PWM clay decrease due to the loss of soil materials (including clay) when the A and B horizons are eroded over time.
All measures of profile thickness increase dramatically for Pre–Sacagawea Ridge soils in the Sinks Canyon area (). Individual horizons typically are much thicker (e.g., the Cox horizon of TM-1 is ∼6 m), and rubification is more pronounced. B horizons in Pre–Sacagawea Ridge soils always have 5YR hue. Variations in color, structure, texture, and clay film morphology are more obvious between adjacent horizons of Pre–Sacagawea Ridge soils than among Sacagawea Ridge soils, so much so that they resemble disconformities (e.g., Bt1-to-2Bt2 in soil DS-1; Btk-to-Btkb in soil WD-1). The most obvious cause of this phenomenon is erosion followed by aeolian deposition, colluvial accumulation, or soil mixing. Over the estimated >600 ka of their development soils on these deposits may have experienced numerous episodes of erosion caused by periglacial desiccation and deflation processes similar to those proposed by CitationHall (1999) and by CitationApplegarth and Dahms (2001) on moraines at Bull Lake, Dinwoody Lakes, and Torrey Lake. The only measures of clay accumulation that increase from the Sacagawea Ridge to the Pre–Sacagawea Ridge soils are pedogenic clay and the clay AI (); in fact, these measures of clay accumulation are nearly identical in all soils except the younger Pre–Sacagawea Ridge profiles. Similarly, the PDI is the only soil development index to increase continuously over time (); all other indices decrease for the Older Sacagawea Ridge soils. The reasons for the decrease in the rubification index is not clear, but the low texture index may reflect the amount of grus added to the soil via boulder weathering.
Boulder weathering and pedogenic carbonate characteristics vary widely in Pre–Sacagawea Ridge soils. All boulders below 80 cm in profile DS-1 are weathered to grus whereas boulders in profile WD-1 are only slightly weathered. Also, varying amounts of pedogenic carbonate have accumulated in horizons of soil WD-1. Carbonate morphology is discontinuous and ranges from stage 1 to stage 3 (CitationBirkeland, 1999). Wedge-like bodies of materials similar to the overlying horizon are present between fragments of the carbonate-rich horizon (Btkb). This morphology suggests some combination of severe desiccation or periglacial activity may have affected this deposit. The only other soil described in this study that contains pedogenic carbonate is HR-2 (developed in Bull Lake–age material), which exhibits a continuous Stage 1 carbonate morphology. The pedogenic carbonate in these 2 profiles apparently are most likely due to their location at the beyond the canyon mouth, where microclimate conditions are warmer and drier than those within Sinks Canyon.
Comparing RA Characteristics to Those at Other Localities in the Wind River Range
BOULDER WEATHERING
Descriptions of boulder weathering on Pleistocene moraines are limited to a few locales in the Wind River Range. CitationBlackwelder (1915) and CitationRichmond (1986a, Citation1987) described general characteristics of boulders for Pinedale and Bull Lake moraines, but neither of them applied quantitative techniques. Hall's unpublished work (1989) provides the only quantitative measures for clast weathering on Pleistocene moraines in the Wind River Range. Hall's data for clast frequency, surface clast weathering, and weathering pit depth on the Pinedale and Bull Lake moraines at Fremont Lake and Big Sandy Opening show that boulders on Bull Lake moraines are more weathered than those on Pinedale moraines (usually by at least a factor of 2). Hall points out that these results generally agree with the conclusion of CitationBurke and Birkeland (1979) that a change in RA data by a factor of 2 is preferred before assigning deposits to different first-order glaciations rather than as second-order fluctuations within a first-order glaciation.
Although different methods were used to obtain boulder weathering data in Sinks Canyon than those of CitationHall (1989) at Fremont Lake and Big Sandy Opening, the results are similar. In Sinks Canyon, weathering pit depths and the percent of split boulders are the most useful boulder weathering parameters (). These data show that pit depths generally increase by a factor of 2 from Pinedale to Bull Lake moraines, while the percent of split boulders increases by a factor of 6 to 8 (). These weathering characteristics increase by at least a factor of 2 from the Bull Lake to Sacagawea Ridge deposits. Note that the weathering parameters increase by less than 2 from Pinedale to Early Wisconsin deposits and that weathering has progressed on Pre–Sacagawea Ridge boulders to the extent that these parameters are not easily measured due to erosional loss off of the boulders.
Moraine morphology is a useful parameter in Sinks Canyon only for differentiating Pinedale from Bull Lake deposits and Bull Lake from all older deposits. Surface boulder frequencies are not useful in Sinks Canyon because the inner slopes of the moraines are steep, promoting exhumation of subsurface boulders on the moraine slopes.
SOIL DEVELOPMENT
Soil development is the most common form of RA data used to differentiate Pleistocene moraines in the Wind River Range. Variability among soils developed on piedmont moraines in the range is described in several studies (e.g., CitationBirkeland 1973; CitationBurke and Birkeland, 1979; CitationMahaney, 1978; CitationShroba and Birkeland, 1983; CitationSorenson, 1987; CitationHall, 1989; CitationDahms, 1991; CitationHall and Shroba, 1993, Citation1995; CitationHall, 1999).
Local variability among soils on similar-age moraines in Sinks Canyon is attributed mainly to erosion of their slopes as the moraines lie against the steep canyon wall, and to variations in microclimate and vegetation cover along the ∼12-km length of the canyon (). Soils also vary across the canyon according to slope aspect (northwest versus southeast). Loess is ubiquitous here, so that A horizons on all units often contain more fine materials than the underlying B horizons.
Regionally, soil characteristics on similar-age glacial deposits vary widely from the northeast to the southwest flanks of the range. This is chiefly due to differences in till composition between these areas. The lithology of the parent till of the moraines is important in these areas, because it controls the initial content of carbonate and clay in the C horizons.
Piedmont moraines on the northeast flank of the range are dominantly composed of till with a fine-grained (silty) matrix that contains abundant carbonates from the Paleozoic sedimentary rocks that form the walls of the lower canyons through which the valley glaciers flowed (). Thus, the most useful relative-age characteristics of soils on moraines on the northeastern slope moraines is the carbonate contents of B and C horizons (CitationMurphy and Richmond, 1965; CitationRichmond and Murphy, 1965; CitationRichmond, 1986a; CitationShroba, 1989; CitationHall and Shroba, 1993; CitationHall, 1999; CitationApplegarth and Dahms, 2001).
Most piedmont moraines along the southwestern flank of the range generally are composed of quartz-rich tills with a coarse-grained sandy matrix (derived chiefly from sediments derived from granodiorite and granite), which usually contain no detectable primary carbonate. With few primary carbonates in the C horizons, moraine soils on the southwestern flank of the Wind River Range accumulate carbonate only by aeolian influx so that carbonate characteristics are useful only to generally differentiate tills of the Pinedale glaciation from tills of the Bull Lake glaciation (soil development has not been reported from older tills). Thus, previous studies on the southwestern flank of the range report that the most useful soil RA characteristics are loess and solum thickness, a number of B horizon weathering parameters, contents of illuvial clay, and PDI (CitationShroba, 1977; CitationShroba and Birkeland, 1983; CitationSorenson, 1987; CitationHall, 1989; CitationDahms, 1991, Citation1993, Citation1994; CitationHall and Shroba, 1993, Citation1995).
As noted previously, the lithology of most of the Sinks Canyon deposits differs from that previously reported for northeastern flank localities because the tills above the canyon's mouth contain no primary carbonate. Only 3 soils of the Sinks Canyon chronosequence contain carbonates from the local Paleozoic bedrock (): soil profiles HR-2, WD-1, and FC-1. Because of the common lack of carbonates in the tills in and near Sinks Canyon, the most useful RA characteristics of soils here are more similar to those described from the southwestern flank of the range (, ).
SINKS CANYON SOILS VERSUS SOUTHWEST FLANK SOILS
I compared the above clay indices, solum thicknesses, and PDI of Sinks Canyon soils with those reported from the southwestern flank of the range through the use of ratios between the average values of each measurement from both regions. The values from the southwest flank of the range were taken only from published data for soils developed in Pinedale, Early Wisconsin, and Bull Lake tills (; ; CitationHall, 1989, Citation1999; CitationHall and Shroba, 1995). The southwest flank soils data are of 2 kinds: (1) direct measures of soil characteristics (solum thickness, clay maxima in B horizons), and (2) profile weighted means of percent clay (PWM) and whole-profile indices where the values for a single characteristic (AI) or a set of characteristics (PDI) are derived for entire profiles based on the known or inferred values of the parent material.
The above measurements show little difference with time among areas. Soils of all ages vary in similar ways for all measurements except for the clay AI (). Ratios for solum thickness (, ; ) vary around 1.0, indicating soils on similar age deposits have similar thicknesses on both sides of the range. The ratios for PWM and PDI for nearly all soils are <1.0, indicating that soils develop slightly faster on the southwest flank moraines. The only soils in Sinks Canyon with larger PWM and PDI values than those of the southwestern flank of the range are those on Bull Lake moraines. However, Sinks Canyon soils have consistently larger values for clay maxima in B horizons than the southwest flank soils. The differences apparently do not result from higher clay contents of the Sinks Canyon tills. Clay contents of till considered to be of Early Wisconsin age at Fremont Lake varies from 3 to 4% (CitationHall and Shroba, 1995), whereas the Early Wisconsin till in Sinks Canyon contains from 5 to 16% clay. C horizons of the Sinks Canyon Pinedale and Bull Lake soils average 5 and 9.5% clay, whereas C horizons of soils on Pinedale and Bull Lake moraines of the southwestern flank average 4.3 and 12% clay, respectively. Thus, other pedogenic factors such as aeolian influx rates or microclimate variables must be responsible for the higher clay contents in Sinks Canyon soils.
It is curious that while the PWM values for clay are smaller in Sinks Canyon soils (, ; , 10) the clay AI is considerably larger. This difference most likely is related to the procedure by which each parameter is calculated. PWM values relate clay percent as a function of solum thickness while the AI values relate the amount of clay accumulation to the original clay content in the parent material. Thus, the small PWM values of Sinks Canyon soils may reflect higher erosion rates of the soils, whereas the larger AI values suggest that clays accumulate faster in the soils than the soil horizons are thinned by erosion. Such an interpretation is supported by the high clay maxima values in the Sinks Canyon soils noted above and by the ratio of the AI values for the Sinks Canyon and southwest flank soils (). The largest AI ratios for Sinks Canyon are for the soils in Pinedale deposits (>3× the values for the southwest flank), but AI values decrease over time in Sinks Canyon so that AI ratios for soils on Bull Lake deposits in Sinks Canyon essentially match the AI values for soils on the southwest flank Bull Lake moraines. Many Sinks Canyon soils have more clay in A horizons than in the underlying B horizons (). Since no dust influx data exists for the northeast flank that is comparable to the southwest slope data (CitationDahms, 1993; CitationDahms and Rawlins, 1996), the role of dust influx to Sinks Canyon soils cannot yet be estimated. It is possible, however, that the addition of clay through dust flux to soils on younger deposits eventually is nullified by other pedogenic factors (erosion, etc) over time. It also is possible that chemical weathering rates are higher in the southwest slope soils so that more authigenic clays are produced in these soils over time than in soils of the same age in Sinks Canyon. Mean annual precipitation is similar for each area (22 cm yr−1 near the town of Pinedale versus an estimate of 25 cm yr−1 noted earlier near the Missouri geology camp). Because Sinks Canyon is on the leeward slope of the range, adiabatic processes may cause temperature and evapotranspiration to be higher in Sinks Canyon so that chemical weathering rates actually are higher on the southwest flank of the range.
Numeric Age Data
Although none were analyzed specifically for this study, a limited number of cosmogenic radionuclide exposure ages are available for Pinedale, Early Wisconsin, Bull Lake, and older Pre–Sacagawea Ridge deposits in the study area. Sampling, laboratory, accelerator, and analytical procedures for these samples were described previously (CitationFabel et al., in press; CitationDahms et al., 2002).
CitationFabel et al. (in press) report 10Be exposure ages from samples of polished and striated bedrock surfaces along 2 side-valley transects near Popo Agie Falls (). All samples were taken from below the mapped elevations of Pinedale deposits in this portion of the canyon. The samples fall into three main groups. Mean 10Be exposure ages of the youngest samples (nearest the valley floor) of 15.4 ± 1 ka and 17.5 ± 1.0 ka fit well with those reported from Pinedale recessional deposits elsewhere in this region (Gosse et al., 1995b; CitationChadwick et al., 1997; CitationPhillips et al., 1997). Mean exposure ages of the oldest (and highest) samples along these transects are statistically indistinguishable at 82.6 ± 5.2 ka. Finally, a single 10Be exposure age of 67.6 ± 4.2 ka was reported from a sample of polished bedrock at 2440 m, between the lowest (younger) samples and the highest (older) samples along the transect NE of Popo Agie Falls ().
Fabel et al. suggest the progressively older exposure ages represent incomplete abrasion from lower to higher transect elevations by Pinedale ice of bedrock whose cosmogenic signal was last reset by Bull Lake ice. This interpretation was made before lateral moraines with intermediate weathering characteristics were identified between Bull Lake and Pinedale moraines. Note that exposure ages from erratic boulders on Bull Lake deposits in this region fall between >130 ka and 95 ka (CitationChadwick et al., 1997; CitationPhillips et al., 1997), so that abrasion of exposed bedrock in Sinks Canyon by post–Bull Lake ice must account for an exposure age difference of from ∼130 to ∼12 ka. The exposure ages reported by CitationFabel et al. apparently represent the bedrock abrasion accomplished by 2 post–Bull Lake glaciations in Sinks Canyon.
Preliminary 10Be exposure ages of 4 split and weathered granitic boulders on the Older Pre–Sacagawea Ridge deposit () suggest it is a middle Pleistocene deposit. Exposure ages from the boulders of 783 ka (boulder TM-1), 135 ka (TM-2), 351 ka (TM-3), and 180 ka (TM-4) were calculated using a production rate of 5.1 10Be atoms g-1a-1 and an estimated erosion rate of 2.0 mm ka−1 (sample preparation and AMS measurements by PRIME lab, 2002; production rate from CitationStone, 2000; age estimates by J. C. Gosse, personal communication).
The exposure age calculations do not account for the possibility that the boulders may initially have been buried. It is possible that at least 3 m of material may have been eroded from the Sacagawea Ridge deposits (600 to 770 ka; CitationHall and Jaworowski, 1999) inside Sinks Canyon, based on the different thicknesses (5.5 m versus 2.1 m) of the 2 most complete soils described on this map unit (soils G-2 and FC-2, , ). By extrapolation, it is possible that at least 4 to 6 m of material initially buried some of the boulders now found at the surface of the Younger and Older Pre–Sacagawea Ridge deposits. If these boulders initially were buried and subsequently were exhumed, then the age of the deposit could be much older than the above exposure ages. No exposure ages are available for granitic corestones from the unglaciated part of the Wind River Range that we might compare to old glaciated boulders, so it is uncertain what ages such corestones might yield. Nevertheless, the combination of elevation above the adjacent Younger Pre–Sacagawea Ridge deposit, the preliminary exposure ages of the boulders, and the possibility that the exposure ages do not account for burial of the boulders suggests the Table Mountain deposit is older than O-isotope stage 18 (>770 ka).
Conclusions
Relative and numeric age-data indicate that morphostratigraphic units are present in Sinks Canyon that correspond to the 4 Pleistocene glaciations previously identified in the Wind River Range. Deposits above the canyon also suggest that 2 previously unidentified glacial advances occurred here. Sinks Canyon presently is the only locality in the Rocky Mountains where deposits that correspond to Pinedale, Early Wisconsin, Bull Lake, Sacagawea Ridge, and Pre–Sacagawea Ridge glaciations are all reported from one locality.
Pinedale glacial deposits in Sinks Canyon include a complex of 5 terminal-recessional-lateral moraines, all of which consist of till on the canyon floor and along the walls of the upper canyon above and southwest of the Missouri geology camp. Pinedale deposits exhibit characteristics indistinguishable from similar deposits described elsewhere in the range.
Early Wisconsin moraines are mapped here only as lateral moraines between the Pinedale and Bull Lake moraines. Early Wisconsin moraines exhibit characteristics similar to those described previously at Fremont Lake (CitationHall and Shroba, 1995). These characteristics include soil development and boulder weathering characteristics that generally fall between those described for tills of the Pinedale and Bull Lake glaciations.
Bull Lake moraines are the highest glacial deposits near the southwestern edge of the map, where the deposits form 2 moraine crests on the north canyon wall south of Fairfield Creek. Till of the Bull Lake glaciation is covered by younger talus deposits along the northern wall of the lower canyon below (north of) the Missouri geology camp; Bull Lake moraines remain exposed along the south canyon wall to the mouth of the canyon. Bull Lake deposits are mapped on both sides of the Middle Popo Agie River immediately inside and outside the canyon mouth. Deposits of the Bull Lake glaciation are the youngest to have extended beyond the canyon mouth. Bull Lake deposits exhibit more strongly developed soils and boulder weathering than the adjacent Pinedale and Early Wisconsin deposits.
Pre–Bull Lake morphostratigraphic units in Sinks Canyon all are extremely weathered and fragmentary. The Sacagawea Ridge and the Younger Pre–Sacagawea Ridge moraines exhibit extremely subdued topography whereas the Older Pre–Sacagawea Ridge deposits are only remnants of till units. Large surface boulders on all of these deposits are split into several pieces, and soil profiles show evidence of extreme weathering—as suggested by high contents of pedogenic clay, shallow depths to clay-rich B horizons, and complete weathering to grus of boulders below ∼80 cm. Older Pre–Sacagawea Ridge deposits are preserved at only two localities: on the south canyon rim above the Missouri geology camp and on the north rim of Table Mountain. Both localities are ∼150 m above the nearest Younger Pre–Sacagawea Ridge unit.
Evidence of Pinedale, Early Wisconsin, Bull Lake, and Older Pre–Sacagawea Ridge striations here is supported by 10Be exposure ages from erratic boulders and polished and striated bedrock surfaces. Bedrock surfaces exposed behind Pinedale recessional moraines produce mean exposure ages from 15.4 to 17.5 ka. The presence of Early Wisconsin ice here is supported by an exposure age on polished bedrock of 67.6 ± 4.2 that is thought to represent incomplete removal of bedrock by post–Bull Lake ice abrasion. 10Be exposure ages are still being refined from boulders on the Older Pre–Sacagawea Ridge diamicton on Table Mountain. Four preliminary exposure ages of from 135 to 783 ka suggest that if we account for initial burial of the boulders and subsequent erosion of the deposit and of the boulders, the diamicton could have been emplaced during the early-to-middle Pleistocene, or at least prior to O-isotope stage 18 (>770 ka; CitationHall and Jaworowski, 1999; CitationBrown et al., 1994).
Sinks Canyon is one of four localities in the range where Sacagawea Ridge deposits are identified (the others are Dinwoody Lakes, Fremont Lake, and Bull Lake). If no deposits are older than 660–770 ka at Richmond's Cedar Ridge section (CitationRichmond, 1964; CitationHall and Jaworowski, 1999) and the Younger and Older Pre–Sacagawea Ridge deposits in Sinks Canyon represent separate glaciations, then Sinks Canyon contains the most complete record of glaciation to date in the Wind River Range and has the only surficial record of Pleistocene glaciation prior to O-isotope stage 18 in the Rocky Mountains.
FIGURE 1. Map of the Wind River Range showing the location of Sinks Canyon on the northeastern flank of the range. The locations of other localities important to the Rocky Mountain glacial succession are provided for reference
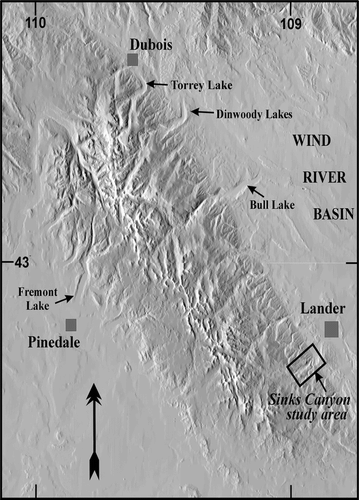
FIGURE 2. Map of Pleistocene glacial deposits in and near Sinks Canyon. Elevations of contour lines are in feet and are generalized from those on the Cony Mountain, Fossil Hill, Mt. Arter SE, Lander, and Wolf Point U.S. Geological Survey 7.5-ft topographic maps (Wyoming)
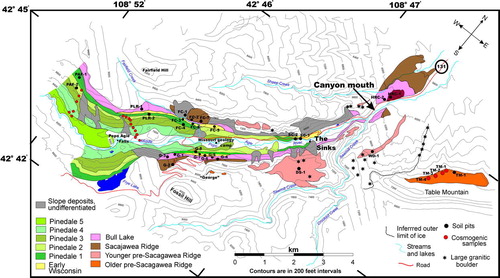
FIGURE 3. (a) View toward the southwest from the north rim of Sinks Canyon. A prominent bedrock step is at right center. The trees on the south canyon wall obscure the morphology of the Pinedale, Early Wisconsin, Bull Lake, and Sacagawea Ridge moraines. (b) View of the Pinedale, Early Wisconsin, Bull Lake, and Sacagawea Ridge moraines mapped in the southwest portion of the canyon as seen from the point labeled “George” in . (c) View from “George” toward the northeast to the mouth of the canyon. Lander is near top center. Large grassy area above the valley at upper right is part the Younger Pre–Sacagawea Ridge morphostratigraphic unit. (d) View from the north rim of Table Mountain toward the southwest showing the mouth of Sinks Canyon and two Younger Pre–Sacagawea Ridge moraine remnants mapped beyond the mouth of the canyon
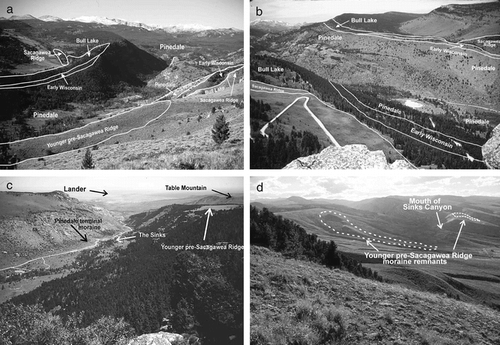
FIGURE 4. Representative morphology of granite boulders on Pleistocene deposits in Sinks Canyon. (a) Boulders on Bull Lake moraine north of PLR-2 (). Backpack indicated for scale. (b) Split and weathered boulder on the Younger Pre–Sacagawea Ridge map unit shown on the south rim of Sinks Canyon in . (c) Scatter of erratic granite boulders between Table Mountain and the Younger Pre–Sacagawea Ridge moraine remnant southwest of Table Mountain shown in . View is from the highest (southwest) end of the moraine remnant. All of the boulders that are clearly visible in the distance (such as the one indicated) are >3 m in diameter and lie directly on the dipslope of the Permian Park City Formation; they are thought to have had all surrounding till eroded from around them. (d) View of two of the granite boulders on the Older Pre–Sacagawea Ridge deposit mapped on Table Mountain () from which preliminary 10Be exposure ages were obtained. Hat and backpack for scale. These boulders yielded exposure ages of 783 kyr (TM-1) and 135 kyr (TM-2). View is from Table Mountain looking north toward Lander
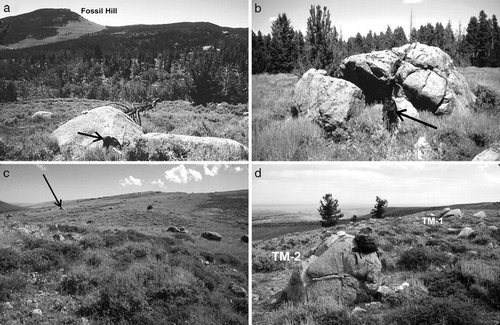
FIGURE 5. Solum thickness (A + B horizons) and depths to Cox-C horizon boundaries of soils plotted against estimated age of the glacial parent material in Sinks Canyon. Early-to-mid-Pleistocene age estimates are used for the Pre–Sacagawea Ridge deposits
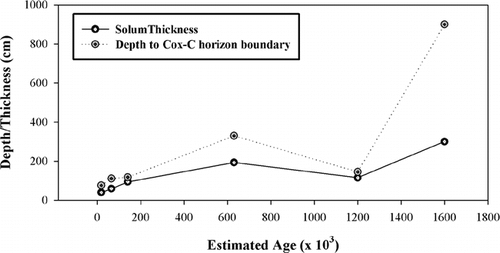
FIGURE 6. Plot of the various methods used to estimate clay accumulation in soils described in Sinks Canyon. Clay data is plotted against age of the parent material using early-to-mid-Pleistocene ages for the Pre–Sacagawea Ridge deposits
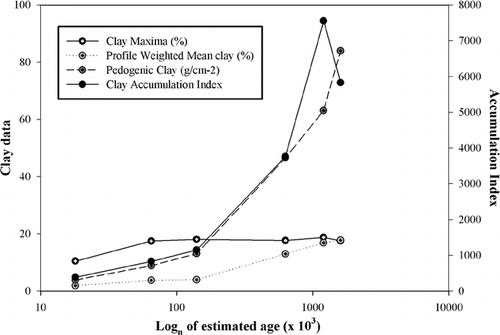
FIGURE 7. Plot of soil development indices that are most representative of the relative ages of the glacial deposits in Sinks Canyon. Index values are plotted against ages of the deposits using early-to-mid Pleistocene ages for the Pre–Sacagawea Ridge deposits
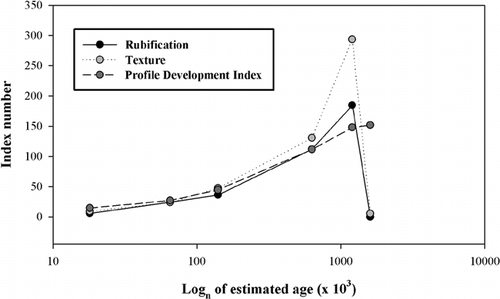
FIGURE 8. Plot of clay accumulation data for soils developed on moraines of the southwestern slope of the Wind River Range vs. ages of moraines. Note that soils on Pinedale and Early Wisconsin deposits exhibit similar values, whereas soil on Bull Lake deposits have values from ∼2–3× those of soils on the younger deposits. This phenomenon may represent the processes noted by CitationHall (1999) whereby the soil sola on older glacial deposits undergo periglacial erosion during subsequent full glacial conditions. Data taken from CitationHall (1989), CitationHall and Shroba (1993, Citation1995), and summarized in
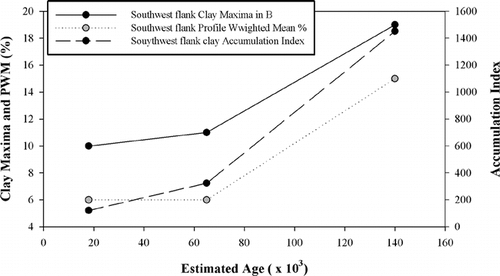
FIGURE 9. Ratios of clay accumulation and soil development index values for soils on Sinks Canyon moraines versus values for soils on moraines of the southwest slope of the Wind River Range (). Data are plotted against moraine ages. Ratios compare soil development characteristics for the two areas. Note that Sinks Canyon values are within ±0.5 of the western slope values for most characteristics when profile depth is used to weight the values. Ratios that plot outside the above range (PWM and AI) are for characteristics that are not weighted for profile depth. Early-to-mid-Pleistocene age estimates are for the parent materials of the Pre–Sacagawea Ridge soils
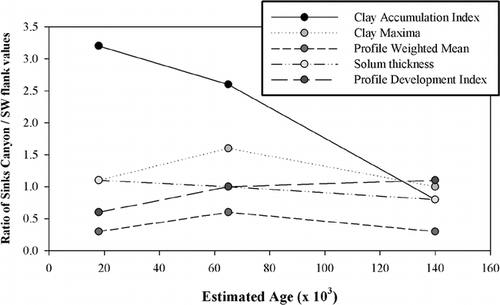
Table 1 Moraine morphology and weathering data for glacial deposits in and near Sinks Canyon, Wyoming
Table 2 Morphologic characteristics for soils in Sinks Canyon, Wyoming
Table 2 (Cont)
Table 2 (Cont)
Table 3 Laboratory data for soils in and near Sinks Canyon, Wyoming
Table 3 (Cont)
Table 3 (Cont)
Table 4 Relative development characteristics for Sinks Canyon soils
Table 5 Soil Relative Age data compiled from localities on the SW flank of the Wind River Rangea
Acknowledgments
This project was partially funded in 1994, 1996, and 1998 by Summer Fellowships from the Graduate College of the University of Northern Iowa. Cosmogenic exposure ages from Table Mountain were provided through a seed grant from PRIME Lab, Purdue University. Bob Bauer at the University of Missouri Edwin R. Branson field camp graciously provided field support for the duration of this research. Allyson Anderson provided field assistance in 1996, and Mike Applegarth performed the soil bulk density analyses. I thank Darrel Trembly and Rick Wise for their permission to work in Sinks Canyon State Park. I also thank several private landowners, including Jack and Alice Nicholas, the Dave Raynolds family, the Bob Thomsen family, the Walker family, and David Conrad as well as Central Wyoming College and the Wyoming Nature Conservancy for access to areas outside the canyon. The U.S. Forest Service allowed sampling in the Shoshone National Forest through Special Use Permit #2037-01. Derek Fabel constructed the contours on Figure 2. Ralph Shroba and Rich Madole provided constructive suggestions that substantially improved the manuscript.
References Cited
- Applegarth, M. and D. E. Dahms . 2001. Soil catenas of calcareous tills, Whiskey Basin, Wyoming, USA. Catena 42:17–38.
- Birkeland, P. W. 1973. Use of relative age-dating methods in a stratigraphic study of rock glacier deposits, Mt. Sopris, Colorado. Arctic and Alpine Research 5:401–416.
- Birkeland, P. W. 1999. Soils and Geomorphology. New York: Oxford University Press. 430 pp.
- Birkeland, P. W. , M. Machette , and K. Haller . 1991. Soils as tool for applied Quaternary geology. Miscellaneous Publication 91–3, Utah Geological and Mineral Survey. 63 pp.
- Blackwelder, E. 1915. Cenozoic history of the mountains of central Wyoming. Journal of Geology 23:97–117. 193–217, 307–340.
- Brown, L. , J. Pickens , and B. Singer . 1994. Matuyama-Brunhes transition recorded in lava flows of the Chilean Andes. Geology 22:299–302.
- Burke, R. M. and P. W. Birkeland . 1979. Reevaluation of Multiparameter Relative Dating Techniques and Their Application to the Glacial Sequence along the Eastern Escarpment of the Sierra Nevada, California. Quaternary Research 11:21–51.
- Chadwick, O. A. , R. D. Hall , and F. M. Phillips . 1997. Chronology of Pleistocene glacial advances in the central Rocky Mountains. Geological Society of America Bulletin 109:1443–1452.
- Colman, S. M. and K. L. Pierce . 1986. Glacial sequence near McCall, Idaho: weathering rinds, soil development, morphology, and other relative age criteria. Quaternary Research 25:25–42.
- Dahms, D. E. 1991. Eolian sedimentation and soil development on moraine catenas of the Wind River Mountains, west-central Wyoming. Ph.D. dissertation, Dept. of Geography, University of Kansas, Lawrence, KS, U.S.A. 340 pp.
- Dahms, D. E. 1993. Mineralogical evidence for eolian sediments in soils on late Quaternary moraines, Wind River Mountains, Wyoming. Geoderma 59:175–196.
- Dahms, D. E. 1994. Mid-Holocene erosion of soil catenas on moraines near the Type Pinedale Till, Wind River Range, Wyoming. Quaternary Research 42:41–48.
- Dahms, D. E. 2002. Glacial stratigraphy of Stough Creek basin, Wind River Range, Wyoming. Geomorphology 42:59–83.
- Dahms, D. E. 2004. Glacial Limits in the Middle and Southern Rocky Mountains, U.S.A., south of the Yellowstone Ice Cap. In Ehers, J., and Gibbard, P. L., (eds.), Quaternary Glaciations—Extent and Chronology: Part II North America. Vol. 2 of Developments in Quaternary Science. Amsterdam: Elsevier, 269–282. Map included on CD-ROM (scale 1:1,000,000).
- Dahms, D. E. and C. L. Rawlins . 1996. A two-year record of eolian sedimentation in the Wind River Range, Wyoming, U.S.A. Arctic and Alpine Research 28:210–216.
- Dahms, D. E. , J. C. Gosse , and D. Elmore . 2002. Preliminary 10Be evidence for Pre–Sacagawea Ridge glaciation(s) from Sinks Canyon, Wind River Range, Wyoming. Abstracts with Program, GSA Annual Meeting, Denver 34:6 131.
- Fabel, D. , J. Harbor , D. Dahms , A. James , D. Elmore , K. Daley , and C. Steele . in press. Spatial patterns of glacial erosion at a valley scale derived using cosmogenic radionuclide concentrations in rock. Annals of the Association of American Geographers. .
- Frost, B. R. , C. D. Frost , T. P. Hulsebosch , and S. M. Swapp . 2000. Origin of the charnockites of the Louis Lake batholith, Wind River Range, Wyoming. Journal of Petrology 41:1759–1776.
- Gosse, J. C. , E. D. Evenson , J. Klein , B. Lawn , and R. Middleton . 1995a. Precise cosmogenic 10Be measurements in western North America: support for a global Younger Dryas cooling event. Geology 23:877–80.
- Gosse, J. C. , J. Klein , E. B. Evenson , B. Lawn , and R. Middleton . 1995b. Beryllium-10 dating of the duration and retreat of the last Pinedale glacial sequence. Science 268:1329–1333.
- Hall, R. D. 1983. The influence of till petrology on weathering of moraines in southwestern Montana and northwestern Wyomng. In Evenson, E. B. (ed.), Tills and Related Deposits. Proceedings, INQUA symposia, U.S.A. 1981, Argentina 1982. Balkema: 127–139.
- Hall, R. D. 1989. Contribution to 1989 Friends of the Pleistocene Trip, Wind River Mountains. Department of Geology, Indiana-Purdue University at Indianapolis. 50 pp.
- Hall, R. D. 1999. Effects of climate change on soils in glacial deposits, Wind River Basin, Wyoming. Quaternary Research 51:248–261.
- Hall, R. D. and C. Jaworowski . 1999. Reinterpretation of the Cedar Ridge Section, Wind River Range, Wyoming: implications for the glacial chronology of the Rocky Mountains. Geological Society of America Bulletin 111:1233–1249.
- Hall, R. D. and R. R. Shroba . 1993. Soils developed in the glacial deposits of the type areas of the Pinedale and Bull Lake glaciations, Wind River Range, Wyoming, U.S.A. Arctic and Alpine Research 25:368–373.
- Hall, R. D. and R. R. Shroba . 1995. Soil evidence for a glaciation intermediate between the Bull Lake and Pinedale glaciations at Fremont Lake, Wind River Range, Wyoming, USA. Arctic and Alpine Research 27:89–98.
- Harden, J. W. 1982. A quantitative index of soil development from field descriptions: examples from a chronosequence in central California. Geoderma 28:1–28.
- Harden, J. W. , E. M. Taylor , C. Hill , R. K. Mark , L. D. McFadden , M. C. Reheis , J. M. Sowers , and S. G. Wells . 1991. Rates of soil development from four soil chronosequences in the southern Great Basin. Quaternary Research 35:383–399.
- Jackson, M. L. 1969. Soil Chemical Analysis: Advanced Course. Department of Soil Science, University of Wisconsin, Madison.
- Keefer, W. R. 1965. Stratigraphy and geologic history of the uppermost Cretaceous, Paleocene, and Lower Eocene rocks in the Wind River Basin, Wyoming. U.S. Geological Survey Professional Paper 495-A. 77 pp.
- Klute, A. (ed.),. 1986. Methods of Soil Analysis: Part 1, Physical and Mineralogical Methods, 2nd ed. Madison, WI: American Society of Agronomy.
- Love, J. D. and A. C. Christiansen . 1985. Geologic map of Wyoming. U. S. Geological Survey Map scale 1: 500,000.
- Love, J. D. , A. C. Christiansen , and R. W. Jones . 1979. Preliminary geologic map of the Lander 1 degrees by 2 degrees quadrangle, central Wyoming. U.S. Geological Survey Open-File Report OF-79-1301. Map scale 1:250,000.
- Love, J. D. , A. C. Christiansen , and A. J. Ver Ploeg . 1992. Stratigraphic nomenclature chart for Wyoming. Geological Survey of Wyoming Open-File Report 92–2. 1 sheet.
- Madole, R. F. 1982. Possible origins of till-like deposits near the summit of the Front Range in north-central Colorado. U. S. Geological Survey Professional Paper 1243. 31 pp.
- Mahaney, W. C. 1978. Late Quaternary stratigraphy and soils in the Wind River Mountains, western Wyoming. In Mahaney, W. C. (ed.), Quaternary Soils. Geological Abstracts, Norwich, England, 223–264.
- McCalpin, J. and M. Berry . 1996. Soil catenas to estimate ages of movements on normal fault scarps, with an example from the Wasatch fault zone, Utah, U.S.A. Catena 27:265–286.
- McFadden, L. 1988. Climatic influences on rates and processes of soil development in Quaternary deposits of southern California. In Reinhardt, J., and Sigleo, W. R. (eds.), Paleosols and Weathering through Geologic Time: Principles and Applications. . Geological Society of America Special Paper 206:153–177.
- McFadden, L. and R. J. Weldon . 1987. Rates and processes of soil development in Quaternary terraces in Cajon Pass, southern California. Geological Society of America Bulletin 98:280–293.
- Muhs, D. 1983. Airborne dust fall on the California Channel Islands, U.S.A. Journal of Arid Environments 6:223–238.
- Murphy, J. F. and G. M. Richmond . 1965. Geologic Map of the Bull Lake West quadrangle, Fremont County, Wyoming. U.S. Geological Survey Geological Quadrangle Map GQ-432. Map scale 1: 24,000.
- National Soil Survey Committee of Canada, 1974. The System of Soil Classification for Canada. Canada Department of Agriculture Publication 1455.
- Phillips, F. M. , M. G. Zreda , J. C. Gosse , J. Klein , E. B. Evenson , R. D. Hall , O. A. Chadwick , and P. Sharma . 1997. Cosmogenic 36Cl and 10Be ages of Quaternary glacial and fluvial deposits of the Wind River Range, Wyoming. Geological Society of America Bulletin 109:1453–1463.
- Reheis, M. C. and R. Kihl . 1995. Dust deposition in southern Nevada and California, 1984–1989: relations to climate, source area, and source lithology. Journal of Geophysical Research 100:8893–8918.
- Reheis, M. , J. C. Goodmacher , J. W. Harden , L. D. McFadden , T. K. Rockwell , R. R. Shroba , J. M. Sowers , and E. M. Taylor . 1995. Quaternary soils and dust deposition in southern Nevada and California. Geological Society of America Bulletin 107:1003–1022.
- Richmond, G. M. 1964. Three pre–Bull Lake tills in the Wind River Mountains, Wyoming: A reinterpretation. U.S. Geological Survey Professional Paper 501-D: D109–D140.
- Richmond, G. M. 1965. Glaciation of the Rocky Mountains. In Wright, H. E., Jr., and Frey, D. G. (eds.), The Quaternary of the United States. Princeton, NJ: Princeton University Press, 217–230.
- Richmond, G. M. 1973. Geologic map of the Fremont Lake south quadrangle, Sublette County, Wyoming. U.S. Geological survey, Geologic Quadrangle Map GQ-1138.
- Richmond, G. M. 1976. Pleistocene stratigraphy and chronology in the Rocky Mountains of western Wyoming. In Mahaney, W. C. (ed.), Quaternary Stratigraphy of North America. Stroudsburg, PA: Dowden, Hutchinson, and Ross, 353–379.
- Richmond, G. M. 1983. Modification of glacial sequence along Big Sandy River, southern Wind River Range, Wyoming. Geological Society of America Abstracts 15:5 431.
- Richmond, G. M. 1986a. Stratigraphy and correlation of glacial deposits of the Rocky Mountains, the Colorado Plateau and the ranges of the Great Basin. In Sibrava, V., Bowen, D. Q., and Richmond, G. M. (eds.), Quaternary Glaciations in the Northern Hemisphere. Oxford: Pergamon Press, 106–108.
- Richmond, G. M. 1986b. Stratigraphy and chronology of glaciations in Yellowstone National Park. In Sibrava, V., Bowen, D. Q., and Richmond, G. M. (eds.), Quaternary Glaciation of the Northern Hemisphere. Oxford: Pergamon Press, 83–98.
- Richmond, G. M. 1987. Type Pinedale Till in the Fremont Lake area, Wind River Range, Wyoming. Geological Society of America, Centennial Field Guide—Rocky Mountain Section, 201–204.
- Richmond, G. M. and J. F. Murphy . 1965. Geologic map of the Bull Lake East quadrangle, Fremont County, Wyoming. U.S. Geological Survey Geological Quadrangle Map GQ-431. Map scale 1: 24,000.
- Richmond, G. M. and J. F. Murphy . 1989. Preliminary Quaternary geologic map of the Dinwoody Lake area, Fremont County, Wyoming. U.S. Geological Survey Open-File Report 89:435–441.
- Shroba, R. R. 1977. Soil development in Quaternary tills, rock glacier deposits, and taluses, southern and central Rocky Mountains. Ph.D. dissertation, University of Colorado, Boulder, CO. 424 pp.
- Shroba, R. R. 1989. Physical properties and laboratory data for soils formed in Pleistocene tills at Bull Lake, Dinwoody Lakes, and Fremont Lakes, Fremont and Sublette counties, Wyoming. U.S. Geological Survey Open-File Report OFR-89-370. 14 pp.
- Shroba, R. R. and P. W. Birkeland . 1983. Trends in Late Quaternary soil development in the Rocky Mountains and Sierra Nevada of the western United States. In Wright, H. E., Jr. (ed.), Late Quaternary Environments of the United States, Volume I: The Late Pleistocene. Minneapolis: University of Minnesota Press, 145–156.
- Singer, M. J. and P. Janitzky . 1986. Field and laboratory procedures used in a soil chronosequence study. U.S. Geological Survey Bulletin, 1648.
- Soil Conservation Service, 1972. Soil survey laboratory methods and procedures for collecting soil samples. U.S. Department of Agriculture, Soil Survey Investigative Report No. 1.
- Soil Survey Staff, 1994. Soil Survey Manual. U.S. Department of Agriculture Handbook No. 18.
- Soil Survey Staff, 1999. Soil Taxonomy, 2nd ed. U.S. Department of Agriculture, NRCS, Agriculture Handbook No. 436.
- Sorenson, C. J. 1987. Soils Map of the Fremont Lake South Quadrangle, Sublette County, Wyoming. USGS Miscellaneous Investigations Map I-1800.
- Stone, J. 2000. Air pressure and cosmogenic isotope production. Journal of Geophysical Research 105-B10:23753–23760.
- Taylor, E. M. 1986. Impact of time and climate on Quaternary soils in the Yucca Mountain area of the Nevada test site. MS thesis, University of Colorado–Boulder.